- Research article
- Open access
- Published: 13 November 2023

A quantitative evaluation of the impact of vaccine roll-out rate and coverage on reducing deaths: insights from the first 2 years of COVID-19 epidemic in Iran
- Mahan Ghafari ORCID: orcid.org/0000-0002-4123-3287 1 , 2 ,
- Sepanta Hosseinpour 3 ,
- Mohammad Saeid Rezaee-Zavareh 4 ,
- Stefan Dascalu 2 ,
- Somayeh Rostamian ORCID: orcid.org/0000-0002-7584-9493 5 ,
- Kiarash Aramesh 6 ,
- Kaveh Madani 7 &
- Shahram Kordasti ORCID: orcid.org/0000-0002-0347-4207 8
BMC Medicine volume 21 , Article number: 429 ( 2023 ) Cite this article
2433 Accesses
35 Altmetric
Metrics details
Vaccination has played a pivotal role in reducing the burden of COVID-19. Despite numerous studies highlighting its benefits in reducing the risk of severe disease and death, we still lack a quantitative understanding of how varying vaccination roll-out rates influence COVID-19 mortality.
We developed a framework for estimating the number of avertable COVID-19 deaths (ACDs) by vaccination in Iran. To achieve this, we compared Iran’s vaccination roll-out rates with those of eight model countries that predominantly used inactivated virus vaccines. We calculated net differences in the number of fully vaccinated individuals under counterfactual scenarios where Iran’s per-capita roll-out rate was replaced with that of the model countries. This, in turn, enabled us to determine age specific ACDs for the Iranian population under counterfactual scenarios where number of COVID-19 deaths are estimated using all-cause mortality data. These estimates covered the period from the start of 2020 to 20 April 2022.
We found that while Iran would have had an approximately similar number of fully vaccinated individuals under counterfactual roll-out rates based on Bangladesh, Nepal, Sri Lanka, and Turkey (~ 65–70%), adopting Turkey’s roll-out rates could have averted 50,000 (95% confidence interval: 38,100–53,500) additional deaths, while following Bangladesh’s rates may have resulted in 52,800 (17,400–189,500) more fatalities in Iran. Surprisingly, mimicking Argentina’s slower roll-out led to only 12,600 (10,400–13,300) fewer deaths, despite a higher counterfactual percentage of fully vaccinated individuals (~ 79%). Emulating Montenegro or Bolivia, with faster per capita roll-out rates and approximately 50% counterfactual full vaccination, could have prevented more deaths in older age groups, especially during the early waves. Finally, replicating Bahrain’s model as an upper-bound benchmark, Iran could have averted 75,300 (56,000–83,000) deaths, primarily in the > 50 age groups.
Conclusions
Our analysis revealed that faster roll-outs were consistently associated with higher numbers of averted deaths, even in scenarios with lower overall coverage. This study offers valuable insights into future decision-making regarding infectious disease epidemic management through vaccination strategies. It accomplishes this by comparing various countries’ relative performance in terms of timing, pace, and vaccination coverage, ultimately contributing to the prevention of COVID-19-related deaths.
Peer Review reports
On 4 May 2023, the World Health Organization (WHO) determined that the public health emergency phase of the COVID-19 pandemic was over and laid out recommendations for countries on their transition into the long-term management of COVID-19, among other infectious diseases [ 1 ]. While the announcement that the healthcare emergency was declared over may have brought some relief to the public, it did not signify an end to the pandemic. As such, governments still need to stay vigilant and support the global effort to take necessary actions for suppressing COVID-19 transmission and reducing the burden of the disease. Among the various public health measures that were implemented throughout the pandemic, maximising the impact of vaccination worldwide has been a key factor in bringing the public health emergency to an end [ 2 ]. In this respect, lessons derived from national COVID-19 vaccination campaigns worldwide are crucial as they demonstrate the successes and shortcomings of different countries in reducing the burden of disease during a public health emergency.
Since early 2021, vaccination has been a major contributor to reducing the burden of COVID-19 globally [ 3 , 4 ]. However, its benefits were not equitably distributed to every country partly due to challenges with the production, distribution, and affordability of vaccines [ 5 , 6 ] despite efforts to fairly allocate them globally [ 7 ] and partly due to vaccine hesitancy [ 8 ], which remains a threat to global health. While many studies have focused on quantifying the effectiveness of vaccination campaigns by estimating the number of prevented deaths as a result of vaccination [ 3 , 9 , 10 , 11 ], fewer studies focused on quantifying the impact of national vaccination programmes’ speed and timings on reducing the burden of COVID-19 [ 12 , 13 , 14 , 15 ]. These studies mainly investigated the economic and epidemiological impacts of vaccine roll-out timing and speed on disease burden under hypothetical scenarios of fixed or time-varying roll-out rates. However, very limited attention has been given to quantitatively comparing countries’ relative performance based on their vaccine roll-out rates on reducing the burden of COVID-19. Such comparisons would allow for the identification of countries with best practices in implementing effective vaccination programmes, highlight global disparities in vaccination roll-outs, provide a basis for evaluating the impact of specific policies and strategies related to vaccination roll-out speed and timing, and promote a more data-driven decision-making in public health.
By late April 2022, Iran had achieved a vaccination coverage of nearly three quarters of its eligible population against COVID-19, which marked a relatively high level compared to many other upper-middle-income countries (UMICs) and lower-middle-income countries (LMICs) [ 16 ]. During this period, approximately 80% of administered doses consisted of the BBIBP-CorV inactivated virus vaccine, while about 9% were home-grown vaccines, primarily the BIV1-COvIran inactivated virus vaccine (Additional file 1 : Tables S1 and S2). The remaining doses were mainly composed of AZD1222 and Sputnik V viral vector vaccines. However, despite the high overall vaccination coverage, Iran’s performance fell short when compared to nearly all other LMICs and UMICs with similar vaccination coverage. Having reported nearly 130,000 confirmed deaths and an excess death mortality twice as high by December 2021 [ 17 ], Iran ranked 29th out of all 44 UMICs in terms of the number of averted deaths by vaccination per person (Additional file 1 : Fig. S1) [ 3 ].
While several countries initiated the process of purchasing vaccines towards the end of 2020 and early 2021 through local production, bilateral advance purchase agreements, and the COVID-19 Vaccine Global Access Facility (COVAX) [ 18 , 19 ], Iran faced several challenges with securing an adequate and timely supply of vaccines. This was partly due to limited global vaccine production capacity and high demand and in part due to geopolitical factors that were unique to Iran [ 20 ]. A prime example of geopolitical tensions was the decision to ban the importation of vaccines from the USA and UK to Iran [ 21 ]. The country was also not included in the first interim distribution forecast list from COVAX and received its first batch on 5 April 2021 [ 22 ] while several other countries received them nearly a month earlier [ 23 , 24 ]. These factors, along with several others such as delayed home-grown production of vaccines and importations from other countries, contributed to the postponed commencement of the vaccination campaign in Iran. Consequently, 6 months after the start of their vaccination, less than 5% of the Iranian population had been fully vaccinated [ 20 ].
A range of public health interventions such as lockdowns, social distancing, contact tracing, and mask wearing in public spaces played a crucial role in mitigating the burden of COVID-19 with varying degrees of effectiveness [ 25 ]. As the mass production of vaccines ramped up in 2021, countries began to plan the relaxation of restrictions in tandem with the accelerated pace of vaccination roll-out. During this period, the pivotal role of effective vaccination strategies in reducing the burden of disease became increasingly important. This context underscores the importance of evaluating Iran’s vaccination campaign, as it provides a unique opportunity to understand the impact of delayed vaccination on reducing the number of vaccine-preventable deaths. It can also shed light on the factors that contributed to the increased burden of COVID-19 relative to countries with faster vaccination roll-out and identify potential problems and best practices in vaccination strategies that can be applied more broadly to other countries in the future.
In this work, we developed a framework to retroactively calculate the age-stratified number of avertable deaths in Iran had the country followed the same per capita roll-out rates as other countries. By selecting eight model countries that predominantly vaccinated their populations with inactivated virus vaccines, we examined the impact of vaccination programme start dates, roll-out rates, and overall coverage on avertable COVID-19 deaths (ACDs) in Iran. Our main focus was to estimate the number of avertable deaths by vaccination and not other public health interventions such as lockdowns which have also been shown to play a major role in shaping the burden of disease in Iran [ 26 ]. In the end, we discussed a few key public health decisions that may have contributed to the delayed start of vaccination in Iran.
We collected all-cause mortality data from Iran’s National Organisation for Civil Registration ( https://www.sabteahval.ir/en ) and the number of fully vaccinated individuals from Our World in Data [ 16 ] and the WHO dashboard [ 27 ]. We also obtained the economic status of each country from the World Bank income group in 2020 [ 28 ], a year prior to the start of vaccination campaigns in most countries. We selected candidate model countries with comparable income levels and predominant vaccine types to Iran, particularly those that mainly used inactivated virus vaccines such as the BBIBP-CorV vaccine by Sinopharm, as documented in the UNICEF COVID-19 Vaccine Market Dashboard [ 3 , 29 ]. Since Iran was classed as UMIC before the commencement of vaccine distribution up to 2020 and LMIC from 2021 onwards, we included candidate model countries from both LMICs and UMICs with comparable income levels [ 28 , 30 , 31 ]. These countries include Argentina (UMIC), Bangladesh (LMIC), Bolivia (LMIC), Montenegro (UMIC), Nepal (LMIC), Sri Lanka (LMIC), and Turkey (UMIC). We also included Bahrain, a high-income country (HIC), as an upper-bound benchmark for vaccine coverage and pace relative to Iran.
We assumed that the vaccination roll-out in selected countries would follow the same pattern where the older age groups and those at risk would be vaccinated first in a descending age order [ 32 ]. Since the information on the roll-out start dates for Iran is only available for the first dose in each age group, we assumed that the time difference in receiving the full dose (two doses for most vaccines, one or three for a few other manufacturers) between each consecutive age group is the same as the time difference for the primary dose (see Additional file 1 : Table S3).
To calculate the net difference in the number of fully vaccinated individuals between Iran and modelled countries over time, we first took the per capita daily number of fully vaccinated individuals in model country M , r M ( t ) = n M ( t ) /P M , where n ( t ) is the daily number of fully vaccinated people and P is the total population size of the country. We then re-normalised the per capita number of fully vaccinated individuals in country M with respect to Iran’s population size such that the counterfactual number of fully vaccinated Iranians based on model country M would be, ñ Iran ( t ) = r M ( t ) P Iran . The net difference in the daily number of fully vaccinated Iranians in age group i , Δ n ( i ) ( t ), based on the counterfactual vaccine roll-out rate of model country M then becomes:
where ᾶ ( i ) ( t ) and α ( i ) ( t ) represent the fraction of newly vaccinated individuals under counterfactual and factual scenarios, respectively, and n ( i ) Iran is the number of fully vaccinated individuals in age group i in Iran at time t . The allocation of newly vaccinated individuals follows a descending age order, starting with the oldest age group receiving the vaccines first. The time for subsequent younger age groups to begin vaccination is determined based on the number of days elapsed since the older age group received the primary dose (Additional file 1 : Table S3).
The number of avertable deaths as measured from changes in excess mortality in age group i on day t as a result of net difference in vaccine roll-out rate under the counterfactual scenario based on model country M becomes:
where p ( i ) is the vaccine effectiveness against death in age group i ∈ {5–9,10–14, …,75–79, 80 +} years old. In other words, if there is no net difference in the number of fully vaccinated individuals on day t in age group i , there will not be any averted deaths due to vaccination 2 weeks later (i.e. D ( i ) ( t + 14 ) = 0), and the number of daily deaths at time t + 14 will remain the same as the estimated excess deaths in that age group. We assumed that fully vaccinated individuals became protected against deaths from COVID-19 2 weeks after receiving the full dose and that the effectiveness of the vaccine against death is identical across all inactivated virus vaccines and remains the same over time such that p ( i ) = 0.923 (95% confidence interval: 0.672–0.982) for i > 60 years age groups and p ( i ) = 0.801 (95% confidence interval: 0.611–0.898) for i ≤ 60 years age groups [ 33 ].
We used a previously published model for calculating the number of COVID-19 deaths in Iran using excess mortality data across all age groups eligible to receive vaccination [ 32 ]. Age-stratified excess mortality also enabled us to take into account the age-dependent profile of COVID-19 infection fatality ratio (see Figure. 2 in ref [ 32 ]). Our analysis covered the period from the start of the pandemic up to 20 April 2022 as the association between excess mortality and reported COVID-19 deaths weakened after the end of the Omicron BA.1/2 wave (see Additional file 1 : Fig. S2).
Our analysis revealed that by 20 April 2022, Iran had 292,666 (95% confidence interval: 262,414–322,919) deaths associated with COVID-19 based on excess mortality estimates and that 67.5% of the population were fully vaccinated. To further investigate the extent to which Iran’s performance could have been enhanced or diminished if alternative vaccination strategies were employed, we examined counterfactual scenarios whereby Iran’s vaccine roll-out rate was replaced with that of the model countries to see if Iran would have had more (or fewer) ACDs.
Our findings showed that for a fixed overall percentage of fully vaccinated individuals, faster roll-out rates were associated with higher ACDs. While the percentage of fully vaccinated Iranians based on a counterfactual vaccine roll-out rate from Bangladesh, Nepal, Sri Lanka, and Turkey would have roughly been the same (~ 65–70%), Iran could have averted as many as 50,000 (95% confidence interval: 38,100–53,500) deaths if it had followed Turkey’s per capita roll-out rates and would have had as many as 52,800 (17,400-189, 500) more deaths if it had followed Bangladesh (Table 1 ). This corresponds to a nearly 17% reduction in Iran’s excess mortality following Turkey’s and an 18% increase in excess mortality following Bangladesh’s rates. The reason for this is Turkey started their vaccination programme two months earlier than Bangladesh (and Iran) and had a much faster per capita roll-out rate. Following Argentina’s per capita roll-out rates, Iran would have only had a 4% reduction in excess mortality and averted as many as 12,600 (95% CI: 10,400–13,300) deaths despite the fact that Argentina, similar to Turkey, had an early vaccination start date. This is because Argentina had a much slower roll-out rate and, as a result, fewer deaths would have been averted during the Alpha and Delta waves in Iran, particularly in the older age groups (Table 2 ; also see Additional file 1 : Fig. S2 and S3).
We also found that following either Montenegro’s or Bolivia’s per capita roll-out rates, Iran could have averted many more deaths in the older age groups despite having a 17–20% lower percentage of fully vaccinated individuals (see Table 1 ). This is because faster roll-out rates based on these model countries would have enabled many more individuals to be protected in the > 50 age groups during the Alpha and Delta waves. However, due to their lower overall percentage of fully vaccinated individuals, as many as 8700 (95% CI: 2000–35,300) more individuals in the < 50 age groups could have lost their lives during the Omicron BA.1 wave (see Table 2 ). The reason for the wide uncertainty in the number of ACDs for these age groups is that if the vaccine effectiveness against death were very high (up to 90% protective against deaths), then having a lower coverage in younger age groups could have ended with many more deaths during the Omicron BA.1 wave (see the “ Methods ” section and Table 2 ).
To further investigate the impact of faster vaccine roll-out rates on ACDs in Iran, we also compared Bolivia’s and Nepal’s counterfactual vaccine roll-out rates. While both countries had similar overall outcomes in terms of cumulative ACDs by mid-April 2022 (see Table 1 ), following Nepal’s rates, Iran could have had as many as 6400 (1800–26,000) more deaths during Alpha and Delta waves in younger age groups. On the other hand, following Bolivia’s rates, Iran would have had only 900 (300–7600) more deaths over the same period and age groups (see Table 2 ). This is despite the fact that Bolivia would have had 16% lower overall coverage compared to Nepal under counterfactual scenairos (see Table 1 ). This is also the same reason why Iran performed much worse than many other LMICs and UMICs with similar or even lower percentages of fully vaccinated individuals because during these two waves, particularly Delta, where the country had its highest per capita death rates (see Additional file 1 : Fig. S2), it could have averted many more deaths if it were to vaccinate the population earlier.
Finally, we also compared Iran’s vaccination roll-out against Bahrain. This country had one of the fastest and most effective vaccination campaigns in the Eastern Mediterranean region [ 16 ], which can, therefore, provide a reasonable upper bound for the maximum number of ACDs in Iran. We found that following Bahrain’s model, a total of 75,300 (56,000–83,000) deaths could have been averted in Iran during the first 2 years of the pandemic --corresponding to approximately 26% reduction in Iran’s excess mortality (Table 1 ). The majority of these ACDs would have affected the > 50 age groups during the Alpha and Delta waves from mid-February to late December 2021 (Table 2 ; see also Additional file 1 : Fig. S3).
In this study, we developed a quantitative framework to retrospectively estimate the number of COVID-19 vaccine-preventable deaths in Iran by using per-capita vaccine roll-out rates from other countries as a basis for comparison. Iran was selected as a case study due to the delayed start of its national vaccination campaign which has been suggested to have contributed to a higher mortality and disease burden [ 20 , 32 , 34 ]. Our framework provides a simple and rapid assessment to gauge the impact of relative vaccination coverage, pace, and timing from different countries on avertable deaths. The method provides insight into the identification of countries with effective vaccination programmes, a basis for evaluating the impact of specific policies related to vaccination roll-out speed and timing, and encourages transparency and accountability in the management of vaccination programmes. The applicability of our method extends beyond the COVID-19 pandemic and can also aid future decisions around vaccination strategies aimed at reducing the burden of other infectious diseases with epidemic and/or pandemic potential such as polio, influenza, and measles where vaccination timing and coverage among targeted age-groups is important [ 35 , 36 , 37 ].
Our findings revealed that had Iran followed the per capita roll-out rates of certain model countries, it could have potentially averted a significantly higher number of deaths, particularly during the first 9 months of 2021, due to their faster COVID-19 vaccine roll-out rates. The impact of faster roll-outs would have been far greater in averting deaths in the older age groups due to their elevated infection fatality rate [ 38 , 39 ], whereas achieving higher overall coverage would have been more effective in preventing deaths in younger age groups. For instance, our results indicated that Iran could have saved nearly 31,000 more lives if it had followed the fast roll-out rates of Montenegro despite having a 20% lower overall coverage. This is because many more lives could have been saved in the older age groups during the Alpha and Delta waves despite having more deaths in the younger age groups during the Omicron BA.1 wave in Iran.
Comparisons with Bahrain, a country with a highly effective vaccination campaign, demonstrated that nearly 75,000 more deaths could have been averted in Iran by following a similar model. These findings showed the potential benefits of early vaccination start dates but more importantly faster roll-out rates in reducing the disease burden. Another example of an early and effective vaccination campaign is Israel which had a swift vaccination roll-out using predominantly mRNA vaccines. It experienced markedly lower per capita mortality rates during the Delta and Omicron waves compared to many other countries with slower vaccination rates [ 40 ]. These observations also align with the findings from observational studies showing that highly vaccinated communities experience significantly fewer deaths [ 41 ].
A key strength of the study lied in our reliance on excess mortality estimates rather than reported deaths for calculating ACDs, a method that has been shown to reliably capture the true death toll from COVID-19 in Iran as it avoids under-reporting biases [ 17 , 32 , 42 ]. Our estimates for ACDs should be interpreted as a conservative lower bound, as we did not incorporate the indirect effects of vaccination on reducing transmission rates and the complex interplay of other non-pharmaceutical interventions on lowering virus circulation which can in turn further contribute to increasing ACDs. To address these limitations, a comprehensive transmission dynamic model would be necessary, demanding rich data on parameters such as population infection history, waning immunity, and vaccine effectiveness across variants and vaccine types, a wealth of information lacking for many resource-constrained regions, including Iran [ 10 ]. Therefore, given the dearth of empirical data for precise parameter estimations [ 42 ] and risk of model over-parametrisation, we believe our model provides a fast and simple framework for estimating ACDs for Iran and other countries with similar limitations using excess mortality data which is readily available for most countries around the world and does not greatly suffer from under-reporting biases [ 43 , 44 ].
One of the major contributors to the delayed vaccination programme in Iran was the prioritisation of domestic vaccine production over securing early deals with other vaccine producers through COVAX and bilateral agreements [ 45 ], particularly the emphasis on the local production of BIV1-COvIran vaccine from Barekat Pharmaceutical, a home-grown vaccine company which faced scaling challenges as well as concerns over transparency and data sharing [ 46 , 47 ]. Among the other missed opportunities in Iran’s vaccination programme in 2021 was the banning of 150,000 doses of the Pfizer-BioNTech vaccine donated through Iran’s Red Crescent organisation which could have prevented thousands of COVID-19 deaths [ 20 ]. Given that mRNA vaccines elicit stronger humoral immunity relative to inactivated virus vaccines [ 50 , 51 , 52 ], it is plausible that they would have prevented more infections and deaths if they were more widely used or in combination with inactivated virus vaccines as heterologous vaccine regimens [ 53 ]. Another example of such missed opportunities was the decision not to participate in phase 3 clinical trials of inactivated virus vaccines. Iran’s Ministry of Health had a policy to only participate in such trials only if the manufacturer made a commitment to jointly collaborate with Iran in the technology transfer and production of vaccines. Such an agreement was only reached with the Finley Institute of Cuba which ran its phase 3 trials in Iran [ 48 , 49 ]. However, this vaccine was not granted emergency use authorisation until much later and without any large importations from Cuba or local production in Iran, making up only 1.7% of all administered vaccine doses in Iran (see Supplementary Table 2 ) [ 20 ].
In contrast, some of the model countries included in this study such as Argentina, Bahrain, and Turkey all participated in trials for inactivated virus vaccines and benefited from securing more doses at earlier dates compared to Iran. This highlights the advantages of participating in such clinical trials for faster vaccine rollouts. Bangladesh, on the other hand, did not participate in any phase 3 trials [ 54 ] and Nepal’s vaccination roll-out was in part affected by the halting of vaccine exports from India which the country relied on in 2021 [ 55 ]. Additionally, Montenegro’s swift vaccine roll-out, despite not participating in clinical trials, underscores the importance of rapid deployment of vaccines, especially in smaller-sized nations [ 56 ].
Closer examination of Iran’s COVID-19 vaccination experience also provides valuable insights for future public health policy decisions. The delay in initiating the vaccination campaign resulted from preventable factors, including the prohibition of vaccine imports from certain countries despite their proven safety and efficacy, delayed efforts to secure vaccine doses, and an over-reliance on the punctual delivery of domestically developed vaccines. These factors contributed to a lower number of vaccine-preventable deaths in Iran, but their individual impact is not quantified in this study. The causes of delay highlight undue political interference in the health sector, the absence of independent bodies to challenge politically motivated decisions, and a lack of transparency to address corruption allegations. The study underscored the importance of implementing the consensual and culturally sensitive principle of ‘Independent Review’ through the establishment of independent research committees, institutional review boards, and academic organisations, serving as key lessons in research ethics and public health ethics [ 57 ].
In conclusion, this study provided a quantitative framework to compare the performance of different countries based on their timing, pace, and coverage of vaccination and their impact on avertable COVID-19 deaths. It demonstrated the critical role of faster roll-out rates on further reducing COVID-19 deaths, particularly during the waves of infection with the Alpha and Delta variants in older age-groups with highest risk of severe disease outcome and deaths and provided a means to find effective vaccination strategies in managing infectious disease epidemics based on country-level comparisons. More broadly, our modelling framework can provide a tool for policymakers and public health officials to assess the successes and challenges of other countries, address disparities in vaccination, evaluate policy effectiveness, make data-driven decisions, and prepare for future health emergencies.
Availability of data and materials
All software code and analysis scripts are available in Mathematica version 11 on GitHub ( https://github.com/mg878/Vaccine_preventable_Deaths ). All-cause mortality data is available on Iran’s National Organisation for Civil Registration ( https://www.sabteahval.ir ). Number of fully vaccinated individuals are available from Our World in Data ( https://ourworldindata.org/coronavirus ).
Statement on the fifteenth meeting of the IHR (2005) Emergency Committee on the COVID-19 pandemic. https://www.who.int/news/item/05-05-2023-statement-on-the-fifteenth-meeting-of-the-international-health-regulations-(2005)-emergency-committee-regarding-the-coronavirus-disease-(covid-19)-pandemic . Accessed 28 May 2023.
Lazarus JV, Romero D, Kopka CJ, Karim SA, Abu-Raddad LJ, Almeida G, et al. A multinational Delphi consensus to end the COVID-19 public health threat. Nature. 2022;611:332–45.
Article CAS PubMed PubMed Central Google Scholar
Watson OJ, Barnsley G, Toor J, Hogan AB, Winskill P, Ghani AC. Global impact of the first year of COVID-19 vaccination: a mathematical modelling study. Lancet Infect Dis. 2022;22:1293–302.
Notarte KI, Catahay JA, Velasco JV, Pastrana A, Ver AT, Pangilinan FC, et al. Impact of COVID-19 vaccination on the risk of developing long-COVID and on existing long-COVID symptoms: A systematic review. EClinicalMedicine. 2022;53:101624.
Article PubMed PubMed Central Google Scholar
Wouters OJ, Shadlen KC, Salcher-Konrad M, Pollard AJ, Larson HJ, Teerawattananon Y, et al. Challenges in ensuring global access to COVID-19 vaccines: production, affordability, allocation, and deployment. Lancet. 2021. https://doi.org/10.1016/S0140-6736(21)00306-8 .
Bollyky TJ, Gostin LO, Hamburg MA. The Equitable distribution of COVID-19 therapeutics and vaccines. JAMA. 2020;323:2462–3.
Article CAS PubMed Google Scholar
Fair allocation mechanism for COVID-19 vaccines through the COVAX Facility. https://www.who.int/publications/m/item/fair-allocation-mechanism-for-covid-19-vaccines-through-the-covax-facility . Accessed 28 May 2023.
Wiysonge CS, Ndwandwe D, Ryan J, Jaca A, Batouré O, Anya B-PM, et al. Vaccine hesitancy in the era of COVID-19: could lessons from the past help in divining the future? Hum Vaccin Immunother. 2022;18:1–3.
Vilches TN, Moghadas SM, Sah P, Fitzpatrick MC, Shoukat A, Pandey A, et al. Estimating COVID-19 Infections, hospitalizations, and deaths following the US Vaccination campaigns during the pandemic. JAMA Netw Open. 2022;5:e2142725.
Jia KM, Hanage WP, Lipsitch M, Johnson AG, Amin AB, Ali AR, et al. Estimated preventable COVID-19-associated deaths due to non-vaccination in the United States. Eur J Epidemiol. 2023;24:1–4.
Meslé MM, Brown J, Mook P, Hagan J, Pastore R, Bundle N, et al. Estimated number of deaths directly averted in people 60 years and older as a result of COVID-19 vaccination in the WHO European Region, December 2020 to November 2021. Euro Surveill. 2021;26:2101021
Liu Y, Procter SR, Pearson CAB, Montero AM, Torres-Rueda S, Asfaw E, et al. Assessing the impacts of COVID-19 vaccination programme’s timing and speed on health benefits, cost-effectiveness, and relative affordability in 27 African countries. BMC Med. 2023;21:85.
Sah P, Vilches TN, Moghadas SM, Fitzpatrick MC, Singer BH, Hotez PJ, et al. Accelerated vaccine rollout is imperative to mitigate highly transmissible COVID-19 variants. EClinicalMedicine. 2021;35:100865.
Bauer S, Contreras S, Dehning J, Linden M, Iftekhar E, Mohr SB, et al. Relaxing restrictions at the pace of vaccination increases freedom and guards against further COVID-19 waves. PLoS Comput Biol. 2021;17:e1009288.
Ferreira LS, Darcie Marquitti FM, Paixão da Silva RL, Borges ME, Ferreira da Costa Gomes M, Cruz OG, et al. Estimating the impact of implementation and timing of the COVID-19 vaccination programme in Brazil: a counterfactual analysis. Lancet Reg Health Am. 2023;17:100397.
Mathieu E, Ritchie H, Rodés-Guirao L, Appel C, Giattino C, Hasell J, et al. Coronavirus pandemic (COVID-19). Our World in Data. 2020. Published online at OurWorldInData.org. Retrieved from: https://ourworldindata.org/coronavirus .
Ghafari M, Kadivar A, Katzourakis A. Excess deaths associated with the Iranian COVID-19 epidemic: a province-level analysis. Int J Infect Dis. 2021;107:101–15.
Callaway E. The unequal scramble for coronavirus vaccines - by the numbers. Nature. 2020;584:506–7.
Dyer O. COVID-19: Countries are learning what others paid for vaccines. BMJ. 2021;372:n281.
Article PubMed Google Scholar
Ghafari M, Rezaee-Zavareh MS, Dascalu S, Katzourakis A. Iran’s COVID-19 vaccination programme: using transparency to build public trust in immunisation. The BMJ. 2021. https://blogs.bmj.com/bmj/2021/08/03/irans-covid-19-vaccination-programme-using-transparency-to-build-public-trust-in-immunisation/ . Accessed 28 May 2023.
Iran bans import of UK and US COVID-19 vaccines, saying they’re “completely untrustworthy.” France 24. 2021. https://www.france24.com/en/middle-east/20210109-iran-bans-import-of-uk-and-us-covid-19-vaccines-saying-they-re-completely-untrustworthy . Accessed 28 May 2023.
Iran receives first delivery of COVID-19 vaccines through COVAX facility. https://www.unicef.org/iran/en/press-releases/iran-receives-first-delivery-covid-19-vaccines-through-covax-facility . Accessed 31 May 2023.
The first shipment of COVAX vaccines. https://www.unicef.org/supply/stories/first-shipment-covax-vaccines . Accessed 31 May 2023.
First COVID-19 vaccines arrive in Malawi thanks to COVAX Facility. https://www.eeas.europa.eu/eeas/first-covid-19-vaccines-arrive-malawi-thanks-covax-facility_en . Accessed 31 May 2023.
Royal Society Expert Working Group. COVID-19: examining the effectiveness of non-pharmaceutical interventions. 2023.
Ghafari M, Hejazi B, Karshenas A, Dascalu S, Kadvidar A, Khosravi MA, et al. Lessons for preparedness and reasons for concern from the early COVID-19 epidemic in Iran. Epidemics. 2021;36:100472.
WHO Coronavirus (COVID-19) dashboard. https://covid19.who.int/ . Accessed 31 May 2023.
United Nations: Department of Economic and Social Affairs. World economic situation and prospects. New York. NY: United Nations; 2020. p. 2020.
Google Scholar
COVID-19 market dashboard. https://www.unicef.org/supply/covid-19-market-dashboard . Accessed 31 May 2023.
United Nations: Department of Economic and Social Affairs. World economic situation and prospects. New York. NY: United Nations; 2021. p. 2021.
United Nations: Department of Economic and Social Affairs. World economic situation and prospects. New York. NY: United Nations; 2022. p. 2022.
Ghafari M, Watson OJ, Karlinsky A, Ferretti L, Katzourakis A. A framework for reconstructing SARS-CoV-2 transmission dynamics using excess mortality data. Nat Commun. 2022;13:3015.
Al Kaabi N, Oulhaj A, Ganesan S, Al Hosani FI, Najim O, Ibrahim H, et al. Effectiveness of BBIBP-CorV vaccine against severe outcomes of COVID-19 in Abu Dhabi. United Arab Emirates Nat Commun. 2022;13:3215.
PubMed Google Scholar
Safavi-Naini SAA, Pourhoseingholi MA. The early impact of COVID-19 vaccination on deaths among elderly people in Iran. Gastroenterol Hepatol Bed Bench. 2022;15:103–5.
PubMed PubMed Central Google Scholar
Baptista Risi J, Jr. The control of poliomyelitis in Brazil. Rev Infect Dis. 1984;6(Suppl 2):S400–3.
Ferguson NM, Cummings DAT, Fraser C, Cajka JC, Cooley PC, Burke DS. Strategies for mitigating an influenza pandemic. Nature. 2006;442:448–52.
Noh J-W, Kim Y-M, Akram N, Yoo KB, Cheon J, Lee LJ, et al. Determinants of timeliness in early childhood vaccination among mothers with vaccination cards in Sindh province, Pakistan: a secondary analysis of cross-sectional survey data. BMJ Open. 2019;9:e028922.
COVID-19 Forecasting Team. Variation in the COVID-19 infection-fatality ratio by age, time, and geography during the pre-vaccine era: a systematic analysis. Lancet. 2022;399:1469–88.
Article PubMed Central Google Scholar
O’Driscoll M, Dos Santos GR, Wang L, Cummings DAT, Azman AS, Paireau J, et al. Age-specific mortality and immunity patterns of SARS-CoV-2. Nature. 2020. https://doi.org/10.1038/s41586-020-2918-0 .
Sandmann FG, Jit M. Rapid COVID-19 vaccine rollout: immense success but challenges ahead. Lancet Infect Dis. 2022;22:302–4.
Suthar AB, Wang J, Seffren V, Wiegand RE, Griffing S, Zell E. Public health impact of COVID-19 vaccines in the US: observational study. BMJ. 2022;377:e069317.
Ghafari M, Kadivar A, Katzourakis A. Estimates of anti-SARS-CoV-2 antibody seroprevalence in Iran. The Lancet Infectious Diseases. 2021;21:602–3
Msemburi W, Karlinsky A, Knutson V, Aleshin-Guendel S, Chatterji S, Wakefield J. The WHO estimates of excess mortality associated with the COVID-19 pandemic. Nature. 2023;613:130–7.
Karlinsky A, Kobak D. Tracking excess mortality across countries during the COVID-19 pandemic with the World Mortality Dataset. Elife. 2021;10:e69336.
Torbati Y. Amid covid surge, Iran cut corners to approve yet-unproven vaccine. The Washington Post. 2022. https://www.washingtonpost.com/world/2022/08/20/iran-covid-vaccine-approval/ .
Rovetta A, Garavaglia R, Vitale A, Meccia E, Tesfaye BT, Mezzana P, et al. An improved peer-review system to compensate for scientific misconduct in health-sensitive topics. 2022;44:1605601.
Negotiating with major vaccine manufacturers in the world [Persian]. https://dolat.ir/detail/344397 . Accessed 31 May 2023.
Mok CKP, Cohen CA, Cheng SMS, Chen C, Kwok K-O, Yiu K, et al. Comparison of the immunogenicity of BNT162b2 and CoronaVac COVID-19 vaccines in Hong Kong. Respirology. 2022;27:301–10.
Premikha M, Chiew CJ, Wei WE, Leo YS, Ong B, Lye DC, et al. Comparative effectiveness of mRNA and inactivated whole-virus vaccines against Coronavirus disease 2019 infection and severe disease in Singapore. Clin Infect Dis. 2022;75:1442–5.
Tan CY, Chiew CJ, Lee VJ, Ong B, Lye DC, Tan KB. Comparative effectiveness of 3 or 4 doses of mRNA and inactivated whole-virus vaccines against COVID-19 infection, hospitalization and severe outcomes among elderly in Singapore. Lancet Reg Health West Pac. 2022;29:100654.
Au WY, Cheung PP-H. Effectiveness of heterologous and homologous COVID-19 vaccine regimens: living systematic review with network meta-analysis. BMJ. 2022;377:e069989.
Mostafavi E, Eybpoosh S, Karamouzian M, Khalili M, Haji-Maghsoudi S, Salehi-Vaziri M, et al. Efficacy and safety of a protein-based SARS-CoV-2 vaccine: a randomized clinical trial. JAMA Netw Open. 2023;6:e2310302.
Iranian Registry of Clinical Trials (IRCT). https://www.irct.ir/trial/54833 . Accessed 29 May 2023.
Islam MR, Hasan M, Nasreen W, Tushar MI, Bhuiyan MA. The COVID-19 vaccination experience in Bangladesh: Findings from a cross-sectional study. Int J Immunopathol Pharmacol. 2021;35:20587384211065628.
Kansakar S, Dumre SP, Raut A, Huy NT. From lockdown to vaccines: challenges and response in Nepal during the COVID-19 pandemic. Lancet Respir Med. 2021;9:694–5.
Calleja N, Gualtieri A, Terzic N, Scoutellas V, Calleja-Agius J. Managing COVID-19 in four small countries: initial response to the pandemic in San Marino, Montenegro. Malta and Cyprus Health Policy. 2022;126:281–6.
Universal Declaration on bioethics and Human Rights. 2005. https://www.unesco.org/en/legal-affairs/universal-declaration-bioethics-and-human-rights?hub=66535 . Accessed 10 Jun 2023.
Covid-19 Update: Islamic Republic of Iran (No. 832 / 31 May 2022). World Health Organization - Regional Office for the Eastern Mediterranean. http://www.emro.who.int/iran .
Hodcroft E. CoVariants: SARS-CoV-2 mutations and variants of interest. https://covariants.org/ . Accessed 18 Jan 2023.
Download references
Acknowledgements
M.G. would like to thank Oliver Watson for analysing and sharing of data on the global impact of vaccination and Ariel Karlinsky, member of the World Health Organization Technical Advisory Group on COVID-19 Mortality Assessment, and the Center for Interdisciplinary Data Science Research (CIDR) at the Hebrew University of Jerusalem for analysing and sharing of excess mortality data.
M.G. was funded by the Biotechnology and Biological Science Research Council (BBSCR) grant number BB/M011224/1.
Author information
Authors and affiliations.
Big Data Institute and Pandemic Sciences Institute, Nuffield Department of Medicine, University of Oxford, Oxford, UK
Mahan Ghafari
Department of Biology, University of Oxford, Oxford, UK
Mahan Ghafari & Stefan Dascalu
School of Dentistry, The University of Queensland, Herston, QLD 4006, Australia
Sepanta Hosseinpour
Middle East Liver Diseases (MELD) Center, Tehran, Iran
Mohammad Saeid Rezaee-Zavareh
Department of Medicine, National Heart and Lung Institute, Imperial College London, London, UK
Somayeh Rostamian
The James F. Drane Bioethics Institute, PennWest University, Edinboro, PA, USA
Kiarash Aramesh
United Nations University Institute for Water, Environment and Health (UNU-INWEH), Hamilton, ON, Canada
Kaveh Madani
Comprehensive Cancer Centre, School of Cancer and Pharmaceutical Sciences, King’s College London, London, UK
Shahram Kordasti
You can also search for this author in PubMed Google Scholar
Contributions
Writing (original draft): M.G. and S.K.; analysis and study design: M.G.; data collection: M.G., S.H., M.S.R., S.R.; writing (review and editing): M.G., S.H., M.S.R., S.D., K.A., K.M, and S.K.; funding acquisition: S.K.; supervision: M.G. and S.K. All authors read and approved the final manuscript.
Corresponding authors
Correspondence to Mahan Ghafari or Shahram Kordasti .
Ethics declarations
Ethics approval and consent to participate.
Not applicable. The analysis in this study only uses publicly available data sources.
Consent for publication
Not applicable. This manuscript does not contain any individual person’s data in any form.
Competing interests
The authors declare no competing interests.
Additional information
Publisher’s note.
Springer Nature remains neutral with regard to jurisdictional claims in published maps and institutional affiliations.
Supplementary Information
Additional file 1: table s1..
A timeline of vaccine imports to Iran from 3 February 2021 to 16 November 2021. Table S2. Total number of administered doses of COVID-19 vaccines in Iran per vaccine name by 29 May 2022 [ 58 ]. Table S3. Iran’s vaccine roll-out dates per age-group [ 32 ]. Figure S1. Deaths averted by vaccination for upper and lower middle income countries, including Iran, up to 8 December 2021. Data on deaths averted per person and per vaccine are downloaded from [ 3 ]. The economic status of each country is based on the World Bank income groups in 2020 [ 28 ]. Figure S2. Association between reported cases, deaths, and excess deaths over time and with respect to variants of SARS-CoV-2. (A) Reported cases, deaths, and excess deaths (as quantified by the sum of excess deaths per age group) as percentage of highest peak (Delta) in Iran over time. (B) Proportion of the total number of SARS-CoV-2 variants over time in Iran. Data obtained from [ 59 ]. Figure S3. Avertable COVID-19 deaths in Iran over time based on the per capita vaccine roll-out rates from model countries. Top panel shows Iran’s weekly excess deaths (black) and counterfactual excess deaths (magenta) had it followed the vaccination rate for each model country. Shaded areas show the 95% confidence interval for the counterfactual excess deaths based on varying degree of vaccine effectiveness against deaths. Central panel shows the avertable deaths based on vaccination rates from a given model country. It shows the difference between weekly excess deaths and counterfactual excess deaths in the top panel. Bottom panel shows the percentage of excess vaccination for a given model country relative to Iran’s vaccination rates per age group. Shaded areas in green (red) show periods where there would have been more (less) vaccination had Iran followed the vaccination rates as the model country.
Rights and permissions
Open Access This article is licensed under a Creative Commons Attribution 4.0 International License, which permits use, sharing, adaptation, distribution and reproduction in any medium or format, as long as you give appropriate credit to the original author(s) and the source, provide a link to the Creative Commons licence, and indicate if changes were made. The images or other third party material in this article are included in the article's Creative Commons licence, unless indicated otherwise in a credit line to the material. If material is not included in the article's Creative Commons licence and your intended use is not permitted by statutory regulation or exceeds the permitted use, you will need to obtain permission directly from the copyright holder. To view a copy of this licence, visit http://creativecommons.org/licenses/by/4.0/ . The Creative Commons Public Domain Dedication waiver ( http://creativecommons.org/publicdomain/zero/1.0/ ) applies to the data made available in this article, unless otherwise stated in a credit line to the data.
Reprints and permissions
About this article
Cite this article.
Ghafari, M., Hosseinpour, S., Rezaee-Zavareh, M.S. et al. A quantitative evaluation of the impact of vaccine roll-out rate and coverage on reducing deaths: insights from the first 2 years of COVID-19 epidemic in Iran. BMC Med 21 , 429 (2023). https://doi.org/10.1186/s12916-023-03127-8
Download citation
Received : 12 June 2023
Accepted : 23 October 2023
Published : 13 November 2023
DOI : https://doi.org/10.1186/s12916-023-03127-8
Share this article
Anyone you share the following link with will be able to read this content:
Sorry, a shareable link is not currently available for this article.
Provided by the Springer Nature SharedIt content-sharing initiative
- Vaccination
- Excess mortality
- Counterfactual scenarios
- Decision-making
BMC Medicine
ISSN: 1741-7015
- Submission enquiries: [email protected]
- General enquiries: [email protected]
Thank you for visiting nature.com. You are using a browser version with limited support for CSS. To obtain the best experience, we recommend you use a more up to date browser (or turn off compatibility mode in Internet Explorer). In the meantime, to ensure continued support, we are displaying the site without styles and JavaScript.
- View all journals
- My Account Login
- Explore content
- About the journal
- Publish with us
- Sign up for alerts
- Open access
- Published: 27 May 2024
Safety outcomes following COVID-19 vaccination and infection in 5.1 million children in England
- Emma Copland ORCID: orcid.org/0000-0001-9280-5241 1 ,
- Martina Patone 1 ,
- Defne Saatci ORCID: orcid.org/0000-0002-2414-2906 1 ,
- Lahiru Handunnetthi 2 , 3 ,
- Jennifer Hirst 1 ,
- David P. J. Hunt ORCID: orcid.org/0000-0003-4230-0207 4 ,
- Nicholas L. Mills ORCID: orcid.org/0000-0003-0533-7991 5 , 6 ,
- Paul Moss ORCID: orcid.org/0000-0002-6895-1967 7 ,
- Aziz Sheikh ORCID: orcid.org/0000-0001-7022-3056 1 , 6 ,
- Carol A. C. Coupland 1 , 8 ,
- Anthony Harnden 1 ,
- Chris Robertson ORCID: orcid.org/0000-0001-6848-5241 9 &
- Julia Hippisley-Cox ORCID: orcid.org/0000-0002-2479-7283 1
Nature Communications volume 15 , Article number: 3822 ( 2024 ) Cite this article
479 Accesses
168 Altmetric
Metrics details
- Epidemiology
- Public health
The risk-benefit profile of COVID-19 vaccination in children remains uncertain. A self-controlled case-series study was conducted using linked data of 5.1 million children in England to compare risks of hospitalisation from vaccine safety outcomes after COVID-19 vaccination and infection. In 5-11-year-olds, we found no increased risks of adverse events 1–42 days following vaccination with BNT162b2, mRNA-1273 or ChAdOX1. In 12-17-year-olds, we estimated 3 (95%CI 0–5) and 5 (95%CI 3–6) additional cases of myocarditis per million following a first and second dose with BNT162b2, respectively. An additional 12 (95%CI 0–23) hospitalisations with epilepsy and 4 (95%CI 0–6) with demyelinating disease (in females only, mainly optic neuritis) were estimated per million following a second dose with BNT162b2. SARS-CoV-2 infection was associated with increased risks of hospitalisation from seven outcomes including multisystem inflammatory syndrome and myocarditis, but these risks were largely absent in those vaccinated prior to infection. We report a favourable safety profile of COVID-19 vaccination in under-18s.
Similar content being viewed by others

Neurological complications after first dose of COVID-19 vaccines and SARS-CoV-2 infection
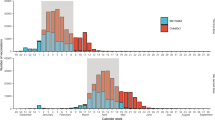
Comparative effectiveness of the BNT162b2 and ChAdOx1 vaccines against Covid-19 in people over 50
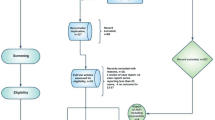
Systematic review and meta-analysis of myocarditis and pericarditis in adolescents following COVID-19 BNT162b2 vaccination
Introduction.
The United Kingdom (UK) approved COVID-19 vaccination for all children aged 12 and over in September 2021. This was extended to 5–11-year-olds in April 2022 in a one-off programme offering a primary course of COVID-19 vaccination to children who were not in a vulnerable or high-risk group 1 . Despite uptake being very high in adults, with over 80% receiving at least one dose of COVID-19 vaccine as of 11th May 2023, uptake has been lower in children, with 62% of 16–17-year-olds, 46% of 12–15-year-olds and 10% of 5–11-year-olds being vaccinated against COVID-19 2 . In the UK, the vast majority of vaccinated children received the BNT162b2 (Pfizer/BioNTech) COVID-19 vaccine, as the UK Joint Committee on Vaccination and Immunisation (JCVI) advised against using ChAdOX1 (AstraZeneca) in people under 40 or mRNA-1273 (Moderna/SpikeVax) in children under 18 during the time period that most children were vaccinated 3 .
In November 2022, the JCVI recommended that 16–49-year-olds who are not in a clinical risk group should no longer be offered a third dose of vaccine from February 2023, and that primary course vaccination in 5–49-year-olds should be targeted to groups at high risk of severe COVID-19 4 . They have advised to continue vaccinating clinically vulnerable children aged 6 months and above and to vaccinate otherwise healthy children aged 12 years and above living with immunosuppressed individuals 4 .
Although the benefits of COVID-19 vaccines in older adults clearly outweigh the risks of rare complications 5 , 6 , 7 , 8 , the balance of risks and benefits in young people remains uncertain. Whilst clinical trials have demonstrated the effectiveness of COVID-19 vaccines in reducing the risk of severe COVID-19 in children aged 5–15 years, the absolute risk of severe outcomes, including hospitalisation, intensive care unit (ICU) admission and death, following infection is low 9 , 10 , 11 , 12 . A serious consequence of SARS-CoV-2 infection in children is multisystem inflammatory syndrome (MIS-C) 13 , 14 , which can lead to coronary artery aneurysms, cardiac dysfunction, and multiorgan inflammatory manifestations 15 . Post-COVID syndrome, or long COVID, is another serious outcome of SARS-CoV-2 infection 16 . The possible impact of long COVID in children is still unclear, but it is estimated to affect 10% of those infected by SARS-CoV-2 and could potentially result in lifelong illness 16 , 17 . COVID-19 vaccines in childhood may reduce the risk of MIS-C 13 and long COVID 17 , and secondary benefits might include increasing overall population immunity, thereby minimising disruptions to education and maintaining overall well-being and health within this age group.
Concerns around vaccine safety have been identified as a barrier to COVID-19 vaccine acceptance, particularly in the context of parents and guardians giving consent for their children to be vaccinated 18 , 19 , 20 . An increased risk of myocarditis has been consistently reported after the delivery of mRNA vaccines, predominantly affecting males aged 18 to 35 years and most notably after the second dose 21 , 22 . This elevated risk of mRNA vaccination-associated myocarditis has also been reported in adolescent males, although these cases have generally been mild and the long-term prognosis is favourable 23 , 24 , 25 , 26 , 27 , 28 , 29 , 30 , 31 , 32 . No serious safety concerns have yet been raised in younger children; however, population-based studies assessing the risk of adverse events following vaccination compared with an unvaccinated group are lacking 33 , 34 , 35 .
In light of the JCVI’s advice to continue vaccinating specific groups of children against COVID-19, and the potential for future mass vaccination programmes if new SARS-CoV-2 variants emerge or the number of severe COVID-19 cases increase, it remains important to quantify the overall risks and benefits of COVID-19 vaccination in this age group to inform future vaccine policy. Therefore, our primary aim was to investigate and compare the risks of pre-specified vaccine safety outcomes following vaccination with BNT162b2, mRNA-1273 and ChAdOX1 in children. We also aimed to compare the risks of these safety outcomes following SARS-CoV-2 infection in vaccinated and unvaccinated children, as a guide to inform global public health policy considerations.
We used the English National Immunisation Management Service (NIMS) database of COVID-19 vaccination, linked at the individual-level to national data for mortality, hospital admissions, and SARS-CoV-2 infection. We undertook a self-controlled case series design, originally developed to examine vaccine safety 36 , 37 , to investigate the association between COVID-19 vaccines available in the UK between 8th December 2020 and 7th August 2022 (BNT162b2, mRNA-1273 and ChAdOx1) and hospitalisation with the following pre-specified outcomes: myocarditis 21 , 22 , MIS-C 38 , immune thrombocytopenia (ITP) 39 , epilepsy 40 , acute pancreatitis 41 , acute disseminated encephalomyelitis (ADEM) 42 , Guillain-Barre syndrome 43 , appendicitis 44 , demyelinating disease 6 , myositis 45 , angioedema 46 and anaphylaxis 46 . We also investigated the association of SARS-CoV-2 infection with these outcomes in children who had been vaccinated prior to infection compared to those who were unvaccinated at time of infection. We compared the incidence of hospitalisation from each outcome in the six weeks following vaccination or SARS-CoV-2 infection relative to the baseline period, and estimated the absolute risk as the excess number of events expected per million children exposed. We also conducted a matched cohort analysis using vaccinated and unvaccinated children included the QResearch primary care database to improve the robustness of the study.
Number of children receiving COVID-19 vaccines
A total of 5,197,925 young people aged 5–17 years, comprising 1,842,159 children aged 5–11 years and 3,355,766 adolescents aged 12–17 years were included in the study. 4,347,781 young adults aged 18–24 years, were included as a comparison. The characteristics of the young people included in the study are detailed in Table 1 and Supplementary Table 1 .
In children aged 5–11 years, 32% ( n = 581,545) received at least one dose of COVID-19 vaccine and 16% ( n = 303,118) received a second dose within the study period. Over 99.9% of 5–11-year-olds who received at least one COVID-19 vaccine dose received the BNT162b2 vaccine (Table 2 ). 82% ( n = 1,508,661) of children in this age group had a positive SARS-CoV-2 test recorded between 8th December 2020 and 7th August 2022, 0.4% ( n = 5665) of whom received their first COVID-19 vaccine dose prior to their first recorded positive SARS-CoV-2 test (Table 2 ).
In adolescents aged 12–17 years, 86% ( n = 2,882,229) received a first dose of COVID-19 vaccine, 67% ( n = 2,254,214) received a second dose and 14% ( n = 454,868) received a third dose (Table 2 ).
The characteristics of the population excluded from the self-controlled case series analysis (i.e. those who did not receive any COVID-19 vaccine and did not have a positive SARS-CoV-2 test recorded during the study period) are presented in Supplementary Table 2 .
Risk of pre-specified safety outcomes following COVID-19 vaccination in children aged 5–11 years
In children aged 5–11 years, we did not observe an increased risk of any of the pre-specified outcomes in the 1–42 days following any dose COVID-19 vaccine with BNT162b2, mRNA-1273 or ChAdOX1 (Table 3 ). However, given that less than 0.1% of vaccinated 5-11-year-olds received a ChAdOX1 or mRNA-1273 vaccine, the probability of type II errors was high as the sample size was too small to detect statistically significant associations for these vaccines.
The clinical characteristics of all children hospitalised with a pre-specified safety event are shown in Supplementary Table 3 . Supplementary Tables 4 – 7 show the incidence rate ratios (IRR) and 95% confidence intervals (CI) for all outcomes 1–42 days and in weekly risk periods following each vaccine dose in 5–11-year-olds in males and females separately, and the effect of ethnicity on the risk of each outcome.
Risks of pre-specified safety outcomes following COVID-19 vaccination in adolescents aged 12–17 years
In the 1–42 days after the first and second doses of BNT162b2, we observed an increased risk of myocarditis in adolescents aged 12–17 years (IRR 1.92, 95%CI 1.08–3.43 and IRR 2.96, 95%CI 1.65–5.32 for first and second dose, respectively) (Table 4 ). We estimated that an additional 3 (95%CI 0–5) cases per million exposed would be anticipated after the first dose and 5 (95%CI 3–6) after the second dose (Fig. 1 ). When we split the risk period into weekly blocks, the increased risk was restricted to 1–14 days following each dose (Supplementary Table 8 ). There was also an increased risk of hospitalisation with epilepsy (IRR 1.17, 95%CI 1.00–1.37; excess events per million: 12, 95%CI 0–23) in the 1–42 days following the second dose of BNT162b2 (Table 4 , Fig. 1 ).
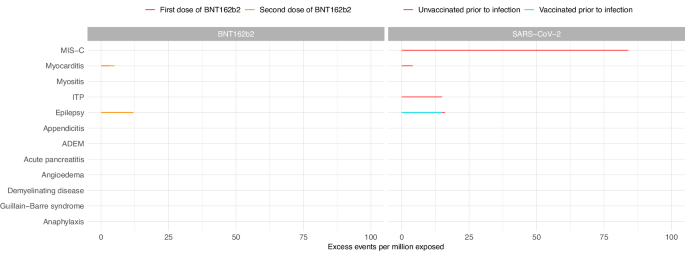
Estimated number of excess events per million (95% CI) based on incidence rate ratios of each outcome in the 1–42 days following vaccination or SARS-CoV-2 positive test compared to baseline period are presented where there were at least five events during the exposure period and when number of excess events is greater than zero. Data available from 8th December 2020 and 7th August 2022. Table 4 contains the data presented in this figure. MIS-C Multisystem inflammatory syndrome; ITP Idiopathic or immune thrombocytopenic purpura, ADEM Acute disseminated encephalomyelitis.
In the sex-stratified analysis, the increased risk of myocarditis after the first dose of BNT162b2 was only observed in females (IRR 4.01, 95%CI 1.33–12.09; excess events per million: 3, 95%CI 1–4), while the increased risk following the second dose was observed in males only (IRR 2.87, 95%CI 1.50–5.51; excess events per million: 9, 95%CI 4–11) (Supplementary Figs. 1 & 2 , Supplementary Tables 4 & 5 ). We additionally observed an increased risk of demyelinating disease, restricted to females (IRR 2.41, 95%CI 1.06-5.48; excess events per million: 4, 95%CI 0–6) following the second dose of BNT162b2. Of the eight female adolescents who experienced demyelinating disease in the 1–42 days following a second dose of BNT162b2, five were coded as optic neuritis.
In a post hoc analysis investigating differences in risk between children of different ethnic backgrounds, we found that the risk of anaphylaxis following a second dose of BNT162b2 in adolescents with non-white ethnicity was higher relative to the risk in adolescents with white ethnicity (relative IRR 2.55, 95%CI 1.00–6.46) (Supplementary Table 6 ). However, when the analysis was restricted to the subgroup of adolescents from non-white ethnic backgrounds, the risk of anaphylaxis in the 1–42 days following a second dose of BNT162b2 was not significantly increased compared to the baseline period (IRR 1.69, 95%CI 0.80–3.54) (Supplementary Table 6 ). We did not identify any differences in vaccine safety between white and non-white ethnicity for any of the other pre-specified outcomes in under-18s.
We found no evidence for significantly increased risks for any of the pre-specified outcomes in the 1–42 days following a first, second or third dose of mRNA-1273 vaccine in 12–17-year-olds (Table 4 ). However, this analysis lacked power to detect statistically significant associations, except for very large effect sizes, as less than 0.1% of adolescents received a first or second dose of mRNA-1273 vaccine.
There was an increased risk of hospitalisation with epilepsy 1–42 days after a first dose of ChAdOX1 (IRR 1.93, 95%CI 1.10–3.39), with an additional 705 (95%CI 129–1033) cases expected per million exposed (Table 4 ). This increased risk was restricted to females in the sex-stratified analysis (IRR 2.26, 95%CI 1.03–4.94) with an additional 813 (95%CI 44–1164) hospitalisations with epilepsy expected per million female adolescents exposed (Supplementary Table 4 ).
We also observed an increased risk of appendicitis in the 1–42 days following the second dose of ChAdOX1 (IRR 4.64, 95%CI 1.77–12.17; excess events per million: 512, 95%CI 283–599) (Table 4 ).
The IRRs and 95% CIs for all outcomes 1–42 days and in weekly risk periods following each vaccine dose in 12–17-year-olds in males and females separately, and the effect of ethnicity on the risk of each outcome, are presented in Supplementary Figs. 1 & 2 , Supplementary Tables 4 – 6 & 8 .
Risks of pre-specified safety outcomes following COVID-19 infection in children aged 5–11 years
In children aged 5-11 years who had received at least one dose of COVID-19 vaccine before the date that their positive SARS-CoV-2 test was recorded, we did not observe increased risks of any of the pre-specified outcomes in the 1–42 days following SARS-CoV-2 infection. In children who had not been vaccinated against COVID-19 prior to infection, there was an increased risk of hospital admission with MIS-C following a SARS-CoV-2 positive test (IRR 11.52, 95%CI 9.25–14.36), with an additional 137 (95%CI 134–140) cases expected per million exposed (Table 3 , Fig. 2 ). In the sex-stratified analysis, the risk of MIS-C was slightly greater in male children (IRR 12.00, 95%CI 8.92–16.12; excess events per million: 162, 95%CI 157-166) compared to female children (IRR 11.13, 95%CI 7.96–15.57; excess events per million: 124, 95%CI 119–127) (Supplementary Figs. 3 & 4 , Supplementary Tables 4 & 5 ). The increased risk was mainly observed in the 22–42 days following the date that the positive SARS-CoV-2 test was recorded (Supplementary Table 7 ).
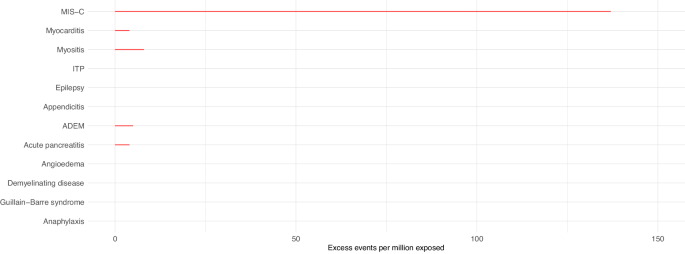
Estimated number of excess events per million (95% CI) based on incidence rate ratios of each outcome in the 1–42 days following SARS-CoV-2 positive test compared to baseline period are presented where there were at least five events during the exposure period and when number of excess events is greater than zero. Data available from 8th December 2020 and 7th August 2022. Table 3 contains the data presented in this figure. MIS-C Multisystem inflammatory syndrome, ITP Idiopathic or immune thrombocytopenic purpura, ADEM Acute disseminated encephalomyelitis.
We also observed increased risks of hospital admission for myositis, myocarditis, acute pancreatitis and ADEM following SARS-CoV-2 infection before vaccination (Table 3 , Fig. 2 ). In the sex-stratified analyses, we additionally identified increased risks of ITP (in both sexes) and anaphylaxis (in females only) (Supplementary Figs. 3 & 4 , Supplementary Tables 4 & 5 ).
The IRRs and 95% CIs for all outcomes 1–42 days following SARS-CoV-2 infection in 5–11-year-olds in males and females separately, and the effect of ethnicity on the risk of each outcome, are presented in Supplementary Figs. 3 & 4 , Supplementary Tables 4 , 5 , 6 & 7 .
Risks of pre-specified safety outcomes following COVID-19 infection in adolescents aged 12–17 years
In adolescents aged 12–17 years, we observed an increased risk of MIS-C (IRR 12.38, 95%CI 8.88–17.28; excess events per million: 84, 95%CI 81–86) in the 1–42 days following a SARS-CoV-2 infection in those who had not been vaccinated prior to SARS-CoV-2 infection (Table 4 , Fig. 1 ). In the sex-stratified analysis, male adolescents were at higher risk of MIS-C following infection compared to females (IRR 12.33, 95%CI 8.31–18.31 and IRR 13.11, 95%CI 6.90–24.91 in males and females, respectively), with an additional 131 (95%CI 126–135) cases expected per million males exposed compared to 48 (95%CI 44-50) in females (Supplementary Figs. 1 & 2 , Supplementary Tables 4 & 5 ).
We also observed increased risks of hospitalisation with myocarditis, ITP and epilepsy in the 1-42 days following SARS-CoV-2 infection in adolescents who had not been vaccinated against COVID-19 prior to infection as well as an increased risk of hospitalisation with epilepsy in those who had received at least one vaccine dose prior to infection (Table 4 , Fig. 1 ). In the sex-stratified analysis, the increased risks of hospitalisation with myocarditis and epilepsy were restricted to males while the increased risk of ITP following infection was only observed in females (Supplementary Figs. 1 & 2 , Supplementary Tables 4 & 5 ). We additionally identified increased risks of appendicitis (in females only) and anaphylaxis (in males only) following SARS-CoV-2 infection.
The IRRs and 95% CIs for all outcomes 1–42 days following SARS-CoV-2 infection in 12-17-year-olds in males and females separately, and the effect of ethnicity on the risk of each outcome, are presented in Supplementary Figs. 1 & 2 , Supplementary Tables 4 , 5 , 6 & 8 .
The results for all analyses in young adults aged 18-24 years are presented in Supplementary Tables 6 & 9 .
Robustness of the self-controlled case series results
The robustness of the results of the self-controlled case series analyses were assessed by (1) checking that the risk of outcomes during the pre-vaccination period (month prior to vaccination to account for potential bias of people with recent hospitalisation being less likely to get vaccinated) was lower than the baseline period and (2) checking that the risk of the positive control outcome (anaphylaxis) was higher following vaccination or SARS-CoV-2 infection than the baseline period. In the vast majority of analyses the estimates of the pre-vaccination period and the risk of anaphylaxis following vaccination or SARS-CoV-2 agreed with what was expected (Supplementary Tables 8 & 9 ).
Matched cohort study
Our matched cohort analysis included 1,580,869 children aged 5–11 years and 1,535,341 adolescents aged 12–17 years. Characteristics of the cohort are detailed in Supplementary Table 10 .
Incidence rates of vaccine safety outcomes in the 1–42 days following each vaccine dose and following SARS-CoV-2 infection in vaccinated and unvaccinated children are presented in Supplementary Table 11 . Incidence rates for all outcomes were significantly higher following SARS-CoV-2 infection compared to COVID-19 vaccination.
We matched 160,262 children aged 5–11 years and 848,186 adolescents aged 12–17 years who had received at least one dose of COVID-19 vaccine to a child of the same age and sex who had not received any COVID-19 vaccine doses by the date of the vaccinated child’s first vaccine dose (characteristics of matched cohort reported in Supplementary Table 12 ).
As in the self-controlled case series analysis, we identified an increased risk of hospitalisation with epilepsy in the 1–42 days following a second dose of COVID-19 vaccine with BNT162b2 in 12-17-year-olds (unadjusted IRR 1.77, 95%CI 1.05–2.99, adjusted IRR 3.88, 95%CI 1.27–11.86), but did not find significantly increased risks of appendicitis or myocarditis with BNT162b2 vaccination in adolescents (Supplementary Table 13 ).
We identified additional increased risks of anaphylaxis and appendicitis in 12-17-year-olds following a first dose of BNT162b2 (unadjusted IRR 3.71, 95%CI 1.23–11.14 and unadjusted IRR 1.37, 95%CI 1.05–1.80, respectively) and an increased risk of hospitalisation with epilepsy following a first dose with BNT162b2 in 5–11-year-olds, although the confidence interval was very wide reflecting the uncertainty of the estimate (unadjusted IRR 16.00, 95%CI 2.12–120.65) (Supplementary Table 13 ).
In general, the estimates from the matched cohort study were in agreement with the results from the self-controlled case series analysis in under-18s.
Unadjusted IRRs and IRRs adjusted for self-reported ethnicity (white, non-white, missing), quintile of deprivation (based on Townsend score) and presence of comorbidity (yes/no) for each outcome are reported in Supplementary Table 13 .
In our study of approximately 5.1 million young people aged 5–17 years, we have examined the risks of vaccine safety outcomes following COVID-19 vaccination and SARS-CoV-2 infection, stratified by age group (5–11 years and 12–17 years) and additionally by sex (males and females). We report several key findings that are relevant to public health policy makers. Firstly, we found no strong evidence for increased risks of any of the 12 safety outcomes investigated following COVID-19 vaccination in children aged 5–11 years. Second, in adolescents aged 12–17 years, we observed an increased risk of hospital admission for myocarditis following a first or second dose of BNT162b2, and an increased risk of hospitalisation with epilepsy and demyelinating disease (in females only) following a second dose of BNT162b2 in our primary analysis. Third, children and adolescents aged 5–17 years who had not received a COVID-19 vaccine dose prior to SARS-CoV-2 infection had an increased risk of hospitalisation from seven of the pre-specified safety outcomes including MIS-C and myocarditis. Finally, the risks of safety outcomes from SARS-CoV-2 infection were largely absent in 5–17-year-olds who received at least one COVID-19 vaccine dose prior to infection.
In our cohort of > 2.8 million vaccinated adolescents aged 12–17 years, we estimated 3 (95%CI 0–5) and 5 (95%CI 3–6) excess cases of myocarditis per million exposed in the 1–42 days following a first and second dose of BNT162b2, respectively. We did not observe an increased risk of myocarditis following vaccination with mRNA-1273 in adolescent males or females as some previous studies have reported 23 , 24 , 25 , 26 , 27 , 28 , 29 , 30 , 31 , 32 . However, the mRNA-1273 vaccine was only given to a relatively small number of adolescents in England, primarily for the third booster dose, therefore the study was underpowered to detect statistically significant associations, except for very large effect sizes. As expected, we observed a substantially increased risk of myocarditis in the 1–42 days following almost all doses of both mRNA vaccines in young adults aged 18–24 years compared to the baseline period, consistent with other studies reporting an increased risk of myocarditis in young adult males following a second mRNA-1273 vaccine dose 24 , 25 , 26 . Importantly, however, there were no deaths following a diagnosis of myocarditis in under-18s, and our findings are likely to reflect self-limiting disease.
We also observed a modest increased risk of hospitalisation with epilepsy in adolescents following a second dose of BNT162b2, with an additional 12 (95%CI 0-23) cases estimated per million exposed in 12–17-year-olds. However, a diagnosis of epilepsy is made over a period of time as it typically involves outpatient referral, a magnetic resonance imaging (MRI) scan and an electroencephalogram 47 . Therefore, this reported increased risk of epilepsy is highly unlikely to reflect new-onset epilepsy triggered by the vaccine. A limitation of the data was that we were only able to exclude prior hospital admissions with epilepsy (or any of the pre-specified outcomes) in the two years preceding the study start date. Therefore, the increase in admissions reported here is more likely to reflect seizures in children with an already underlying diagnosis of epilepsy or other chronic neurological condition but who hadn't been hospitalised in the previous two years, which we were unable to capture in our dataset, rather than new diagnoses.
Seizure exacerbation following COVID-19 vaccination has been reported in previous studies, but primarily in adults with epilepsy rather than children 40 . One survey of 224 children (median age 8 years) with epilepsy reported that 10% of those who had been living with epilepsy for more than two years or who had not been seizure-free in the year prior to vaccination had seizures in the month following the first and second dose of COVID-19 vaccine 48 . However, in children who were seizure-free for at least six months before they were vaccinated, the risk of seizures was decreased following vaccination 48 . Another survey of 278 people with Dravet Syndrome (with a median age 19 years) showed that there was increased seizure frequency following COVID-19 vaccination in 13% of the 120 participants who had received at least one vaccine dose 49 . Our findings suggest that the BNT162b2 vaccine is associated with a small increased risk of hospitalisation with epilepsy, but this risk is most likely restricted to children with pre-existing epilepsy and should be balanced against risks of hospitalisation through natural infection with SARS-CoV-2.
In the sex-stratified analysis, we identified a small increased risk of demyelinating disease in females following a second dose of BNT162b2, with an estimated 4 (95%CI 0-6) excess cases per million. To date there has been weak evidence linking mRNA vaccines with neuroimmunological disorders 50 , 51 , and a similar self-controlled case series in adults only identified links between the ChAdOX1 vaccine and neuroimmunological conditions 6 . The coding description demyelinating disease can refer to a spectrum of monophasic and chronic neuroinflammatory disorders, however, the majority of cases following BNT162b2 were coded as optic neuritis, which is typically monophasic and associated with good visual recovery in most childhood cases 52 . From the neurological perspective, this risk should be balanced against the protection vaccination offers against rare and more severe neuroimmunological sequelae of COVID-19 infection such as ADEM.
This study identified two strong safety signals in adolescents associated with the ChAdOX1 vaccine: appendicitis and epilepsy. A substantially increased risk of appendicitis was observed in adolescents following a second dose of ChAdOX1, with an additional 512 (95%CI 283–599) cases expected per million. This estimate is based on a small sample size as the ChAdOX1 vaccine was not approved for use in under-40s in the UK from April 2021 3 , 53 . Additionally, the increased risk was not identified in the matched cohort study, suggesting the evidence from this study for a causal association between appendicitis and ChAdOX1 vaccination is weak. Appendicitis was highlighted as an outcome of interest for vaccine safety by the US Food and Drug Administration following a clinical trial of BNT162b2, which reported a higher number of appendicitis cases in the vaccine arm compared to the placebo arm 44 . However, subsequent evidence from observational studies and adverse event reporting databases is conflicting, limited to adults and primarily focusing on mRNA vaccines 11 , 54 , 55 .
Following a first dose of ChAdOX1, we observed a substantially increased risk of hospitalisation with epilepsy, particularly in females, with 813 (95%CI 44–1164) excess cases estimated per million female adolescents vaccinated with a first dose of ChAdOX1, but as discussed above, these are not likely to reflect new diagnoses of epilepsy. The small sample size (only 0.3% of adolescents received ChAdOX1 for the first dose) and resulting wide confidence interval for this estimate should be noted. We also found that there was a higher proportion of adolescents with a hospital admission for epilepsy in the two years prior to the study start date who received ChAdOX1 for the first dose (2.7%), compared to BNT162b2 (0.2%) and mRNA-1273 (0.6%). While these individuals were excluded from the analysis, it is indicative that a higher proportion of adolescents who received a ChAdOX1 vaccine were included in a priority group, such as those with a chronic neurological disease including epilepsy 3 , compared to those who received mRNA vaccines and that the majority of hospitalisations with epilepsy following ChAdOX1 were likely in adolescents with a pre-existing condition. We did not identify an increased risk of hospitalisation with epilepsy following vaccination with ChAdOX1 in the matched cohort study. Our findings, together with a recent study that found evidence for increased risk of cardiac death in young women following a first dose of non-mRNA vaccine 56 , suggest that further work would need to be done to ensure the safety of ChAdOX1 in young people if it were to be used in future vaccination programmes.
In our cohort of > 580,000 children aged 5–11 years who were vaccinated against COVID-19, we found no evidence for increased risks of any of the pre-specified adverse events following any dose of BNT162b2, mRNA-1273 or ChAdOX1 vaccine 33 , 34 , 35 . Based on vaccine uptake rates reported by the UK Health Security Agency 2 , this analysis includes most vaccinated children in this age group in England, however, the lack of safety signals identified in this analysis could partially reflect the relatively small sample size due to the low uptake rate of COVID-19 vaccines in 5–11-year-olds. In the matched cohort study including > 160,000 vaccinated 5–11-year-olds, we additionally found an increased risk of hospital admission with epilepsy following a first dose with BNT162b2 compared to unvaccinated children. However, given that epilepsy was not identified as a safety signal in this age group in the self-controlled case series analysis and the lengthy diagnosis pathway for epilepsy as described above, this potential increased risk is most likely restricted to children with pre-existing epilepsy.
Following SARS-CoV-2 infection we observed increased risks of hospital admission from MIS-C, myocarditis, acute pancreatitis, myositis and ADEM in children aged 5–11 years who had not been vaccinated against COVID-19 prior to infection. Most notably, we estimated an additional 137 (95%CI 134–140) hospital admissions from MIS-C in the four to six weeks after the date of a positive test being reported per million children exposed. We also observed increased risks of MIS-C, myocarditis, ITP and hospitalisation with epilepsy in adolescents aged 12–17 years following SARS-CoV-2 infection in those who had not been vaccinated prior to infection. 84 (95%CI 81-86) additional cases of MIS-C per million exposed would be expected following SARS-CoV-2 infection in adolescents of this age group prior to vaccination.
However, in both children and adolescents, these increased risks of serious outcomes from SARS-CoV-2 infection were absent in those who received at least one dose of COVID-19 vaccine prior to infection. The exception was hospitalisation with epilepsy. Our analyses suggested that the risk of admission to hospital with epilepsy was increased following SARS-CoV-2 infection in adolescents aged 12–17 years who were not vaccinated against COVID-19 prior to infection, with an estimated additional 16 (95%CI 2–28) cases per million. A risk was still seen following infection in those who received at least one vaccine dose prior to infection, but at a slightly lower level, with an additional 15 (95%CI 1–24) hospital admissions with epilepsy per million. This study has shown that vaccination is associated with a significantly reduced risk of most SARS-CoV-2 complications in young people, particularly MIS-C, which can be fatal 15 .
This study has several strengths. First, this was a population-based study of prospectively recorded data not subject to recall and selection biases linked to case reports. Second, the large sample size allowed us to investigate rare outcomes, particularly following vaccination with BNT162b2, which could not be assessed through clinical trials. Third, the self-controlled case series study design removes potential confounding from fixed characteristics, and the additional breakdown of our study period into weekly blocks accounted for temporal confounding. We also assessed the robustness of our results through several sensitivity analyses, a matched cohort analysis and a parallel analysis in 18–24-year-olds to ensure that the results from our study were consistent with the current evidence base in adults.
There were also some limitations to this study. First, despite the large sample size, this study may have been under-powered to investigate rare outcomes in 5–11-year-olds due to the relatively small proportion of vaccinated children in this age group. Second, we relied on hospital admission codes and death certification to define the outcomes so our design will not have captured milder events only occurring in the community or only reported in GP records. This could have resulted in a misclassification bias. Third, we only had access to cases of SARS-CoV-2 infection confirmed with a reverse transcription polymerase chain reaction (RT-PCR) test in our database, which were more likely to happen in the early stages of the pandemic or in a hospital setting, and less likely to be used in schools and community settings where the vast majority of routine testing took place. We were also unable to account for unascertained SARS-CoV-2 infections, therefore, some of the adverse events could be misclassified as being associated with vaccination rather than a SARS-CoV-2 infection that was not recorded and the analysis of adverse outcomes following SARS-CoV-2 infection may have been biased by incomplete COVID-19 testing in the English population. Fourth, we were unable to determine the effect of SARS-CoV-2 variant on the risk of adverse events, as detailed data on the viral variant of SARS-CoV-2 underlying recorded infections was also not available in our database. Fifth, although we adjusted for seasonal effects in the self-controlled case series models, we did not explicitly investigate the effect of the wave of the pandemic during which the infection occurred on the risk of adverse events. For example, the incidence of MIS-C has been reported to be lower during the periods when Delta and Omicron variants were dominant, even before 12–15-year-olds started being vaccinated, compared to the period when the Alpha variant was dominant 57 . Given that > 98% of children in this study were vaccinated against COVID-19 during the periods that Delta and Omicron were dominant, we may expect a lower incidence of MIS-C following infection in vaccinated children, who were unlikely to have been infected with the Alpha variant, compared to unvaccinated children, who had a higher likelihood of being infected with the Alpha variant. Lastly, we were unable to assess differences in vaccine safety by level of deprivation, which should be prioritised as a future area of research.
In summary, we found no strong evidence for increased risks of 12 pre-specified vaccine safety outcomes following COVID-19 vaccination in children aged 5–11 years and no new significant safety concerns in 12–17-year-olds following vaccination with mRNA vaccines recommended for use in these age groups in the UK by the JCVI. Additionally, in unvaccinated children we found increased risks of hospitalisation from seven adverse outcomes including MIS-C and myocarditis following SARS-CoV-2 infection that were either not observed, or were reduced, following vaccination. Overall, our findings support a favourable safety profile of COVID-19 vaccination using mRNA vaccines in children and young people aged 5-17 years.
Ethics approval and consent to participate
The QResearch® ethics approval was provided by the East Midlands-Derby Research Ethics Committee [reference 18/EM/0400] and reviewed by the QResearch science committee [Project OX300]. Consent from participants was not required. The study was performed in accordance with the Declaration of Helsinki.
Data sources
We used the NIMS database of COVID-19 vaccination. We linked individual-level vaccination data to national data for mortality (Office for National Statistics), hospital admissions (Hospital Episode Statistics), and SARS-CoV-2 infection assessed by RT-PCR (Second Generation Surveillance System) using the unique NHS number. Additional analyses to assess robustness of the results made use of the QResearch database, which includes anonymised health records from ~1800 family practices across England.
Study design
We undertook a self-controlled case series design, originally developed to examine vaccine safety 36 , 37 , to explore the association between BNT162b2, mRNA-1273 and ChAdOx1 vaccines and pre-specified outcomes. Separate analyses were conducted for each outcome. The analyses were conditional on each case, thus any fixed characteristic during the study period, such as sex and ethnicity, were inherently controlled for by design. Age was considered as a fixed variable because the study period was short.
Study period and population
The cohort included all children aged 5–17 years who had received at least one dose of BNT162b2, mRNA-1273 or ChAdOx1 vaccine or who had a positive SARS-CoV-2 test between 8th December 2020 and 7th August 2022. In each self-controlled case series analysis, we only included children who were admitted to hospital or died from the outcome during the study period and excluded children with a hospitalisation for the same outcome in the two years prior to 8th December 2020 and those who received other COVID-19 vaccine types. We also undertook an analysis in young adults aged 18–24 years as a comparison.
We a priori selected severe outcomes resulting in hospital admission or death which are monitored by national medical regulatory authorities, clinical trials, post-marketing surveillance, emerging scientific literature and neurological, cardiology, paediatric and immunology/vaccine expertise available. Our pre-defined outcomes were previously reported COVID-19 infection- and COVID-19 vaccination-related adverse events with strong evidence in young people: myocarditis 21 , 22 , MIS-C 38 and myositis 45 and those in adults: Guillain-Barre syndrome 43 , demyelinating disease 6 , ITP 39 and appendicitis 44 as well as adverse events reported following any vaccination during childhood and early young adulthood: epilepsy 40 , acute pancreatitis 41 , ADEM 42 and angioedema 46 . The selected outcomes are consistent with previous studies reporting COVID-19 vaccine safety in young people 58 . Anaphylaxis was also included as a positive control outcome because it can occur shortly after vaccination 46 . Outcomes were identified using relevant International Classification of Diseases codes ( https://www.qresearch.org/data/qcode-group-library/ ). For each outcome, we used the first event recorded during the study period and did not incorporate readmissions for the same outcome into the analysis.
Our main vaccine exposures were a first, second and third dose of BNT162b2, mRNA-1273 or ChAdOx1. To account for heterologous vaccination, each vaccine type and dose was considered separately. SARS-CoV-2 infection exposure was defined as the first positive SARS-CoV-2 test (assessed by RT-PCR) within the study period and was included as a separate variable, allowing safety outcomes that occurred following vaccination and infection to be allocated to both exposures in the model and mutually adjusted. We did not include reinfections in the analysis. We distinguished between infection occurring before the first vaccine dose, or after. We defined the exposure risk period as 1–42 days after each exposure under the assumption that the adverse events under consideration were unlikely to be related to the exposures if they occurred after 42 days. In the case where two vaccine doses were given within 42 days of each other, the risk period of the earlier dose was truncated on the date prior to the day that the next vaccine dose was received. People with a recent hospital admission may delay vaccination, therefore a pre-risk period of 1–28 days before each exposure was excluded from the baseline period to account for this potential bias 36 . Since people may have SARS-CoV-2 testing on hospital admission, we allocated day zero to a risk period of its own 36 . Whilst these admissions may have been caused by SARS-CoV-2 infection, reverse causality involved in their detection could lead to overestimation of the effect of infection on the outcome. All remaining observation time was included in the baseline period.
Statistical analysis
We analysed the data with the self-controlled case series method using a conditional Poisson regression model with an offset for the length of the exposure risk period. We fixed age as the age at date of first COVID-19 vaccination or date of first positive SARS-CoV-2 test recorded in study period for those who were unvaccinated. To allow for underlying seasonal effects, we split the study observation period into two-week periods and adjusted for these as a factor variable in the statistical models. We censored follow-up at the earliest of time of death, fourth dose or study end. We estimated the additional number of events per million persons exposed following vaccination or infection 59 and assessed significance at the 5% level.
In the primary analysis, we fitted the self-controlled case series model separately in children aged 5–11 years and adolescents aged 12–17 years and stratified by sex (male and female). We additionally fitted the model in 18–24-year-olds for comparison. As sensitivity analyses, we fitted the self-controlled case series model starting the observation period at the day of first, second and third vaccine dose and without censoring for deaths due to the outcome, to ascertain the robustness of our results when the outcome increases the probability of death. We fitted the model excluding those who received a third dose, as they might be the most clinically vulnerable individuals. We also fitted the model including children who were vaccinated but did not have a record of a SARS-CoV-2 infection during the study period, to assess the effect of the vaccine alone, to account for the possibility that an adverse event could have occurred within 42 days of both a vaccine dose and a SARS-CoV-2 infection. Finally, we fitted the model including only SARS-CoV-2 positive patients who had not received any vaccine to assess the effect of SARS-CoV-2 exposure alone. We additionally tested the effect of ethnicity (white or non-white) on the risk of each outcome by including an interaction term between ethnicity and vaccine/infection exposures.
Matched cohort analysis
We also conducted a post hoc matched cohort study using the QResearch database of primary care records, linked to hospital episode statistics, COVID-19 vaccination and SARS-CoV-2 infection data. The study population included all vaccinated children aged 5–17 years with GP records in the QResearch database, and matched unvaccinated children, irrespective of SARS-CoV-2 test status during the study period. We matched vaccinated children to children of the same age and sex who were unvaccinated at the time that the vaccinated child received their first dose (rounded to the nearest 7 days) at a ratio of 1:1. Unvaccinated children were sampled from the whole cohort and included children who were vaccinated later in the study period, and these children were censored on date of their first vaccination. The same matched pairs were included in the analysis of the second and third doses for those who received these doses, and where the matched unvaccinated child remained unvaccinated at the time of the second and third doses being received by the vaccinated child.
We estimated incidence rates and fitted conditional Poisson regression models to estimate IRRs of each outcome in the 1–42 days following a first, second or third dose of BNT162b2, mRNA-1273 or ChAdOX1 COVID-19 vaccine. We estimated unadjusted IRRs and IRRs adjusted for self-reported ethnicity (white, non-white, missing), quintile of deprivation (based on Townsend score) and presence of comorbidity (yes/no) that would result in inclusion in clinical risk group (diagnosis prior to vaccine being available).
We additionally conducted a matched cohort analysis in 18–24-year-olds for comparison.
Stata v17 was used for data analysis.
Reporting summary
Further information on research design is available in the Nature Portfolio Reporting Summary linked to this article.
Data availability
The data that support the findings of this study—NIMS Database of COVID-19, mortality (Office of National Statistics), hospital admissions (Hospital Episode Statistics), SARS-CoV-2 infection data (SGSS) and primary care (QResearch)—are not publicly available because they are based on deidentified national clinical records. Due to national and organizational data privacy regulations, individual-level data such as those used for this study cannot be shared openly. Access to the QResearch data is according to the information on the QResearch website ( www.qresearch.org ).
Code availability
A sample of the code used for a similar study has been deposited in the public git repository of the research group, available at https://github.com/qresearchcode/COVID-19-vaccine-safety . This sample code can be used to run a self-controlled case series analysis in STATA.
Health Policy Team. COVID-19 vaccination for children and young people. in Royal College of Paediatrics and Child Health , 2023 (2023).
UK Health Security Agency. Week 19 report (up to week 18 data): 11 May 2023. in Weekly National Influenza and COVID-19 surveillance report (2023).
UK Health Security Agency. COVID-19: the green book, chapter 14a. (2020).
Department of Health and Social Care. JCVI statement on the COVID-19 vaccination programme for 2023: 8 November 2022. 2023 (2023).
Hippisley-Cox, J. et al. Risk of thrombocytopenia and thromboembolism after covid-19 vaccination and SARS-CoV-2 positive testing: self-controlled case series study. BMJ 374 , n1931 (2021).
Article PubMed Google Scholar
Patone, M. et al. Neurological complications after first dose of COVID-19 vaccines and SARS-CoV-2 infection. Nat. Med. 27 , 12 (2021).
Google Scholar
Patone, M. et al. Risks of myocarditis, pericarditis, and cardiac arrhythmias associated with COVID-19 vaccination or SARS-CoV-2 infection. Nat. Med. 28 , 2 (2021).
Patone, M. et al. Risk of Myocarditis After Sequential Doses of COVID-19 Vaccine and SARS-CoV-2 Infection by Age and Sex. Circulation 146 , 743–754 (2022).
Article CAS PubMed PubMed Central Google Scholar
Frenck, R. W. et al. Safety, Immunogenicity, and Efficacy of the BNT162b2 Covid-19 Vaccine in Adolescents. N. Engl. J. Med. 385 , 239–250 (2021).
Article CAS PubMed Google Scholar
Ali, K. et al. Evaluation of mRNA-1273 SARS-CoV-2 Vaccine in Adolescents. N. Engl. J. Med. 385 , 2241–2251 (2021).
Barda, N. et al. Safety of the BNT162b2 mRNA Covid-19 Vaccine in a Nationwide Setting. N. Engl. J. Med. 385 , 1078–1090 (2021).
Sacco, C. et al. Effectiveness of BNT162b2 vaccine against SARS-CoV-2 infection and severe COVID-19 in children aged 5–11 years in Italy: a retrospective analysis of January–April, 2022. Lancet 400 , 97–103 (2022).
Nygaard, U. et al. Incidence and clinical phenotype of multisystem inflammatory syndrome in children after infection with the SARS-CoV-2 delta variant by vaccination status: a Danish nationwide prospective cohort study. Lancet Child Adolesc. Health 6 , 459–465 (2022).
Boehmer, T. K. Association Between COVID-19 and Myocarditis Using Hospital-Based Administrative Data - United States, March 2020-January 2021. MMWR Morb. Mortal. Wkly Rep. 70 , 1228–1232 (2021).
Molloy, E. J., Nakra, N., Gale, C., Dimitriades, V. R. & Lakshminrusimha, S. Multisystem inflammatory syndrome in children (MIS-C) and neonates (MIS-N) associated with COVID-19: optimizing definition and management. Pediatr. Res . 93 , 1499–1508 (2023).
Davis, H. E., McCorkell, L., Vogel, J. M. & Topol, E. J. Long COVID: major findings, mechanisms and recommendations. Nat. Rev. Microbiol. 21 , 133–146 (2023).
Lopez-Leon, S. et al. Long-COVID in children and adolescents: a systematic review and meta-analyses. Sci. Rep. 12 , 9950 (2022).
Article ADS CAS PubMed PubMed Central Google Scholar
Clayton, C., Clayton, R., Al-Azri, S., Mogeh, I. & Potter, M. Understanding parental hesitancy toward children’s COVID-19 vaccinations: The influence of government, media and interpersonal communication. Front. Commun. 7 , 1004139 (2023).
Ceannt, R., Vallieres, F., Burns, H., Murphy, J. & Hyland, P. Covid-19 vaccine hesitancy and resistance amongst parents of children under 18 years of age in Ireland. Vaccine 40 , 6196–6200 (2022).
Article PubMed PubMed Central Google Scholar
Bell, S., Clarke, R., Mounier-Jack, S., Walker, J. L. & Paterson, P. Parents’ and guardians’ views on the acceptability of a future COVID-19 vaccine: A multi-methods study in England. Vaccine 38 , 7789–7798 (2020).
World Health Organization. The Pfizer BioNTech (BNT162b2) COVID-19 vaccine: What you need to know. https://www.who.int/news-room/feature-stories/detail/who-can-take-the-pfizer-biontech-covid-19--vaccine-what-you-need-to-know (2022).
World Health Organization. The Moderna COVID-19 (mRNA-1273) vaccine: what you need to know. https://www.who.int/news-room/feature-stories/detail/the-moderna-covid-19-mrna-1273-vaccine-what-you-need-to-know (2022).
Mevorach, D. et al. Myocarditis after BNT162b2 mRNA Vaccine against Covid-19 in Israel. N. Engl. J. Med. 385 , 23 (2021).
Article Google Scholar
Li, M. et al. Myocarditis or Pericarditis Following the COVID-19 Vaccination in Adolescents: A Systematic Review. Vaccines 10 , 1316 (2022).
Goddard, K. et al. Incidence of Myocarditis/Pericarditis Following mRNA COVID-19 Vaccination Among Children and Younger Adults in the United States. Ann. Intern Med. 175 , 11 (2022).
Cheng, D. R. et al. Myocarditis and myopericarditis cases following COVID-19 mRNA vaccines administered to 12–17-year olds in Victoria, Australia. BMJ Paediatr. Open 6 , e001472 (2022).
Ling, R. R. et al. Myopericarditis following COVID-19 vaccination and non-COVID-19 vaccination: a systematic review and meta-analysis. Lancet Respir. Med. 10 , 679–688 (2022).
Witberg, G. et al. Myocarditis after BNT162b2 Vaccination in Israeli Adolescents. N. Engl. J. Med. 387 , 1816–1817 (2022).
Krug, A., Stevenson, J. & Høeg, T. B. BNT162b2 Vaccine-Associated Myo/Pericarditis in Adolescents: A Stratified Risk-Benefit Analysis. Eur. J. Clin. Investig. 52 , e13759 (2022).
Article CAS Google Scholar
Buchan, S. A. et al. Myocarditis or Pericarditis Events After BNT162b2 Vaccination in Individuals Aged 12 to 17 Years in Ontario, Canada. JAMA Pediatrics 177 , 410–418 (2023).
Husby, A. et al. Clinical outcomes of myocarditis after SARS-CoV-2 mRNA vaccination in four Nordic countries: population based cohort study. BMJ Med. 2 , e000373 (2023).
Hu, M. et al. Safety of the BNT162b2 COVID-19 Vaccine in Children Aged 5 to 17 Years. JAMA Pediatrics 177 , 7 (2023).
Watanabe, A. et al. Assessment of Efficacy and Safety of mRNA COVID-19 Vaccines in Children Aged 5 to 11 Years: A Systematic Review and Meta-analysis. JAMA Pediatr. 177 , 384–394 (2023).
Malden, D. E. et al. Reactions following Pfizer-BioNTech COVID-19 mRNA vaccination and related healthcare encounters among 7,077 children aged 5-11 years within an integrated healthcare system. Vaccine 41 , 315–322 (2023).
Hause, A. M. Safety Monitoring of Bivalent COVID-19 mRNA Vaccine Booster Doses Among Children Aged 5-11 Years - United States, October 12-January 1, 2023. MMWR Morb. Mortal. Wkly Rep. 72 , 39–43 (2023).
Petersen, I., Douglas, I. & Whitaker, H. Self controlled case series methods: an alternative to standard epidemiological study designs. BMJ 354 , i4515 (2016).
Farrington, C. P., Nash, J. & Miller, E. Case series analysis of adverse reactions to vaccines: a comparative evaluation. Am. J. Epidemiol. 143 , 1165–1173 (1996).
Wangu, Z., Swartz, H. & Doherty, M. Multisystem inflammatory syndrome in children (MIS-C) possibly secondary to COVID-19 mRNA vaccination. BMJ Case Rep. 15 , e247176 (2022).
Visser, C. et al. COVID-19 vaccination in patients with immune thrombocytopenia. Blood Adv. 6 , 1637–1644 (2022).
Lin, K. et al. Should patients with epilepsy be vaccinated against coronavirus disease 2019? A systematic review and meta-analysis. Epilepsy Behav. 134 , 108822 (2022).
Kalra, R. K., Jayadeep, S. & Ball, A. L. Acute Pancreatitis in an Adolescent Following COVID Vaccination. Clin. Pediatrics 61 , 236–240 (2022).
Nabizadeh, F., Noori, M., Rahmani, S. & Hosseini, H. Acute disseminated encephalomyelitis (ADEM) following COVID-19 vaccination: A systematic review. J. Clin. Neurosci. 111 , 57–70 (2023).
Kanou, S., Wardeh, L., Govindarajan, S. & Macnay, K. Guillain-Barre syndrome (GBS) associated with COVID-19 infection that resolved without treatment in a child. BMJ Case Rep. 15 , e245455 (2022).
Food and Drug Administration. Vaccines and Related Biological Products Advisory Committee Meeting. FDA Briefing Document: Pfizer-BioNTech COVID-19 Vaccine. (2020).
Syrmou, V. et al. COVID-19 vaccine-associated myositis: a comprehensive review of the literature driven by a case report. Immunologic Res. 71 , 4 (2023).
Kim, M. A. et al. COVID-19 Vaccine-associated Anaphylaxis and Allergic Reactions: Consensus Statements of the KAAACI Urticaria/Angioedema/Anaphylaxis Working Group. Allergy Asthma Immunol. Res 13 , 526–544 (2021).
National Institute for Health and Care Excellence. Epilepsies in children, young people and adults. (2022).
Wang, Z. et al. Safety and Tolerability of COVID-19 Vaccine in Children With Epilepsy: A Prospective, Multicenter Study. Pediatr. Neurol. 140 , 3–8 (2023).
Hood, V. et al. COVID-19 vaccine in patients with Dravet syndrome: Observations and real-world experiences. Epilepsia 63 , 1778–1786 (2022).
Garg, R. K. & Paliwal, V. K. Spectrum of neurological complications following COVID-19 vaccination. Neurological Sci. 43 , 3–40 (2022).
Ismail, I. I. & Salama, S. A systematic review of cases of CNS demyelination following COVID-19 vaccination. J. Neuroimmunol. 362 , 577765 (2022).
Averseng-Peaureaux, D. et al. Paediatric optic neuritis: factors leading to unfavourable outcome and relapses. Br. J. Ophthalmol. 102 , 808–813 (2018).
Department of Health and Social Care. Use of the AstraZeneca COVID-19 (AZD1222) vaccine: updated JCVI statement, 7 May 2021. https://www.gov.uk/government/publications/use-of-the-astrazeneca-covid-19-vaccine-jcvi-statement-7-may-2021 (2021).
Mitchell, J. & Yue, Q.-Y. Appendicitis as a possible safety signal for the COVID-19 vaccines. Vaccine: X 9 , 100122 (2021).
CAS PubMed Google Scholar
Kildegaard, H. et al. Risk of Appendicitis After mRNA COVID-19 Vaccination in a Danish Population. JAMA Intern. Med. 182 , 684–686 (2022).
Nafilyan, V. et al. Risk of death following COVID-19 vaccination or positive SARS-CoV-2 test in young people in England. Nat. Commun. 14 , 1541 (2023).
Cohen, J. M., Carter, M. J., Cheung, C. R. & Ladhani, S., Group, f.t.E.P.I.M.S.T.r.t.S.-C.-S. Lower Risk of Multisystem Inflammatory Syndrome in Children With the Delta and Omicron Variants of Severe Acute Respiratory Syndrome Coronavirus 2. Clin. Infect. Dis. 76 , e518–e521 (2022).
Center for Biologics Evaluation and Research. BEST Initiative. COVID-19 vaccine safety surveillance: protocol addendum for near real-time surveillance of COVID-19 vaccines in the pediatric population. (2022).
Wilson, K. & Hawken, S. Drug safety studies and measures of effect using the self-controlled case series design. Pharmacoepidemiol Drug Saf. 22 , 108–110 (2013).
Download references
Acknowledgements
This research is funded by the NIHR School for Primary Care Research, Grant Reference Number 622. The views expressed are those of the author(s) and not necessarily those of the NIHR or the Department of Health and Social Care. We acknowledge the contribution of EMIS practices who contribute to QResearch and EMIS Health and the Universities of Nottingham and Oxford for expertise in establishing, developing or supporting the QResearch database. This project involves data derived from anonymised patient-level information collected by the NHS. The SARS-CoV-2 test data were originally collated, maintained and quality assured by Public Health England (PHE) and transferred to NHS England during the study. Access to the data was therefore facilitated by NHS England. The Hospital Episode Statistics, Secondary Users Service (SUS-PLUS) datasets and civil registration data are used by permission from NHS England who retain the copyright in that data. NHS England and Public Health England bears no responsibility for the analysis or interpretation of the data. JHC is supported by an NIHR senior investigator award. NLM is supported by a British Heart Foundation Chair Award (CH/F/21/90010), Programme Grant (RG/20/10/34966) and Research Excellence Award (RE/18/5/34216). DPJH is supported by the Wellcome Trust (215621/Z/19/Z), Medical Research Foundation and UKDRI (principal funder UKRI Medical Research Council).
Author information
Authors and affiliations.
Nuffield Department of Primary Health Care Sciences, University of Oxford, Oxford, UK
Emma Copland, Martina Patone, Defne Saatci, Jennifer Hirst, Aziz Sheikh, Carol A. C. Coupland, Anthony Harnden & Julia Hippisley-Cox
Centre for Human Genetics, University of Oxford, Oxford, UK
Lahiru Handunnetthi
Department of Psychiatry, University of Oxford, Oxford, UK
UK Dementia Research Institute, Centre for Clinical Brain Sciences, University of Edinburgh, Edinburgh, UK
David P. J. Hunt
BHF/University Centre for Cardiovascular Science, University of Edinburgh, Edinburgh, UK
Nicholas L. Mills
Usher Institute, University of Edinburgh, Edinburgh, UK
Nicholas L. Mills & Aziz Sheikh
Institute of Immunology and Immunotherapy, University of Birmingham, Birmingham, UK
Centre for Academic Primary Care, School of Medicine, University of Nottingham, Nottingham, UK
Carol A. C. Coupland
Department of Mathematics and Statistics, University of Strathclyde, Glasgow, UK
Chris Robertson
You can also search for this author in PubMed Google Scholar
Contributions
M.P., E.C., J.H.C., C.A.C.C. led the study conceptualization, development of the research question and analysis plan and interpretation of the results. M.P. and J.H.C. obtained funding, obtained data approvals, undertook the data specification and curation. M.P. and E.C. designed the analysis, contributed to interpretation of the analysis, undertook data analysis and wrote the first draft of the paper. D.S., L.H., J.H., N.L.M., P.M., A.S., A.H., C.R. and D.P.J.H. contributed to the discussion on protocol development and provided critical feedback on drafts of the manuscript. All authors approved the protocol, contributed to the critical revision of the manuscript and approved the final version of the manuscript.
Corresponding author
Correspondence to Julia Hippisley-Cox .
Ethics declarations
Competing interests.
J.H.C. reports grants from National Institute for Health Research (NIHR) Biomedical Research Centre, Oxford, John Fell Oxford University Press Research Fund, Cancer Research UK and Oxford Wellcome Institutional Strategic Support Fund and other research councils, during the conduct of the study outside the scope of this work. J.H.C. is founder and was shareholder until 9 Aug 2023 of ClinRisk Ltd, which produces open and closed source software to implement clinical risk algorithms (outside this work) into clinical computer systems. J.H.C. is an unpaid director of QResearch, a not-for-profit organisation which is a partnership between the University of Oxford and EMIS Health who supply the QResearch database used for this work and is a consultant for Endeavour Predict Ltd outside this work. A.S. serves on a number of UK and Scottish Government COVID-19 advisory groups and was a member of AstraZeneca’s Thrombotic Thrombocytopenic Taskforce; all roles are unremunerated. A.H. is Deputy Chair of the Joint Committee on Vaccination and Immunisation. D.H. serves on the UK Government Commission on Human Medicines P.M. has received speaker and advisory board fees from Moderna and Astra Zeneca. All other authors declare no competing interests related to this paper.
Peer review
Peer review information.
Nature Communications thanks Masao Iwagami, Stéphane Le Vu and Christina Pagel for their contribution to the peer review of this work. A peer review file is available.
Additional information
Publisher’s note Springer Nature remains neutral with regard to jurisdictional claims in published maps and institutional affiliations.
Supplementary information
Supplementary information, peer review file, reporting summary, rights and permissions.
Open Access This article is licensed under a Creative Commons Attribution 4.0 International License, which permits use, sharing, adaptation, distribution and reproduction in any medium or format, as long as you give appropriate credit to the original author(s) and the source, provide a link to the Creative Commons licence, and indicate if changes were made. The images or other third party material in this article are included in the article’s Creative Commons licence, unless indicated otherwise in a credit line to the material. If material is not included in the article’s Creative Commons licence and your intended use is not permitted by statutory regulation or exceeds the permitted use, you will need to obtain permission directly from the copyright holder. To view a copy of this licence, visit http://creativecommons.org/licenses/by/4.0/ .
Reprints and permissions
About this article
Cite this article.
Copland, E., Patone, M., Saatci, D. et al. Safety outcomes following COVID-19 vaccination and infection in 5.1 million children in England. Nat Commun 15 , 3822 (2024). https://doi.org/10.1038/s41467-024-47745-z
Download citation
Received : 17 June 2023
Accepted : 11 April 2024
Published : 27 May 2024
DOI : https://doi.org/10.1038/s41467-024-47745-z
Share this article
Anyone you share the following link with will be able to read this content:
Sorry, a shareable link is not currently available for this article.
Provided by the Springer Nature SharedIt content-sharing initiative
By submitting a comment you agree to abide by our Terms and Community Guidelines . If you find something abusive or that does not comply with our terms or guidelines please flag it as inappropriate.
Quick links
- Explore articles by subject
- Guide to authors
- Editorial policies
Sign up for the Nature Briefing: Microbiology newsletter — what matters in microbiology research, free to your inbox weekly.

Open Access is an initiative that aims to make scientific research freely available to all. To date our community has made over 100 million downloads. It’s based on principles of collaboration, unobstructed discovery, and, most importantly, scientific progression. As PhD students, we found it difficult to access the research we needed, so we decided to create a new Open Access publisher that levels the playing field for scientists across the world. How? By making research easy to access, and puts the academic needs of the researchers before the business interests of publishers.
We are a community of more than 103,000 authors and editors from 3,291 institutions spanning 160 countries, including Nobel Prize winners and some of the world’s most-cited researchers. Publishing on IntechOpen allows authors to earn citations and find new collaborators, meaning more people see your work not only from your own field of study, but from other related fields too.
Brief introduction to this section that descibes Open Access especially from an IntechOpen perspective
Want to get in touch? Contact our London head office or media team here
Our team is growing all the time, so we’re always on the lookout for smart people who want to help us reshape the world of scientific publishing.
Home > Books > Epidemic Preparedness and Control
A Review of COVID-19 Vaccines, Immunogenicity, Safety, and Efficacy toward Addressing Vaccine Hesitancy, Inequity, and Future Epidemic Preparedness
Submitted: 30 August 2023 Reviewed: 08 October 2023 Published: 03 November 2023
DOI: 10.5772/intechopen.1003607
Cite this chapter
There are two ways to cite this chapter:
From the Edited Volume
Epidemic Preparedness and Control
Márcia Aparecida Sperança
To purchase hard copies of this book, please contact the representative in India: CBS Publishers & Distributors Pvt. Ltd. www.cbspd.com | [email protected]
Chapter metrics overview
53 Chapter Downloads
Impact of this chapter
Total Chapter Downloads on intechopen.com

Total Chapter Views on intechopen.com
This chapter provides an update on COVID-19 vaccines, emphasizing their immunogenicity, safety, efficacy, and potential impact on vaccine hesitancy, inequity, and future epidemic preparedness. Various vaccine types, such as mRNA-based, DNA-based, viral vector, inactivated, and protein subunit vaccines, are explored, evaluating their mechanisms and advantages in eliciting robust immune responses. Safety is thoroughly assessed using clinical trials and real-world data to address hesitancy concerns. Strategies for equitable distribution are discussed to achieve widespread coverage and overcome barriers. Lessons drawn from the pandemic serve as a roadmap for proactive measures aimed at bolstering epidemic preparedness, highlighting the critical role of global cooperation and equitable vaccine distribution in safeguarding public health worldwide.
- COVID-19 vaccine
- immunogenicity
- vaccine hesitancy
- vaccine inequity
- and future epidemic preparedness
Author Information
- Clinical Vaccine R&D Center, Chonnam National University Medical School, Hwasun-gun, Jeonnam, Republic of Korea
Vandara Loeurng
- Department of BioMedical Sciences, Chonnam National University Medical School, Hwasun-gun, Jeonnam, Republic of Korea
- Department of Veterinary Medicine, Meanchey National University, Banteay Meanchey province, Cambodia
*Address all correspondence to: [email protected]
1. Introduction
On January 30, 2020, the World Health Organization (WHO) officially declared the outbreak of novel coronavirus disease 2019 (COVID-19) as a global pandemic. This declaration was made in response to the highly transmittable and pathogenic nature of the severe acute respiratory syndrome coronavirus 2 (SARS-CoV-2), an RNA virus [ 1 ]. Since then, COVID-19 has caused more than 6 million deaths worldwide [ 2 ], resulting in significant economic losses globally [ 3 ]. Despite efforts to control the pandemic, it is still ongoing as China continues to report high numbers of cases. Concerns are rising that the “Zero COVID” policies implemented in China may not effectively contain the spread of COVID-19 [ 4 ]. Furthermore, scientific evidence suggests that climate changes, such as global warming, rapid population growth, urban expansion, and deforestation, are bringing human habitation closer to livestock and wildlife, potentially serving as hosts for future pandemic pathogens [ 5 ].
Since the onset of the disease, vaccines and vaccination strategies have continuously evolved, gaining particular attention in the wake of the COVID-19 outbreak. Notable advancements have been made in the development of novel vaccine technologies, resulting in the creation of effective vaccines against various diseases [ 6 , 7 ]. Specifically addressing the ongoing global pandemic caused by the SARS-CoV-2 virus, COVID-19 vaccines have been swiftly developed and distributed, serving as a crucial tool in the fight against the pandemic [ 8 , 9 , 10 ]. Clinical trials have demonstrated that these vaccines effectively prevent severe illness, hospitalization, and death. Furthermore, they possess the potential to slow down virus transmission and reduce the emergence of new variants ( Tables 1 – 3 ). The widespread administration of vaccines is vital in safeguarding vulnerable populations, including the elderly and individuals with underlying health conditions [ 10 ]. Compared to natural infection, vaccination plays a prominent role in saving humanity from various COVID-19 variants, including the recent surge of the Omicron variant and aiding in the recovery from the global economic crisis ( Tables 1 – 3 ).
A comprehensive review of the immunogenic characteristics exhibited by approved COVID-19 vaccines.
A comprehensive review of the safety profiles demonstrated by approved COVID-19 vaccines.
A comprehensive review of the effectiveness of approved COVID-19 vaccines in preventing symptomatic COVID-19.
While COVID-19 vaccines offer numerous benefits, they also have limitations regarding their immunogenicity, safety, and effectiveness. Vaccine hesitancy is a concern, as individuals may refuse vaccination due to apprehensions about the immunogenicity, safety, and efficacy of the vaccines [ 61 , 62 , 63 , 64 , 65 ]. The efficacy rates of COVID-19 vaccines vary among different types, and emerging virus variants can impact their effectiveness. For instance, mRNA vaccines have demonstrated higher efficacy rates compared to viral vector and inactivated virus vaccines ( Table 3 ). Extensive research and monitoring have been conducted to ensure the safety of COVID-19 vaccines with most reported adverse events being mild and temporary ( Table 2 ). However, rare cases of severe adverse events, such as blood clots, have been associated with some vaccines, leading to temporary suspensions in vaccine distribution and increased surveillance [ 65 , 66 ].
Overall, COVID-19 vaccines induce immunogenicity by stimulating the production of neutralizing antibodies and cellular immune responses ( Table 1 ). While the inactivated COVID-19 vaccines may exhibit slightly lower immunogenicity compared to certain other formed vaccines, they still prove effective in reducing the severity of COVID-19 and providing protection against severe outcomes ( Table 3 ). Despite the development and approval of multiple vaccines, there remains a significant challenge of inequitable access to vaccines, particularly in low- and middle-income countries (LMICs) [ 67 ]. Additionally, logistical obstacles such as cold chain storage and vaccine distribution have hindered efficient delivery in certain regions [ 68 ].
At this juncture, it is crucial for us to reflect upon the valuable lessons learned during the COVID-19 pandemic spanning the past 3 years. It is imperative that we contemplate how to prepare better and effectively respond to future challenges. In addition to implementing measures like social distancing, it is essential to have a comprehensive understanding of vaccine technologies and the immunogenicity, safety, and efficacy of vaccines. The objective of this chapter is threefold: (1) To examine and reflect upon recent advancements in vaccine technologies, focusing on the immunogenicity, safety, and efficacy of approved COVID-19 vaccines; (2) To provide critical insights aimed at addressing gaps in vaccine inequity, ensuring that access to vaccines is fair and equitable for all; (3) To provide critical insights and recommendations for preparedness and control measures in response to potential future pandemics. By addressing these aspects, we aim to enhance our preparedness and response capabilities, ultimately striving for more effective control and management of future health crises.
2. Overview of SARS-CoV-2 and its biology
The severe acute respiratory syndrome coronavirus 2 (SARS-CoV-2), belonging to the coronavirus family, has led to outbreaks of various diseases. This family of viruses includes common viruses responsible for ailments ranging from common colds to more severe conditions like severe acute respiratory syndrome (SARS), which caused an outbreak in 2003 [ 69 ], as well as middle east respiratory syndrome (MERS), which caused an outbreak in 2012 [ 70 ]. The most recent outbreak occurred in 2019 with the emergence of SARS-CoV-2 [ 1 ], resulting in significant global health impacts [ 2 ] and economic consequences [ 3 ].
SARS-CoV-2 is an enveloped virus characterized by its positive-sense single-stranded RNA genome. The genome of SARS-CoV-2 contains instructions for the production of four primary structural proteins: spike glycoprotein (S), envelope protein (E), membrane protein (M), and nucleoprotein (N) [ 71 ]. These four structural proteins come together to encapsulate the viral genetic material (RNA), forming a complete virus capable of replication, transmission, and disease onset ( Figures 1 and 2 ).

The structural representation of SARS-CoV-2. This visual depiction was obtained from biorender ( https://www.biorender.com ).

The portrayal of SARS-CoV-2’s entry into host cells via the ACE2 receptor. This visual representation illustrates the simulated steps and mechanisms involved in the recognition and entry of host cells through ACE2-mediated pathways by the prefusion spike glycosylation (S1). This visual depiction was obtained from biorender ( https://www.biorender.com ).
The virus exhibits a spherical shape and displays spike-like projections on its surface, which gives it a crown-like appearance ( Figure 1 ). These projections are composed of the glycoprotein spike (S protein), consisting of two subunits, S1 and S2, and play a crucial role in receptor recognition and fusion with the host cell membrane [ 72 , 73 ]. Specifically, SARS-CoV-2 attaches to host cells by utilizing its spike protein to bind to the angiotensin-converting enzyme 2 (ACE2) receptor located on the surface of human cells. Upon entering the host cell, the viral RNA genome is released and serves as a template for the synthesis of viral proteins. The viral RNA is then replicated, packaged into new virus particles, and subsequently released from the host cell to infect other cells, leading to the development of novel coronavirus disease 2019 (COVID-19) ( Figure 2 ). In the absence of prevention or treatment, SARS-CoV-2 primarily targets the respiratory tract and can cause symptoms ranging from mild to severe respiratory illness [ 74 ]. It is worth noting that the virus can also infect other organs, including the skin, kidneys, endocrine organs, and eyes [ 75 ]. The severity of COVID-19 disease can vary widely and is influenced by factors such as age, underlying health conditions, and viral load [ 76 ].
Mounting an effective immune response to SARS-CoV-2 infection plays a crucial role in controlling the virus and mitigating the development of severe disease [ 77 , 78 , 79 , 80 ]. Vaccination, in particular, elicits the production of antibodies targeting the S proteins, which have been shown to generate neutralizing antibodies capable of impeding the virus from infecting host cells. Additionally, T cells are activated, enabling them to identify and eliminate infected cells ( Table 1 ).
Like all viruses, including SARS-CoV-2, there are numerous mutations that can give rise to new variants with distinct characteristics. These characteristics can include rapid transmission, increased disease severity, or resistance to vaccines and treatments [ 81 , 82 ]. These altered versions of the virus are commonly referred to as “variants”. Some variants have shown higher transmissibility and may be linked to an elevated risk of severe illness [ 83 , 84 , 85 ]. The emergence of these variants highlights the critical need for ongoing surveillance and research to comprehend the evolution and dissemination of the virus [ 81 ]. The Omicron variant of SARS-CoV-2, initially identified in South Africa, was reported to the WHO on November 24, 2021, as a novel variant [ 84 ].
3. An update of COVID-19 vaccines
Recent advancements in vaccine technology have led to the development of various vaccines available in the market ( Figure 3 ) [ 6 , 7 ]. These vaccines consist of several crucial components. First, they contain an antigen, which can be in the form of a live-attenuated or killed virus, or a specific protein derived from the virus. The presence of the antigen enables our bodies to recognize and combat the disease if we come into contact with it in the future. Second, vaccines include an adjuvant, which assists in enhancing our immune response and improving the effectiveness of the vaccine. Additionally, vaccines are formulated with preservatives to maintain their efficacy over time, and stabilizers to prevent mechanical failures during storage and transportation. It is important to note that all the ingredients used in vaccines, as well as the vaccines themselves, undergo thorough testing and monitoring to ensure their safety and efficacy [ 7 ].

An overview of the approved COVID-19 vaccines. Fifty approved COVID-19 vaccines were obtained from the COVID-19 vaccine tracker ( https://covid19.trackvaccines.org/ ), with data updated until December 2, 2022, and accessed on June 19, 2023. The vaccine platforms are classified into four main categories, based on their vaccine technologies. Each vaccine platform as a representative example was illustrated for its immunogenic mechanisms. (A) inactivated vaccines (11 vaccines), (B) protein-based vaccines (19 protein vaccines & 1 VLP vaccine), (C) viral vector vaccines (9 vaccines), and (D) nucleic acid vaccines (9 mRNA vaccines & 1 DNA vaccine). This visual depiction was produced using a biorender ( https://www.biorender.com ).
Up until December 2, 2022, a significant milestone has been reached with the approval of 50 vaccines by at least one country. Additionally, the WHO has granted Emergency Use Listing (EUL) to 11 vaccines, benefiting a total of 201 countries [ 86 ]. This remarkable progress showcases the global efforts in combating the COVID-19 pandemic. The extensive pursuit of vaccine development is evident in the reported 821 vaccine trials. Currently, there are 242 vaccine candidates undergoing clinical trials at various stages of progress. Among them, 66 vaccines are in Phase 1 trials, indicating their initial safety and dosage assessments. Phase 2 trials involve 72 vaccines, evaluating their effectiveness in larger populations. Furthermore, 92 vaccines are in Phase 3 trials, which involve large-scale testing to determine their overall efficacy. Notably, 50 vaccines have already received authorization, indicating their successful completion of clinical trials and regulatory approval. These crucial vaccine trials are being conducted in 80 countries worldwide, highlighting the widespread collaboration and dedication in the pursuit of effective COVID-19 vaccines ( Figure 4 ).

An overview of the present scenario concerning COVID-19 vaccines available in the market. The figure was adopted from the COVID-19 vaccine tracker ( https://covid19.trackvaccines.org/ ), with data updated until December 2, 2022, and accessed on June 19, 2023. This visual depiction was produced using a biorender ( https://www.biorender.com ).
Furthermore, the authorized COVID-19 vaccines encompass a diverse array of types, showcasing advancements in medical technology. These include mRNA, DNA, viral vectors, inactivated or killed viruses, and protein-based subunit vaccines ( Figure 3 ). mRNA vaccines, such as the notable ones developed by Pfizer-BioNTech and Moderna, harness the power of genetic material to initiate an immune response within the body. Similarly, DNA vaccines like Zydus Cadila utilize genetic material to stimulate the immune system’s defenses. Viral vector vaccines, such as the well-known ones manufactured by AstraZeneca and Johnson & Johnson, take advantage of a modified virus as a delivery system to transport genetic material and activate the immune response. By utilizing this approach, these vaccines can effectively trigger the body’s defense mechanisms against the virus. Inactivated or killed virus vaccines, exemplified by the commendable efforts of Sinovac or Sinopharm, employ viruses that have been rendered inactive or killed to provoke an immune response. Through this method, the immune system becomes primed to recognize and combat the virus should an actual infection occur. Protein subunit vaccines, including the notable ones produced by Novavax or Medicago, employ specific proteins derived from the virus itself. By introducing these viral proteins into the body, an immune response is triggered, enabling the immune system to develop defenses against the virus. Collectively, these various COVID-19 vaccine platforms have demonstrated their efficacy in generating neutralizing antibodies and stimulating cell-mediated responses, as depicted in Figure 3 and referenced in Tables 1 – 3 .
4. The immunogenicity of COVID-19 vaccines
Vaccine immunogenicity refers to the capacity of a vaccine to stimulate an immune response within the body. The primary objective of vaccination is to activate the immune system, prompting it to recognize and counter specific components of a pathogen, such as a virus, while avoiding the onset of the actual disease [ 87 ]. Upon administration of the vaccine, the immune response is triggered, leading to the activation of various immune cells, including B cells. The immune system identifies the foreign components within the vaccine as antigens and initiates an immune response against them. B cells are responsible for producing antibodies that can neutralize the pathogen or mark it for elimination by other immune cells. T cells, comprising helper T cells and killer T cells, play a crucial role in coordinating and executing the immune response [ 88 ].
Vaccine immunogenicity is evaluated through clinical trials, wherein researchers measure the production of specific antibodies, such as neutralizing antibodies, in the blood of vaccinated individuals ( Table 1 ). These antibodies are designed to recognize and bind to the specific components of the targeted pathogen that the vaccine aims to address. The presence of these antibodies indicates that the vaccine has successfully stimulated an immune response. The level and quality of the immune response induced by a vaccine can vary based on several factors, including the vaccine type, the antigens it contains, the dosage, and the vaccination schedule [ 89 ]. High immunogenicity is desirable as it suggests that the vaccine effectively triggers a robust immune response and provides protection against the specific pathogen being targeted [ 90 ].
Table 1 provides a comprehensive analysis of the immunogenicity of approved COVID-19 vaccines. Overall, these vaccines have been shown to elicit strong immune responses, including the generation of neutralizing antibodies and virus-specific T cells which are effective in reducing the risk of severe disease, hospitalization, and death due to COVID-19.
5. The safety of COVID-19 vaccines
Vaccine safety encompasses the comprehensive evaluation of potential risks and adverse effects associated with the administration of a vaccine. This evaluation involves rigorous testing throughout clinical trials and ongoing monitoring following its approval and widespread use [ 2 ]. Before entering clinical trials, vaccines typically undergo preclinical testing, including laboratory studies and animal testing to assess their safety and effectiveness. This initial evaluation helps identify any potential safety concerns and informs the decision to proceed with human trials. Subsequently, vaccines progress through various phases of clinical trials involving human participants. These trials thoroughly examine the vaccine’s safety, immunogenicity, and efficacy. Throughout these trials, vaccine safety is closely monitored, and participants are carefully observed for any adverse reactions or side effects [ 91 , 92 , 93 ].
Table 2 provides a comprehensive analysis of the safety profile of approved COVID-19 vaccines, which has been extensively studied in both clinical trials and real-world settings. Most of the reported adverse events have been mild and temporary, such as localized pain at the injection site and low-grade fever [ 92 ]. Although rare, there have been reports of more severe adverse events, including anaphylaxis [ 93 ]. However, it is important to note that the occurrence of such serious adverse events is very low [ 93 ]. Overall, the risk of experiencing severe adverse events from the vaccines is significantly lower compared to the risk of severe illness resulting from COVID-19 ( Table 2 ).
6. The efficacy of COVID-19 vaccines
Vaccine efficacy is a measurement of the effectiveness of a vaccine in disease or infection prevention, determined through controlled clinical trials under controlled conditions. This measure quantifies the reduction in disease incidence among vaccinated individuals in comparison to their unvaccinated counterparts, offering insights into the vaccine’s capacity to protect against a specific pathogen. Clinical trials randomly assign participants to receive either the vaccine or a placebo, monitoring the occurrence of the targeted disease or infection in both groups [ 94 ]. By comparing the incidence rates, researchers can calculate the vaccine’s efficacy, expressed as a percentage that represents the reduced risk of developing the disease among vaccinated individuals.
Table 3 provides an efficacy review analysis of approved COVID-19 vaccines in preventing symptomatic COVID-19. Clinical trials and real-world data have demonstrated that all COVID-19 vaccines effectively protect against severe illness, hospitalization, and mortality. When it comes to preventing symptomatic COVID-19, mRNA vaccines have demonstrated notably higher efficacy rates compared to other types of vaccines ( Table 3 ) [ 94 ].
7. Opinions on immunogenicity, safety, and efficacy toward filling gaps of vaccine hesitancy
The evaluation of COVID-19 vaccines takes into account important factors such as immunogenicity, safety, and efficacy. A thorough review of these factors for authorized COVID-19 vaccines reveals that various vaccine platforms, including inactivated vaccines, viral vector vaccines, mRNA vaccines, DNA vaccines, and protein-based vaccines, demonstrate strong immunogenicity by eliciting neutralizing antibodies and cellular immune responses ( Table 1 ). Moreover, studies have demonstrated that these vaccines are both safe and effective in preventing symptomatic cases of COVID-19 ( Tables 2 and 3 ).
In terms of immunogenicity, both the recommended two-dose regimen and a single dose of the Johnson & Johnson vaccine have been shown to elicit strong immune responses in clinical trials and real-world data ( Table 1 ). These responses include the production of neutralizing antibodies and the activation of T-cell responses, which are important for combating the SARS-CoV-2 virus. While the level of immunogenicity may vary among different vaccine platforms, overall, vaccines have proven effective in reducing the severity of COVID-19 symptoms and preventing hospitalization and mortality ( Table 3 ).
When it comes to safety, robust monitoring systems have been implemented to continuously evaluate the side effects of vaccines [ 53 , 92 , 93 ]. Most adverse events have been mild and temporary, including symptoms like pain at the injection site, fatigue, or low-grade fever ( Table 2 ). These mild to moderate side effects have been observed across various vaccine platforms and generally resolve on their own within a few days. Serious adverse events are exceedingly rare [ 53 ], and the benefits of vaccination in preventing severe COVID-19 far outweigh the potential risks. However, ensuring vaccine safety remains a top priority, and ongoing surveillance plays a critical role in promptly addressing any emerging safety concerns [ 53 ].
In terms of efficacy, COVID-19 vaccines have shown remarkable effectiveness in preventing both the incidence and severity of COVID-19 [ 94 ] ( Table 3 ). Vaccination has proven to be highly successful in reducing hospitalizations and fatalities, even in the presence of emerging variants of concern. However, breakthrough infections can still occur, particularly with new variants like “Omicron,” underscoring the need for ongoing surveillance, booster doses, and the development of updated vaccines to address viral mutations. Continued vigilance and adaptation are crucial to ensure the continued effectiveness of COVID-19 vaccination efforts according to a report [ 95 ].
Furthermore, the prime-boost vaccination strategy has demonstrated its potency, safety, and effectiveness in combating COVID-19 ( Tables 2 and 3 ). In addition, studies have shown that heterologous prime-boost vaccination using combinations of an inactivated vaccine, AstraZeneca, Pfizer, and Moderna vaccines can be even more potent compared to homologous prime-boost vaccination using any single vaccine alone [ 96 , 97 ]. The implementation of homologous or heterologous prime-boost vaccination strategies has been observed. In Cambodia, for instance, a homologous or heterologous prime-boost vaccination approach has been implemented, with inactivated vaccines used as the priming vaccine and viral vector or mRNA vaccines as the boosting vaccine. Encouragingly, no adverse events have been reported, and the vaccinated population in Cambodia has experienced a significant reduction in mortality [ 98 ]. These findings further emphasize the overall convenience and benefits of COVID-19 vaccination strategies.
8. Opinions on immunogenicity, safety, and efficacy toward filling gaps of vaccine inequity
In the global context, the impact of COVID-19 vaccines is influenced by the administration of numerous approved vaccines worldwide, as illustrated in Figures 4 and 3 . A staggering 13.47 billion vaccine doses have been administered globally, with an average of 70.3% of the world population having received at least one dose and 93,683 doses being administered daily [ 8 ]. However, it is concerning that only 32.2% of individuals in low and middle-income countries (LMICs) have received at least one vaccine dose, primarily utilizing vaccines with lower prominence [ 8 ]. These statistics highlight the existence of a “vaccine inequity” gap between LMICs and developed nations.
The “vaccine inequity” observed among LMICs can be attributed to several crucial factors. These include the inability of these countries to manufacture their own vaccines, limited budgets to purchase advanced vaccines like Pfizer or Moderna [ 7 ], and concerns regarding the immunogenicity, safety, and efficacy of lower-profile vaccines, which may lead to vaccine hesitancy [ 61 , 62 , 63 , 64 , 65 ]. In light of these challenges, it is important to emphasize that the available vaccines, as outlined in Tables 2 and 3 , have demonstrated overall safety and effectiveness, particularly in reducing hospitalizations and deaths. Therefore, individuals are encouraged to receive any vaccines that are accessible and affordable to help mitigate the impact of COVID-19.
COVAX and vaccine diplomacy are crucial strategies that have significant impacts on achieving equitable distribution of vaccines. By pooling resources and coordinating with vaccine manufacturers, COVAX aims to provide vaccines to LMICs that may otherwise struggle to secure sufficient doses. This initiative helps bridge the gap between nations with ample resources and those facing financial and logistical challenges. In addition, to effectively address vaccine inequity, it is also crucial to establish comprehensive vaccine surveillance systems that monitor the immunogenicity, safety profile, and efficacy of vaccines in regional countries. Taking inspiration from the UK report released by the UK Health Security Agency on October 21, 2021 [ 95 ], implementing such surveillance systems can provide valuable insights and help ensure that vaccines are being administered effectively and safely in different regions. This approach would significantly enhance trust in vaccine uptake within these countries and serve as essential documentation for future vaccine research and development. In Cambodia, for instance, a range of vaccines, including inactivated vaccines (Sinovax & Sinopharm), mRNA vaccines (Pfizer & Moderna), and viral vector vaccines (Astrazeneca & Janssen), are being used [ 7 , 98 ]. It would be highly beneficial if Cambodia also conducts evaluation reports similar to the UK case [ 95 ]. These reports will be invaluable for future vaccine research and development in Cambodia, providing insights into which vaccine-based platforms offer long-lasting protective and therapeutic efficacy for the country’s geographic and regional populations. Additionally, the reports will bolster efforts to enhance vaccine production capabilities, enabling better preparedness for future pandemics and ensuring the availability, safety, effectiveness, and affordability of vaccines in Cambodia.
In the global context, furthermore, as the UK holds the presidency of the G7, it should also consider conducting a comprehensive study in line with the research conducted by Edward J Mills and Gilmar Reis [ 99 ]. This study would involve head-to-head clinical trials comparing vaccines such as Pfizer, Moderna, Astrazeneca, Sputnik V, and Sinopharm, among others. The aim of this study would be to accumulate data and establish trust in the immunogenicity, safety, and efficacy of these vaccines. Including lower-profile vaccines in the study would have a significant impact on many LMICs, maximizing vaccine uptake in those regions. Importantly, such a study would contribute to shaping a better global solution regarding the prioritization of vaccine production, determining who should be offered the vaccine and establishing guidelines for booster shots, both in LMICs and developed nations. Moreover, it would enhance preparedness and control measures for future pandemic threats, ensuring a more effective response.
In cases where certain lower-profile vaccines are not approved for use in the UK, conducting a head-to-head study becomes limited. However, it is crucial to consider alternative approaches such as a collective report from G7 and G20 countries, along with data from LMICs. This would provide a more comprehensive analysis of vaccines’ immunogenicity, safety, and efficacy. Additionally, the inclusion of vaccines like the DNA vaccine (Zydus Candila) from India, VLP (Medicago) from Canada, and Novavax (USA) ( Figure 3 ) should be considered. Another valuable approach would be to develop a methodology that combines data from separate studies to estimate the immunogenicity, safety, and efficacy of different vaccines. This approach would enhance the credibility and reliability of the findings.
9. Opinions on immunogenicity, safety and efficacy toward future epidemic preparedness and control
This report presents an update on the current landscape of COVID-19 vaccines, emphasizing their immunogenicity, safety, and efficacy in the context of filling gaps of vaccine hesitancy, vaccine inequity, and future epidemic preparedness and control. The development and widespread administration of vaccines have played a crucial role in mitigating the impact of the ongoing COVID-19 pandemic [ 9 , 10 ]. With numerous vaccines receiving emergency use authorization, the knowledge gained from their development and deployment can significantly contribute to future epidemic preparedness and control efforts [ 100 ]. The remarkable speed at which vaccines were developed and distributed globally highlights the potential for expediting vaccine development in response to emerging infectious diseases. Through collaborative endeavors involving governments, researchers, and pharmaceutical companies, we can further enhance vaccine production, distribution, and ensure equitable access, thus strengthening global preparedness against future epidemics [ 7 ].
Furthermore, it is crucial for LMICs to prioritize the enhancement of laboratory capacities to enable prompt and accurate diagnosis of infectious diseases [ 101 ]. This can be achieved by investing in infrastructure, equipment, and training for laboratory personnel. By strengthening laboratory capabilities, LMICs can effectively contribute to epidemic prevention, detection, and control. It is also important for LMICs to allocate resources to support research and development initiatives focused on combating epidemics [ 7 ]. This entails providing financial support to local research institutions, fostering collaborations with international partners, and conducting studies on the local epidemiology of infectious diseases, actively practice in non-pandemic times through one health concept [ 101 ]. To ensure reliable and standardized testing, it is imperative to establish well-equipped laboratories, provide comprehensive training to laboratory staff, and implement robust quality control measures [ 7 ]. Additionally, addressing vaccine hesitancy is paramount. This can be accomplished through transparent communication, accessible education, and community engagement. Public health campaigns should prioritize disseminating accurate information, dispel myths and misinformation, and address concerns in order to enhance vaccine acceptance among the population.
10. Conclusion
In summary, the utilization of COVID-19 vaccines has demonstrated their effectiveness in mitigating the impact of the pandemic, showcasing favorable immunogenicity, safety, and efficacy against the virus. These valuable insights gained from developing and deploying vaccines can be invaluable for future epidemic preparedness and control. To ensure successful vaccination strategies in future outbreaks and epidemics, it is imperative to prioritize factors such as maintaining public trust, promoting equitable access to vaccines, and continuing research and development efforts. By applying these lessons, we can strengthen our readiness to effectively address and control potential future epidemics.
Conflict of interest
The authors declare no competing financial interests or personal relationships that could have appeared to influence the work reported in this chapter.
- 1. Hu B, Guo H, Zhou P, Shi ZL. Characteristics of SARS-CoV-2 and COVID-19. Nature Reviews. Microbiology. 2021; 19 (3):141-154. DOI: 10.1038/s41579-020-00459-7
- 2. World Health Organization (WHO). WHO Coronavirus (COVID-19) Dashboard. 2023. Available from: https://covid19.who.int/
- 3. World Economic Forum (WEF). World Vs Virus Podcast: An Economist Explains What COVID-19 has done to the Global Economy. 2020. Available from: https://www.weforum.org/agenda/2020/09/an-economist-explains-what-covid-19-has-done-to-the-global-economy/
- 4. United Nations (UN). WHO Continues to Urge China to Share More Data Amid COVID-19 Surge. 2023. Available from: https://news.un.org/en/story/2023/01/1132167
- 5. Morens DM, Fauci AS. Emerging pandemic diseases: How we got to COVID-19. Cell. 2020; 183 (3):837. DOI: 10.1016/j.cell.2020.10.022
- 6. Mascola JR, Fauci AS. Novel vaccine technologies for the 21st century. Nature Reviews. Immunology. 2020; 20 (2):87-88. DOI: 10.1038/s41577-019-0243-3
- 7. MISTI. Readiness for Future Vaccine Development and Production in Cambodia. Phnom Penh Capital City, Cambodia: Ministry of Industry, Science, Technology and Innovation; 2022 e-Book Edition: 2022, ISBN: 9789924-9556-7-2. Available from: https://misti.gov.kh/public/file/202206071654577440.pdf
- 8. Our World In Data. Coronavirus (COVID-19) Vaccinations. 2023. Available from: https://ourworldindata.org/covid-vaccinations
- 9. Mathieu E, Ritchie H, Ortiz-Ospina E, Roser M, Hasell J, Appel C, et al. Author correction: A global database of COVID-19 vaccinations. Nature Human Behaviour. 2021; 5 (7):956-959. DOI: 10.1038/s41562-021-01160-2
- 10. OECD Policy Responses to Coronavirus (COVID-19). Access to COVID-19 Vaccines: Global Approaches in a Global Crisis. 2021. Available from: https://www.oecd.org/coronavirus/policy-responses/access-to-covid-19-vaccines-global-approaches-in-a-global-crisis-c6a18370/
- 11. Zhang Y, Zeng G, Pan H, Li C, Hu Y, Chu K, et al. Safety, tolerability, and immunogenicity of an inactivated SARS-CoV-2 vaccine in healthy adults aged 18-59 years: A randomised, double-blind, placebo-controlled, phase 1/2 clinical trial. The Lancet Infectious Diseases. 2021; 21 (2):181-192. DOI: 10.1016/S1473-3099(20)30843-4
- 12. Han B, Song Y, Li C, Yang W, Ma Q, Jiang Z, et al. Safety, tolerability, and immunogenicity of an inactivated SARS-CoV-2 vaccine (CoronaVac) in healthy children and adolescents: A double-blind, randomised, controlled, phase 1/2 clinical trial. The Lancet Infectious Diseases. 2021; 21 (12):1645-1653. DOI: 10.1016/S1473-3099(21)00319-4
- 13. Wang Y, Ma Y, Xu Y, Liu J, Li X, Chen Y, et al. Resistance of SARS-CoV-2 omicron variant to convalescent and CoronaVac vaccine plasma. Emerging Microbes & Infections. 2022; 11 (1):424-427. DOI: 10.1080/22221751.2022.2027219
- 14. Schultz BM, Melo-González F, Duarte LF, Galvez NMS, Pacheco GA, Soto JA, et al. A booster dose of coronavac increases neutralizing antibodies and T cells that recognize delta and omicron variants of concern. mBio. 30 Aug 2022; 13 (4):e0142322. DOI: 10.1128/mbio.01423-22. Epub 2022 Aug 10. PMID: 35946814; PMCID: PMC9426482
- 15. Ella R, Reddy S, Jogdand H, Sarangi V, Ganneru B, Prasad S, et al. Safety and immunogenicity of an inactivated SARS-CoV-2 vaccine, BBV152: Interim results from a double-blind, randomised, multicentre, phase 2 trial, and 3-month follow-up of a double-blind, randomised phase 1 trial. The Lancet Infectious Diseases. 2021; 21 (7):950-961. DOI: 10.1016/S1473-3099(21)00070-0
- 16. Kumar NP, Banurekha VV, Kumar CPG, Nancy A, Padmapriyadarsini C, Shankar S, et al. Inactivated COVID-19 vaccines: Durability of Covaxin/BBV152 induced immunity against variants of concern. Journal of Travel Medicine. 17 Sep 2022; 29 (6):taac088. DOI: 10.1093/jtm/taac088. PMID: 35900009; PMCID: PMC9384591
- 17. WHO. The Sinopharm COVID-19 Vaccine: What you Need to Know. 2022. Available from: https://www.who.int/news-room/feature-stories/detail/the-sinopharm-covid-19-vaccine-what-you-need-to-know
- 18. Skelly DT, Harding AC, Gilbert-Jaramillo J, Knight ML, Longet S, Brown A, et al. Two doses of SARS-CoV-2 vaccination induce robust immune responses to emerging SARS-CoV-2 variants of concern. Nature Communications. 2021; 12 (1):5061. DOI: 10.1038/s41467-021-25167-5
- 19. REUTERS. Sinopharm’s COVID Booster Reverses Antibody Decline, Enhances Cell-Based Responses - Study. 2021. Available from: https://www.reuters.com/world/china/sinopharms-covid-booster-reverses-antibody-decline-enhances-cell-based-responses-2021-09-17/
- 20. Kanokudom S, Chansaenroj J, Suntronwong N, Assawakosri S, Yorsaeng R, Nilyanimit P, et al. Safety and immunogenicity of a third dose of COVID-19 protein subunit vaccine (Covovax(TM)) after homologous and heterologous two-dose regimens. International Journal of Infectious Diseases. 2023; 126 :64-72. DOI: 10.1016/j.ijid.2022.11.022
- 21. Toledo-Romani ME, Garcia-Carmenate M, Valenzuela-Silva C, Baldoquin-Rodriguez W, Martinez-Perez M, Rodriguez-Gonzalez M, et al. Safety and efficacy of the two doses conjugated protein-based SOBERANA-02 COVID-19 vaccine and of a heterologous three-dose combination with SOBERANA-plus: A double-blind, randomised, placebo-controlled phase 3 clinical trial. The Lancet Regional Health – Americas. 2023; 18 :100423. DOI: 10.1016/j.lana.2022.100423
- 22. Hernandez-Bernal F, Ricardo-Cobas MC, Martin-Bauta Y, Rodriguez-Martinez E, Urrutia-Perez K, Urrutia-Perez K, et al. A phase 3, randomised, double-blind, placebo-controlled clinical trial evaluation of the efficacy and safety of a SARS-CoV-2 recombinant spike RBD protein vaccine in adults (ABDALA-3 study). The Lancet Regional Health – Americas. 2023; 21 :100497. DOI: 10.1016/j.lana.2023.100497
- 23. Cinza-Estévez Z, Resik-Aguirre S, Figueroa-Baile NL, Oquendo-Martinez R, Campa-Legra I, Tejeda-Fuentes A, et al. Immunogenicity and safety assessment of a SARS-CoV-2 recombinant spike RBD protein vaccine (Abdala) in paediatric ages 3 to 18 years old: A double-blinded, multicentre, randomised, phase 1/2 clinical trial (ISMAELILLO study). EClinicalMedicine. 18 Aug 2023; 63 :102160. DOI: 10.1016/j.eclinm.2023.102160. PMID: 37649806; PMCID: PMC10462868
- 24. Li G, Cappuccini F, Marchevsky NG, Aley PK, Aley R, Anslow R, et al. Safety and immunogenicity of the ChAdOx1 nCoV-19 (AZD1222) vaccine in children aged 6-17 years: A preliminary report of COV006, a phase 2 single-blind, randomised, controlled trial. Lancet. 2022; 399 (10342):2212-2225. DOI: 10.1016/S0140-6736(22)00770-X
- 25. Folegatti PM, Ewer KJ, Aley PK, Angus B, Becker S, Belij-Rammerstorfer S, et al. Safety and immunogenicity of the ChAdOx1 nCoV-19 vaccine against SARS-CoV-2: A preliminary report of a phase 1/2, single-blind, randomised controlled trial. Lancet. 2020; 396 (10249):467-478. DOI: 10.1016/S0140-6736(20)31604-4
- 26. WHO. Background Document on the AZD1222 Vaccine against COVID-19 developed by Oxford University and AstraZeneca. 2021. Available from: https://www.bing.com/search?pglt=41&q=Oxford-Astrazeneca+immunogenicity&cvid=d2a72d23020d4bb6becbd835dbfc9e6a&aqs=edge..69i57.5465j0j1&FORM=ANSPA1&PC=SMTS
- 27. Chau NVV, Nguyet LA, Truong NT, Toan LM, Dung NT, Hung LM, et al. Immunogenicity of Oxford-AstraZeneca COVID-19 vaccine in Vietnamese health-care workers. The American Journal of Tropical Medicine and Hygiene. 2022; 106 (2):556-561. DOI: 10.4269/ajtmh.21-0849
- 28. Knoll MD, Wonodi C. Oxford-AstraZeneca COVID-19 vaccine efficacy. Lancet. 2021; 397 (10269):72-74. DOI: 10.1016/S0140-6736(20)32623-4
- 29. WHO. The Oxford/AstraZeneca (ChAdOx1-S [recombinant] vaccine) COVID-19 vaccine: What you need to know. 2022. Available from: https://www.who.int/news-room/feature-stories/detail/the-oxford-astrazeneca-covid-19-vaccine-what-you-need-to-know
- 30. Sadoff J, Le Gars M, Shukarev G, Heerwegh D, Truyers C, de Groot AM, et al. Interim results of a phase 1-2a trial of Ad26.COV2.S Covid-19 vaccine. The New England Journal of Medicine. 2021; 384 (19):1824-1835. DOI: 10.1056/NEJMoa2034201
- 31. Stephenson KE, Le Gars M, Sadoff J, de Groot AM, Heerwegh D, Truyers C, et al. Immunogenicity of the Ad26.COV2.S vaccine for COVID-19. JAMA. 2021; 325 (15):1535-1544. DOI: 10.1001/jama.2021.3645
- 32. Sadoff J, Gray G, Vandebosch A, Cardenas V, Shukarev G, Grinsztejn B, et al. Safety and efficacy of single-dose Ad26.COV2.S vaccine against Covid-19. The New England Journal of Medicine. 2021; 384 (23):2187-2201. DOI: 10.1056/NEJMoa2101544
- 33. NIH. Single-Shot COVID-19 Vaccine Produces Immune Response Against Variants. 2021. Available from: https://www.nih.gov/news-events/nih-research-matters/single-shot-covid-19-vaccine-produces-immune-response-against-variants
- 34. WHO. The Janssen Ad26.COV2.S COVID-19 vaccine: What you need to know. 2022. Available from: https://www.who.int/news-room/feature-stories/detail/the-j-j-covid-19-vaccine-what-you-need-to-know
- 35. Tukhvatulin AI, Dolzhikova IV, Shcheblyakov DV, Zubkova OV, Dzharullaeva AS, Kovyrshina AV, et al. An open, non-randomised, phase 1/2 trial on the safety, tolerability, and immunogenicity of single-dose vaccine “Sputnik light” for prevention of coronavirus infection in healthy adults. The Lancet Regional Health - Europe. 2021; 11 :100241. DOI: 10.1016/j.lanepe.2021.100241
- 36. Sputnik V. About Sputnik Light. 2023. Available from: https://sputnikvaccine.com/about-vaccine/sputnik-light/
- 37. WHO. Background document on the Cansino Ad5-nCoV-S vaccine (Convidecia ®) against COVID-19. 2022. Available from: https://www.who.int/publications/i/item/WHO-2019-nCoV-vaccines-SAGE-recommendation-Ad5-nCoV-Convidecia-background
- 38. WHO. The CanSino Biologics Ad5-nCoV-S [recombinant] COVID-19 vaccine: What you need to know. 2022. Available from: https://www.who.int/news-room/feature-stories/detail/the--cansino-biologics-ad5-ncov-s--recombinant---covid-19-vaccine--what-you-need-to-know
- 39. Rose W, Raju R, Babji S, George A, Madhavan R, Leander Xavier JV, et al. Immunogenicity and safety of homologous and heterologous booster vaccination of ChAdOx1 nCoV-19 (COVISHIELD) and BBV152 (COVAXIN(R)): A non-inferiority phase 4, participant and observer-blinded, randomised study. The Lancet Regional Health – Southeast Asia. 2023; 12 :100141. DOI: 10.1016/j.lansea.2023.100141
- 40. Frenck RW Jr, Klein NP, Kitchin N, Gurtman A, Absalon J, Lockhart S, et al. Safety, immunogenicity, and efficacy of the BNT162b2 Covid-19 vaccine in adolescents. The New England Journal of Medicine. 2021; 385 (3):239-250. DOI: 10.1056/NEJMoa2107456
- 41. Rosa Duque JS, Wang X, Leung D, Cheng SMS, Cohen CA, Mu X, et al. Author correction: Immunogenicity and reactogenicity of SARS-CoV-2 vaccines BNT162b2 and CoronaVac in healthy adolescents. Nature Communications. 2022; 13 (1):4798. DOI: 10.1038/s41467-022-32337-6
- 42. Zheng C, Shao W, Chen X, Zhang B, Wang G, Zhang W. Real-world effectiveness of COVID-19 vaccines: A literature review and meta-analysis. International Journal of Infectious Diseases. 2022; 114 :252-260. DOI: 10.1016/j.ijid.2021.11.009
- 43. Pfizer. Pfizer and BioNTech Announce Omicron-Adapted COVID-19 Vaccine Candidates Demonstrate High Immune Response Against Omicron. 2022. Available from: https://www.pfizer.com/news/press-release/press-release-detail/pfizer-and-biontech-announce-omicron-adapted-covid-19
- 44. moderna. Overview of Moderna’s COVID-19 Vaccine (mRNA-1273). 2020. Available from: https://www.cdc.gov/vaccines/acip/meetings/downloads/slides-2020-12/slides-12-19/02-COVID-Miller-508.pdf
- 45. moderna. Safety and Immunogenicity of a 50 μg Booster Dose of Moderna COVID-19 Vaccine. 2021. Available from: https://www.cdc.gov/vaccines/acip/meetings/downloads/slides-2021-10-20-21/02-COVID-Miller-508.pdf
- 46. Momin T, Kansagra K, Patel H, Sharma S, Sharma B, Patel J, et al. Safety and immunogenicity of a DNA SARS-CoV-2 vaccine (ZyCoV-D): Results of an open-label, non-randomized phase I part of phase I/II clinical study by intradermal route in healthy subjects in India. EClinicalMedicine. 2021; 38 :101020. DOI: 10.1016/j.eclinm.2021.101020
- 47. Dey A, Chozhavel Rajanathan TM, Chandra H, Pericherla HPR, Kumar S, Choonia HS, et al. Immunogenic potential of DNA vaccine candidate, ZyCoV-D against SARS-CoV-2 in animal models. Vaccine. 2021; 39 (30):4108-4116. DOI: 10.1016/j.vaccine.2021.05.098
- 48. Tian JH, Patel N, Haupt R, Zhou H, Weston S, Hammond H, et al. SARS-CoV-2 spike glycoprotein vaccine candidate NVX-CoV2373 immunogenicity in baboons and protection in mice. Nature Communications. 2021; 12 (1):372. DOI: 10.1038/s41467-020-20653-8
- 49. Heath PT, Galiza EP, Baxter DN, Boffito M, Browne D, Burns F, et al. Safety and efficacy of NVX-CoV2373 Covid-19 vaccine. The New England Journal of Medicine. 2021; 385 (13):1172-1183. DOI: 10.1056/NEJMoa2107659
- 50. Alves K, Plested JS, Galbiati S, Chau G, Cloney-Clark S, Zhu M, et al. Immunogenicity of a fourth homologous dose of NVX-CoV2373. The New England Journal of Medicine. 2023; 388 (9):857-859. DOI: 10.1056/NEJMc2215509
- 51. ISAAA. Medicago’s Plant-Based COVID-19 Vaccine Shows Positive Phase 2 Results. 2021. Available from: https://www.isaaa.org/kc/cropbiotechupdate/article/default.asp?ID=18790
- 52. GSK. Medicago and GSK Announce Positive Phase 3 efficacy and Safety results for Adjuvanted Plant-based COVID-19 Vaccine candidate. 2021. Available from: https://www.gsk.com/en-gb/media/press-releases/medicago-and-gsk-announce-positive-phase-3-efficacy-and-safety-results/
- 53. Jin L, Li Z, Zhang X, Li J, Zhu F. CoronaVac: A review of efficacy, safety, and immunogenicity of the inactivated vaccine against SARS-CoV-2. Human Vaccines & Immunotherapeutics. 2022; 18 (6):2096970. DOI: 10.1080/21645515.2022.2096970
- 54. Ella R, Reddy S, Blackwelder W, Potdar V, Yadav P, Sarangi V, et al. Efficacy, safety, and lot-to-lot immunogenicity of an inactivated SARS-CoV-2 vaccine (BBV152): Interim results of a randomised, double-blind, controlled, phase 3 trial. Lancet. 2021; 398 (10317):2173-2184. DOI: 10.1016/S0140-6736(21)02000-6
- 55. Saeed BQ, Al-Shahrabi R, Alhaj SS, Alkokhardi ZM, Adrees AO. Side effects and perceptions following Sinopharm COVID-19 vaccination. International Journal of Infectious Diseases. 2021; 111 :219-226. DOI: 10.1016/j.ijid.2021.08.013
- 56. WHO. The Novavax vaccine against COVID-19: What you need to know. 2022. Available from: https://www.who.int/news-room/feature-stories/detail/the-novavax-vaccine-against-covid-19-what-you-need-to-know
- 57. WHO. The Pfizer BioNTech (BNT162b2) COVID-19 vaccine: What you need to know. 2022. Available from: https://www.who.int/news-room/feature-stories/detail/who-can-take-the-pfizer-biontech-covid-19--vaccine-what-you-need-to-know
- 58. WHO. The Moderna COVID-19 (mRNA-1273) vaccine: what you need to know. 2022. Available from: https://www.who.int/news-room/feature-stories/detail/the-moderna-covid-19-mrna-1273-vaccine-what-you-need-to-know
- 59. Khobragade A, Bhate S, Ramaiah V, Deshpande S, Giri K, Phophle H, et al. Efficacy, safety, and immunogenicity of the DNA SARS-CoV-2 vaccine (ZyCoV-D): The interim efficacy results of a phase 3, randomised, double-blind, placebo-controlled study in India. Lancet. 2022; 399 (10332):1313-1321. DOI: 10.1016/S0140-6736(22)00151-9
- 60. Dotiwala F, Upadhyay AK. A comprehensive review of BBV152 vaccine development, effectiveness, safety, challenges, and prospects. Frontiers in Immunology. 2022; 13 :940715. DOI: 10.3389/fimmu.2022.940715
- 61. Bonnema CE, van Woerden I, Steinberg JR, Nguyen E, Oliphant CM, Cleveland KW, et al. Understanding COVID-19 vaccine hesitancy among students in health professions: A cross-sectional analysis. Journal of Allied Health. 2021; 50 (4):314-320 Available from: https://www.ncbi.nlm.nih.gov/pubmed/34845489
- 62. Knight H, Jia R, Ayling K, Bradbury K, Baker K, Chalder T, et al. Understanding and addressing vaccine hesitancy in the context of COVID-19: Development of a digital intervention. Public Health. 2021; 201 :98-107. DOI: 10.1016/j.puhe.2021.10.006
- 63. Machingaidze S, Wiysonge CS. Understanding COVID-19 vaccine hesitancy. Nature Medicine. 2021; 27 (8):1338-1339. DOI: 10.1038/s41591-021-01459-7
- 64. Rozek LS, Jones P, Menon A, Hicken A, Apsley S, King EJ. Understanding vaccine hesitancy in the context of COVID-19: The role of trust and confidence in a seventeen-country survey. International Journal of Public Health. 2021; 66 :636255. DOI: 10.3389/ijph.2021.636255
- 65. Whiteley WN, Ip S, Cooper JA, Bolton T, Keene S, Walker V, et al. Association of COVID-19 vaccines ChAdOx1 and BNT162b2 with major venous, arterial, or thrombocytopenic events: A population-based cohort study of 46 million adults in England. PLoS Medicine. 2022; 19 (2):e1003926. DOI: 10.1371/journal.pmed.1003926
- 66. MedicalNewsToday. COVID-19 Vaccines and Blood clots: Two Large Studies Investigate. 2022. Available from: https://www.medicalnewstoday.com/articles/covid-19-vaccines-and-blood-clots-two-large-studies-investigate
- 67. Acharya KP, Ghimire TR, Subramanya SH. Access to and equitable distribution of COVID-19 vaccine in low-income countries. npj Vaccines. 2021; 6 (1):54. DOI: 10.1038/s41541-021-00323-6
- 68. Fahrni ML, Ismail IA, Refi DM, Almeman A, Yaakob NC, Saman KM, et al. Management of COVID-19 vaccines cold chain logistics: A scoping review. Journal of Pharmaceutical Policy and Practice. 2022; 15 (1):16. DOI: 10.1186/s40545-022-00411-5
- 69. CDC. Severe Acute Respiratory Syndrome (SARS). 2017. Available from: https://www.cdc.gov/sars/index.html
- 70. CDC. Middle East Respiratory Syndrome (MERS). 2019. Available from: https://www.cdc.gov/coronavirus/mers/
- 71. V'Kovski P, Kratzel A, Steiner S, Stalder H, Thiel V. Coronavirus biology and replication: Implications for SARS-CoV-2. Nature Reviews. Microbiology. 2021; 19 (3):155-170. DOI: 10.1038/s41579-020-00468-6
- 72. Huang Y, Yang C, Xu XF, Xu W, Liu SW. Structural and functional properties of SARS-CoV-2 spike protein: Potential antivirus drug development for COVID-19. Acta Pharmacologica Sinica. 2020; 41 (9):1141-1149. DOI: 10.1038/s41401-020-0485-4
- 73. Sanyal S. How SARS-CoV-2 (COVID-19) spreads within infected hosts - what we know so far. Emerging Topics in Life Sciences. 2020; 4 (4):371-378. DOI: 10.1042/ETLS20200165
- 74. Zhang Y, Geng X, Tan Y, Li Q, Xu C, Xu J, et al. New understanding of the damage of SARS-CoV-2 infection outside the respiratory system. Biomedicine & Pharmacotherapy. 2020; 127 :110195. DOI: 10.1016/j.biopha.2020.110195
- 75. Gavriatopoulou MKE, Fotiou D, Ntanasis-Stathopoulos I, Psaltopoulou T, Kastritis E, Terpos E, et al. Organ-specific manifestations of COVID-19 infection. Clinical and Experimental Medicine. 2020; 20 (4):493-506. DOI: 10.1007/s10238-020-00648-x
- 76. Brodin P. Immune determinants of COVID-19 disease presentation and severity. Nature Medicine. 2021; 27 (1):28-33. DOI: 10.1038/s41591-020-01202-8
- 77. Schiuma G, Beltrami S, Bortolotti D, Rizzo S, Rizzo R. Innate immune response in SARS-CoV-2 infection. Microorganisms. 23 Feb 2022; 10 (3):501. DOI: 10.3390/microorganisms10030501. PMID: 35336077; PMCID: PMC8950297
- 78. Diamond MS, Kanneganti TD. Innate immunity: The first line of defense against SARS-CoV-2. Nature Immunology. 2022; 23 (2):165-176. DOI: 10.1038/s41590-021-01091-0
- 79. Post N, Eddy D, Huntley C, van Schalkwyk MCI, Shrotri M, Leeman D, et al. Antibody response to SARS-CoV-2 infection in humans: A systematic review. PLoS One. 2020; 15 (12):e0244126. DOI: 10.1371/journal.pone.0244126
- 80. Wang X, Yuen TT, Dou Y, Hu J, Li R, Zeng Z, et al. Vaccine-induced protection against SARS-CoV-2 requires IFN-gamma-driven cellular immune response. Nature Communications. 2023; 14 (1):3440. DOI: 10.1038/s41467-023-39096-y
- 81. Markov PV, Ghafari M, Beer M, Lythgoe K, Simmonds P, Stilianakis NI, et al. The evolution of SARS-CoV-2. Nature Reviews. Microbiology. 2023; 21 (6):361-379. DOI: 10.1038/s41579-023-00878-2
- 82. Zabidi NZ, Liew HL, Farouk IA, Puniyamurti A, Yip AJW, Wijesinghe VN, et al. Evolution of SARS-CoV-2 variants: Implications on immune escape, vaccination, therapeutic and diagnostic strategies. Viruses. 10 Apr 2023; 15 (4):944. DOI: 10.3390/v15040944. PMID: 37112923; PMCID: PMC10145020
- 83. Ren W, Zhang Y, Rao J, Wang Z, Lan J, Liu K, et al. Evolution of immune evasion and host range expansion by the SARS-CoV-2 B.1.1.529 (omicron) Variant. mBio. 2023; 14 (2):e0041623. DOI: 10.1128/mbio.00416-23
- 84. Mondi A, Mastrorosa I, Piselli P, Cimaglia C, Matusali G, Carletti F, et al. Evolution of SARS-CoV-2 variants of concern over a period of Delta and omicron cocirculation, among patients hospitalized for COVID-19 in an Italian reference hospital: Impact on clinical outcomes. Journal of Medical Virology. 2023; 95 (6):e28831. DOI: 10.1002/jmv.28831
- 85. Smith CA, Ashby B. Antigenic evolution of SARS-CoV-2 in immunocompromised hosts. Evolution, Medicine, and Public Health. 2023; 11 (1):90-100. DOI: 10.1093/emph/eoac037
- 86. COVID19VaccineTracker. 2023. Available from: https://covid19.trackvaccines.org/
- 87. Volz A, Sutter G. Modified vaccinia virus ankara: History, value in basic research, and current perspectives for vaccine development. Advances in Virus Research. 2017; 97 :187-243. DOI: 10.1016/bs.aivir.2016.07.001. Epub 2016 Aug 1. PMID: 28057259; PMCID: PMC7112317
- 88. Prompetchara E, Ketloy C, Alameh MG, Tharakhet K, Kaewpang P, Yostrerat N, et al. Immunogenicity and protective efficacy of SARS-CoV-2 mRNA vaccine encoding secreted non-stabilized spike in female mice. Nature Communications. 2023; 14 (1):2309. DOI: 10.1038/s41467-023-37795-0
- 89. Pollard AJ, Bijker EM. Publisher correction: A guide to vaccinology: From basic principles to new developments. Nature Reviews. Immunology. 2021; 21 (2):129. DOI: 10.1038/s41577-020-00497-5
- 90. Jeyanathan M, Afkhami S, Smaill F, Miller MS, Lichty BD, Xing Z. Immunological considerations for COVID-19 vaccine strategies. Nature Reviews. Immunology. 2020; 20 (10):615-632. DOI: 10.1038/s41577-020-00434-6
- 91. WHO. Safety of COVID-19 Vaccines. 2022. Available from: https://www.who.int/news-room/feature-stories/detail/safety-of-covid-19-vaccines
- 92. CDC. Safety of COVID-19 Vaccines. 2023. Available from: https://www.cdc.gov/coronavirus/2019-ncov/vaccines/safety/safety-of-vaccines.html
- 93. Wu Q, Dudley MZ, Chen X, Bai X, Dong K, Zhuang T, et al. Evaluation of the safety profile of COVID-19 vaccines: A rapid review. BMC Medicine. 2021; 19 (1):173. DOI: 10.1186/s12916-021-02059-5
- 94. Grana C, Ghosn L, Evrenoglou T, Jarde A, Minozzi S, Bergman H, et al. Efficacy and safety of COVID-19 vaccines. Cochrane Database of Systematic Reviews. 2022; 12 (12):CD015477. DOI: 10.1002/14651858.CD015477
- 95. UK Health Security Agency. COVID-19 Vaccine Surveillance report Week 42. 2021. Available from: https://assets.publishing.service.gov.uk/government/uploads/system/uploads/attachment_data/file/1027511/Vaccine-surveillance-report-week-42.pdf
- 96. He Q, Mao Q, An C, Zhang J, Gao F, Bian L, et al. Heterologous prime-boost: Breaking the protective immune response bottleneck of COVID-19 vaccine candidates. Emerging Microbes & Infections. 2021; 10 (1):629-637. DOI: 10.1080/22221751.2021.1902245
- 97. Pozzetto B, Legros V, Djebali S, Barateau V, Guibert N, Villard M, et al. Immunogenicity and efficacy of heterologous ChAdOx1-BNT162b2 vaccination. Nature. 2021; 600 (7890):701-706. DOI: 10.1038/s41586-021-04120-y
- 98. MoH. Covid-19 Vaccination Progress Report. 2023. Available from: https://www.facebook.com/photo?fbid=671847058305394&set=pcb.671847121638721
- 99. Mills EJ, Reis G. Evaluating COVID-19 vaccines in the real world. Lancet. 2022; 399 (10331):1205-1206. DOI: 10.1016/S0140-6736(22)00194-5
- 100. G7Summit. (2021). 100 days Mission to Respond to Future Pandemic Threats. Available from: https://assets.publishing.service.gov.uk/government/uploads/system/uploads/attachment_data/file/992762/100_Days_Mission_to_respond_to_future_pandemic_threats__3_.pdf
- 101. CDC. (2021). Importance of One Health for COVID-19 and Future Pandemics. Available from: https://www.cdc.gov/media/releases/2021/s1103-one-health.html
© The Author(s). Licensee IntechOpen. This chapter is distributed under the terms of the Creative Commons Attribution 3.0 License , which permits unrestricted use, distribution, and reproduction in any medium, provided the original work is properly cited.
Continue reading from the same book
Edited by Márcia Aparecida Sperança
Published: 22 May 2024
By Moussa Benboubker, Bouchra Oumokhtar, Fouzia Hmami...
36 downloads
By Christian Carlos Zurita-Lizza, Ignacio Rodriguez-S...
62 downloads
By Luis Alberto Camputaro, Alejandro Jose Duarte Cuel...
55 downloads
COVID-19 InfoVaccines: A WHO-supported educational project to promote COVID-19 vaccination information among professionals and the general population
Affiliations.
- 1 Genetics, Vaccines and Pediatric Infectious Diseases Research Group (GENVIP), Instituto de Investigación Sanitaria de Santiago de Compostela, Santiago de Compostela, Spain.
- 2 WHO Collaborating Centre for Vaccine Safety, Santiago de Compostela, Spain.
- 3 Centro de Investigación Biomédica en Red de Epidemiología y Salud Pública (CIBER-ESP), Madrid, Spain.
- 4 Department of Preventive Medicine, University of Santiago de Compostela (USC), Santiago de Compostela, Spain.
- 5 Centro de Investigación Biomédica en Red de Enfermedades Respiratorias (CIBER-ES), Instituto de Salud Carlos III, Madrid, Spain.
- 6 Translational Pediatrics and Infectious Diseases, Hospital Clínico Universitario de Santiago de Compostela and USC, Santiago de Compostela, Spain.
- 7 Servicio de Medicina Preventiva y Salud Pública, Hospital Vital Álvarez Buylla, Asturias, Spain.
- 8 World Health Organization Regional Office for Europe, Copenhagen, Denmark.
- 9 English-Russian medical interpreter/translator, Russian Federation.
- 10 Head of Design and Development Marketing agency, Sisuco Solutions, Madrid, Spain.
- 11 World Health Organization Country Office in Ukraine, Kyiv, Ukraine.
- 12 Digital Marketing Consultant & Project Manager, World Health Organization, Georgia.
- 13 World Health Organization Country Office, Czechia.
- 14 World Health Organization Country Office, Bucharest, Romania.
- PMID: 38782400
- PMCID: PMC11123498
- DOI: 10.1080/21645515.2024.2350817
COVID-19 vaccine uptake varied across countries, in part due to vaccine hesitancy fueled by a lack of trustworthy information. To help health workers provide evidence-based answers to common questions about COVID-19 vaccines and vaccination, and thereby, assist individuals´ decisions on vaccine acceptance, COVID-19 InfoVaccines, a joint WHO-EU project, was launched in February 2021 to support COVID-19 vaccine rollout in 6 Eastern European countries. COVID-19 InfoVaccines was made available in seven languages and shared on social media networks. A total of 262,592 users accessed COVID-19 InfoVaccines.com between February 11, 2021, and January 31 st , 2023. The users were most interested in: general questions; vaccine efficacy and duration of protection; vaccine safety; vaccine co-administration, and dose-interval and interchangeability; though the interest in a specific theme varied in function of the epidemiological situation. A total of 118,510 (45.1%) and 46,644 (17.7%) users scrolled up to 35% and 75% of the COVID-19 InfoVaccines webpage, respectively. The average engagement rate was 71.61%. The users accessed COVID-19 InfoVaccines from 231 countries and territories, but the majority were in Ukraine ( N = 38,404; 14.6%), Spain ( N = 23,327; 8.9%), and Argentina ( N = 21,167; 8.1%). Older Facebook users were more interested in COVID-19 information than younger individuals ( X 2 p-value < .0001). Two hundred twenty-eight videos were shared on YouTube. The average Click-Through-Rate on Facebook was 7.82%, and that on YouTube was 4.4%, with 60 videos having a Click-Through-Rate >5%, falling in the range of average YouTube video Click-Through-Rate (2% - 10%). As misinformation about vaccines and vaccination spreads easily and can negatively impact health-related decisions, initiatives like COVID-19 InfoVaccines are crucial to facilitate access to reliable information.
Keywords: COVID-19; COVID-19 InfoVaccines; Europe; World Health Organization; educational tool; misinformation; vaccine; vaccine hesitancy.
- COVID-19 Vaccines* / administration & dosage
- COVID-19* / epidemiology
- COVID-19* / prevention & control
- Health Education / methods
- Health Personnel / statistics & numerical data
- SARS-CoV-2 / immunology
- Social Media*
- Vaccination Hesitancy / statistics & numerical data
- Vaccination* / psychology
- Vaccination* / statistics & numerical data
- World Health Organization
- COVID-19 Vaccines
Grants and funding
Advertisement
Introduction
Acknowledgments, quantitative analysis of mrna-1273 covid-19 vaccination response in immunocompromised adult hematology patients.

- Split-Screen
- Request Permissions
- Cite Icon Cite
- Search Site
- Open the PDF for in another window
Sabine Haggenburg , Birgit I. Lissenberg-Witte , Rob S. van Binnendijk , Gerco den Hartog , Michel S. Bhoekhan , Nienke J. E. Haverkate , Dennis M. de Rooij , Johan van Meerloo , Jacqueline Cloos , Neeltje A. Kootstra , Dorine Wouters , Suzanne S. Weijers , Ester M. M. van Leeuwen , Hetty J. Bontkes , Saïda Tonouh-Aajoud , Mirjam H. M. Heemskerk , Rogier W. Sanders , Elianne Roelandse-Koop , Quincy Hofsink , Kazimierz Groen , Lucia Çetinel , Louis Schellekens , Yvonne M. den Hartog , Belle Toussaint , Iris M. J. Kant , Thecla Graas , Emma de Pater , Willem A. Dik , Marije D. Engel , Cheyenne R. N. Pierie , Suzanne R. Janssen , Edith van Dijkman , Meliawati Poniman , Judith A. Burger , Joey H. Bouhuijs , Gaby Smits , Nynke Y. Rots , Sonja Zweegman , Arnon P. Kater , Tom van Meerten , Pim G. N. J. Mutsaers , Jaap A. van Doesum , Annoek E. C. Broers , Marit J. van Gils , Abraham Goorhuis , Caroline E. Rutten , Mette D. Hazenberg , Inger S. Nijhof; Quantitative analysis of mRNA-1273 COVID-19 vaccination response in immunocompromised adult hematology patients. Blood Adv 2022; 6 (5): 1537–1546. doi: https://doi.org/10.1182/bloodadvances.2021006917
Download citation file:
- Ris (Zotero)
- Reference Manager
Immunochemotherapy does not preclude adequate antibody responses in hematology patients, except when B cells are absent or dysfunctional.
During the pandemic, COVID-19 vaccination should not be delayed in hematology patients.
Visual Abstract

Vaccination guidelines for patients treated for hematological diseases are typically conservative. Given their high risk for severe COVID-19, it is important to identify those patients that benefit from vaccination. We prospectively quantified serum immunoglobulin G (IgG) antibodies to spike subunit 1 (S1) antigens during and after 2-dose mRNA-1273 (Spikevax/Moderna) vaccination in hematology patients. Obtaining S1 IgG ≥ 300 binding antibody units (BAUs)/mL was considered adequate as it represents the lower level of S1 IgG concentration obtained in healthy individuals, and it correlates with potent virus neutralization. Selected patients (n = 723) were severely immunocompromised owing to their disease or treatment thereof. Nevertheless, >50% of patients obtained S1 IgG ≥ 300 BAUs/mL after 2-dose mRNA-1273. All patients with sickle cell disease or chronic myeloid leukemia obtained adequate antibody concentrations. Around 70% of patients with chronic graft-versus-host disease (cGVHD), multiple myeloma, or untreated chronic lymphocytic leukemia (CLL) obtained S1 IgG ≥ 300 BAUs/mL. Ruxolitinib or hypomethylating therapy but not high-dose chemotherapy blunted responses in myeloid malignancies. Responses in patients with lymphoma, patients with CLL on ibrutinib, and chimeric antigen receptor T-cell recipients were low. The minimal time interval after autologous hematopoietic cell transplantation (HCT) to reach adequate concentrations was <2 months for multiple myeloma, 8 months for lymphoma, and 4 to 6 months after allogeneic HCT. Serum IgG4, absolute B- and natural killer–cell number, and number of immunosuppressants predicted S1 IgG ≥ 300 BAUs/mL. Hematology patients on chemotherapy, shortly after HCT, or with cGVHD should not be precluded from vaccination. This trial was registered at Netherlands Trial Register as #NL9553.
Since the outbreak of the severe acute respiratory syndrome coronavirus 2 (SARS-CoV-2) pandemic, it has become apparent that patients with hematologic diseases are at the highest risk for severe COVID-19, COVID-19–related death, and persistent viral shedding. 1-4 In a large population-based study including >17 million residents of England, it was demonstrated that the mortality risk of SARS-CoV-2 infection in patients diagnosed with a hematologic malignancy in the past 5 years was >2.5-fold higher compared with individuals who had never been diagnosed with a malignant hematologic condition. 1 Mortality in patients with sickle cell disease was 5% compared with 0% in a matched cohort of health care professionals. 2 In early 2021, the Dutch government decided to prioritize COVID-19 vaccination in immunocompromised individuals, including patients with hematologic conditions. Given the pressure on the health care system at that time in the pandemic and the urgent need to protect as many individuals as possible, it was decided to vaccinate all patients, irrespective of current disease or treatment status.
This allowed us to prospectively measure the immunogenicity of the mRNA-1273 (Moderna/Spikevax) vaccination in those patients that are generally considered too immunocompromised to benefit from vaccination and in whom vaccinations are often postponed until (later) after treatment, such as patients currently receiving, or just after (immuno-)chemotherapy, early after transplantation, or after CD19 chimeric antigen receptor (CAR) T-cell therapy, and in patients receiving novel, targeted therapies. 5,6 By quantifying antibody concentrations against the World Health Organization (WHO) standard, 7 we were able to identify those patients that despite their underlying condition mounted an adequate antibody response after mRNA-1273 vaccination. Our results demonstrate that in the midst of the pandemic, COVID-19 vaccination should not be postponed in hematology patients, even when under active or shortly after (immuno-)chemotherapy or cell therapy.
Study participants
In this prospective, observational multicenter cohort study, we included 723 adult hematology patients, classified into 17 different predefined cohorts based on their diagnosis and treatment status ( Table 1 ; supplemental Table 1). Patient cohorts were defined based on relevance for the hematology practice (eg, disease incidence [chronic lymphocytic leukemia (CLL), multiple myeloma] and knowledge gap [less often studied agents in the context of vaccination or often excluded treatment groups]), with the aim to cover benign, lymphoid, and myeloid diseases. Inclusion criteria were age ≥18 years, a diagnosis of lymphoma, multiple myeloma, CLL, acute myeloid leukemia (AML), myelodysplastic syndrome (MDS), myeloproliferative disease, sickle cell disease, and receiving immunochemotherapy or having received such therapy preferably 6 months but maximally 12 months prior to vaccination, or received targeted agents, or have received autologous or allogeneic hematopoietic cell transplantation (HCT) preferably 6 months but maximally 12 months prior to vaccination, or having received CD19-directed CAR T-cell therapy. All these patients are considered immunocompromised, which is also reflected by their increased risk of COVID-19–related death. 1 Exclusion criteria included unwilling or unable to give informed consent, known allergy to one of the components of the vaccine, and a life expectancy of <12 months. Prior infection with SARS-CoV-2 was not an exclusion criterion because the expected seroprevalence was <5% (because of the stringent isolation measures in this patient population), and a test-first strategy for seroprevalence would seriously hamper the speed of vaccination rollout, whereas vaccination of seropositive patients was indicated nonetheless, according to the national vaccination guidelines. All participants received 2 doses of mRNA-1273 28 days apart ( Figure 1A ). Study protocols were approved by the Institutional Review Board of the Amsterdam UMC and participating centers. All patients provided written informed consent prior to study onset.
Baseline characteristics stratified by cohorts
WHO PS, World Health Organization Performance Status.
N IgG ≥ 14.3 BAUs/mL at baseline.
See supplemental Table 1 for clinical details.
B-NHL: n = 21; Hodgkin lymphoma: n = 2; T-NHL: n = 8.
Plus venetoclax: n = 8; plus obinutuzumab: n = 2.
Hypomethylating therapy: azacitidine (n = 10), azacitidine plus venetoclax (n = 4), decitabine (n = 4), and decitabine plus midostaurine (n = 1).

Study synopsis. Study protocol synopsis (A) and patient inclusion (B).
Clinical parameters
Prevaccination baseline leukocyte count, humoral and cellular immune parameters, including quantitative assessment of lymphocytes, B-, T-, and natural killer (NK)-cell numbers, and serum immunoglobulin M (IgM), IgG, and IgG subtypes 1 to 4, demographic parameters, and medical history, including comorbidities and concomitant medication, were collected prospectively prior to each vaccination and 28 days after the second vaccination ( Figure 1A ). Clinical data were collected via standardized case report forms.
Antibody concentrations, definitions, and neutralization
Humoral responses (IgG) against subunit 1 (S1), receptor binding domain (RBD), and nucleocapsid (N) antigen domains of SARS-CoV-2 were determined quantitatively and centrally, using a bead-based multiplex immune assay. 8 SARS-CoV-2 binding antibody concentration was calibrated against the first serum standard for COVID-19 (20/136), as provided by the National Institute for Biological Standards Control, also recommended by the WHO to define specific serum antibody concentrations in an international perspective. 7-9 Seroconversion was defined as obtaining an S1 IgG concentration >10 binding antibody units (BAUs)/mL, and an adequate vaccine response as S1 IgG ≥ 300 BAUs/mL, the IgG concentration that met a plaque reduction neutralization titer 50 of 40 or higher in 2 independent prospective Dutch mRNA-1273 vaccination cohorts. 10-13 Reference antibody levels were extracted from the PIENTER cohort, 14 a nationwide surveillance cohort with >3000 randomly selected Dutch citizens between 2 and 90 years of age that periodically provide a self-collected fingerstick blood sample and complete an online questionnaire. From this cohort, we selected all age-matched participants who had received a second dose of mRNA-1273 14 to 61 days prior to blood sampling (supplemental Table 2). To test antibody neutralization activity, we generated lentiviral-based pseudoviruses expressing the SARS-CoV-2 wild-type variant (D614G) and determined the 50% inhibitory dose as the dilution of plasma with a 50% reduction of infection using HEK293T-ACE2 cells in all patients with 50 to 300 S1 IgG BAUs/mL and in a random selection of patients with S1 IgG ≥ 300 BAUs/mL (supplemental Table 3). 15 SARS-CoV-2 pseudovirus neutralization correlated significantly with authentic SARS-CoV-2 neutralization as measured by plaque reduction neutralization titer or virus neutralization test. 15
Statistical analysis
We aimed to include n = 50 patients per subgroup to estimate S1 IgG ≥ 300 BAUs/mL titers with a precision between 7% (at 0 or 100%) and 29% (at 50%) for Clopper-Pearson (exact) 95% confidence intervals (CI). Differences between groups and timepoints were analyzed with Mann-Whitney U and Wilcoxon signed-rank tests, respectively. Univariable and multivariable logistic regression models were used to identify factors associated with S1 IgG ≥ 300 BAUs/mL 4 weeks after the second vaccination. Factors tested include prevaccination baseline leukocyte count, humoral and cellular immune parameters including lymphocyte, B-, T-, and NK-cell numbers, serum IgM, IgG, and IgG subtypes 1 to 4, demographic parameters, and immunosuppressive therapies. Multivariable models were built including all patients irrespective of cohort using a stepwise forward selection procedure ( P value for entry .05) with all univariable significant demographic, therapy-related, and immunologic parameters, first as part of their subcategory of parameter, followed by a multivariable analysis of all significant parameters together. A sensitivity analysis was performed for the last model by excluding patients with untreated CLL. Pearson correlation was calculated between serum S1 IgG and in vitro pseudovirus neutralization after 10log-transformation of both. Analyses with 2-sided P values of <.05 were considered statistically significant. Statistical analyses were performed using the IBM SPSS Statistics for Windows, Version 26.0 (IBM Corp, Armonk, NY) and R for Windows, Version 4.0.3 (The R Foundation for Statistical Computing, Vienna, Austria). Normality of continuous parameters was assessed by visual inspection of QQ plots. The linearity assumption for continuous parameters in the logistic regression models was investigated by dividing the parameter in quartiles, and parameters violating the linearity assumption were categorized in clinically relevant groups. The aim of this observational study is to identify those patients that respond sufficiently to COVID-19 vaccination. Because of the observational and descriptive nature of this study, there was no formal hypothesis testing framework.
SARS-CoV-2 antibodies and neutralization
A total of 723 patients were included, 695 of whom were available for analysis after completion of the vaccination schedule ( Figure 1B ; Table 1 ). Thirty-one study participants had sickle cell disease and were using hydroxyurea. All other participants had been diagnosed with a hematologic malignancy and were receiving or had recently received targeted therapy, tyrosine kinase inhibitor (TKI) treatment, hypomethylating therapy, (immune)chemotherapy with or without HCT, or CD19-directed CAR T-cell therapy. Median S1 and RBD binding antibody concentrations increased significantly after 2 mRNA-1273 vaccinations, but overall remained significantly lower than the reference population ( Figure 2A ; supplemental Figure 1). A minority of patients (4.9%) had N-specific IgG > 14.3 BAUs/mL at baseline, indicative of previous SARS-CoV-2 infection ( Table 1 ; supplemental Table 4). As they were primed for SARS-CoV-2 before vaccination, they had better S1 IgG responses than previously uninfected patients and obtained median S1 IgG concentrations comparable to those obtained in healthy individuals ( Figure 2A ). They were excluded from further analyses. The majority (70%) of previously uninfected participants reached seroconversion (S1 IgG > 10 BAUs/mL), and 55% obtained S1 IgG ≥ 300 BAUs/mL ( Figure 2A ; supplemental Table 5). Thirty percent of previously uninfected patients did not seroconvert at all. The correlation between serum S1 IgG and in vitro pseudovirus neutralization was strong ( r = 0.85; P < .001; Figure 2B ). In the 5 months after completion of the vaccination schedule (from 28 days after the second vaccination onwards), 17 (out of 723) patients tested SARS-CoV-2 positive. Seven of these patients received antibody therapy, either convalescent plasma (n = 2) or casirivimab/imdevimab (n = 5). All 17 patients experienced symptomatic infection, and 3 patients required hospital care, 2 of which at the ICU. All 3 hospitalized patients were nonresponders (S1 IgG < 10 BAUs/mL). None of the study participants died because of COVID-19.

S1 binding antibody concentration and neutralization. (A) IgG S1 concentration for each timepoint. Red and green: previously uninfected patients (U); blue and purple: previously infected patients (I). Dotted lines indicate seroconversion (S1 IgG > 10 BAUs/mL) and sufficient S1 IgG concentration (≥300 BAUs/mL). * P < .05. (B) Correlation of IgG S1 concentration and pseudovirus neutralization ( r = 0.85; P < .001). ID 50 , 50% inhibitory dose.
Differences between patient cohorts
Median S1 IgG increased significantly in all cohorts after 2 doses of mRNA-1273 ( Figure 3 ). An antibody concentration ≥300 BAUs/mL was obtained in 96% of patients with sickle cell disease on hydroxyurea therapy, in 100% of patients with CML on TKI therapy, and in 94% of patients with AML or high-risk MDS on or <12 months after high-dose chemotherapy ( Figure 3 ; supplemental Table 5). S1 IgG ≥ 300 BAUs/mL was also observed in more than half of patients with multiple myeloma on first-line remission-induction therapy (52%), on daratumumab-containing therapy (69%), on immunomodulatory imide drugs (IMiD; 77%), or within 9 months after high-dose melphalan and autologous HCT (89%), in patients with untreated CLL (70%), and in allogeneic HCT recipients with chronic graft-versus-host disease (cGVHD; 69%). Less than half of patients with myeloproliferative disease on ruxolitinib (49%), with AML or high-risk MDS on hypomethylating therapy (41%), with lymphoma early after BEAM (carmustine, etoposide, cytarabine, melphalan) and autologous HCT (33%), patients shortly after allogeneic HCT (33%), and patients with CLL on ibrutinib (27%) obtained S1 IgG ≥ 300 BAUs/mL. Only few patients with B-non-Hodgkin lymphoma (NHL) on (0%) or within 12 months after (26%) rituximab monotherapy or rituximab-containing immunochemotherapy, and of CD19-directed CAR T-cell therapy recipients (11%) obtained S1 IgG ≥ 300 BAUs/mL ( Figure 3 ; supplemental Table 5). The few responding patients in the CAR T-cell cohort all had normal B-cell numbers. Individual RBD binding antibody concentrations showed similar dynamics over time (not shown).

IgG S1 concentration over time for each patient. Thin lines depict previously uninfected patients (red) and previously infected patients (orange); thick lines indicate the median IgG S1 concentrations. Dotted lines specify seroconversion (S1 IgG > 10 BAUs/mL) and sufficient S1 IgG concentration (≥300 BAUs/mL). * P < .05. BEAM, carmustine, etoposide, cytarabine, melphalan.
Factors associated with antibody responses
Of the immunologic factors, demographic parameters and immunosuppressive medications identified to be associated with obtaining S1 IgG ≥ 300 BAUs/mL in the univariable models, several remained significant in a multivariable model per subcategory of variables ( Figure 4A ) and in an overall model ( Figure 4B ; supplemental Table 6). In the overall model, and across cohorts, age correlated significantly with vaccination response (odds ratio [OR], 0.87 per 5-year increase; 95% CI, 0.79-0.96; P = .005). IgG4 concentration (OR, 1.8 per g/L; CI, 1.1-3.1; P = .019) and absolute number of NK cells higher than the upper limit of normal (OR, 8.4; CI, 2.9-24.4; P < .001) were associated with obtaining S1 IgG ≥ 300 BAUs/mL. Lower than normal numbers of B cells (<0.1 × 10 9 /L) decreased odds to obtain S1 IgG ≥ 300 BAUs/mL (OR, 0.25; CI, 0.15-0.42; P < .001). Higher than normal number of B cells (>0.5 × 10 9 /L) was also associated with decreased odds to obtain S1 IgG ≥ 300 BAUs/mL (OR, 0.35; CI, 0.18-0.67; P < .001), but this association was lost when patients with CLL were excluded (OR, 1.15; CI, 0.40-3.28; supplemental Table 6). Absolute CD3 and CD8 T-cell numbers were significantly associated with obtaining S1 IgG ≥ 300 BAUs/mL when tested per subcategory of parameters but not in the overall model. The use of rituximab (OR, 0.24; CI, 0.10-0.57; P = .001), the Bcl2 inhibitor venetoclax (OR, 0.11; CI, 0.019-0.63; P = .013), and CD19-directed CAR T-cell therapy (OR, 0.16; CI, 0.057-0.48; P = .001) was negatively associated with obtaining S1 IgG ≥ 300 BAUs/mL. This also applied to the number of immunosuppressants used (1-2 immunosuppressants: OR, 0.45; CI, 0.28-0.73; >2: OR, 0.25; CI, 0.10-0.64; P = .001)). The use of specific immunosuppressants, such as mycophenolic acid, calcineurin inhibitors, corticosteroids, or the jak2 inhibitor ruxolitinib, was not significantly associated with the odds to obtain S1 IgG ≥ 300 BAUs/mL whether analyzed in the overall model or per patient cohort (allogeneic HCT, cGVHD, and CLL on ibrutinib; supplemental Table 7). Lenalidomide or pomalidomide treatment and the use of TKI on the other hand were associated with higher odds to obtain S1 IgG ≥ 300 BAUs/mL (OR, 3.5; CI, 1.8-6.7; P < .001 and OR, 2.7; CI, 1.1-7.1; P = .038, respectively; Figure 4B ).

Multivariable analyses of factors associated with S1 IgG responses. (A) Variables significantly associated with S1 IgG ≥ 300 BAUs/mL as a dichotomous outcome tested in a multivariate model per subcategory of variables. (B) Significant variables of model 1 tested in an overall model.
Within cohorts, time after cessation of rituximab, after autologous HCT, or after allogeneic HCT was not significantly associated with the odds to obtain sufficient S1 IgG concentrations (lymphoma: P = .069; multiple myeloma: P = .98; allogeneic HCT: P = .272; supplemental Table 7). Nevertheless, a time interval after which sufficient S1 IgG concentrations were reached could be identified for each cohort. In patients with lymphoma, median S1 IgG ≥ 300 BAUs/mL were not reached before 8 months after cessation of rituximab therapy and after autologous HCT ( Figure 5A ), whereas in patients with multiple myeloma sufficient S1 IgG concentrations were obtained immediately after autologous HCT ( Figure 5B ). In allogeneic HCT recipients, a median S1 IgG ≥ 300 BAUs/mL was not reached before 4 to 6 months after transplantation ( Figure 5C ). In all groups, numbers of circulating B cells did not need to be normalized to obtain S1 IgG ≥ 300 BAUs/mL ( Figure 5 ).

IgG S1 concentration and B cell numbers related to time after end of rituximab or HCT. (A) Patients with lymphoma after autologous HCT or after rituximab. (B) Multiple myeloma after autologous HCT. (C) Allogeneic HCT. Left panels: S1 IgG concentration with dotted lines specifying seroconversion (S1 IgG > 10 BAUs/mL) and sufficient S1 IgG concentration (≥300 BAUs/mL). Right panels: B-cell number with dotted lines at upper and lower limit of normal.
At the time COVID-19 vaccines became available, vaccination guidelines in hematology patients under active treatment were conservative, owing to the paucity of vaccine response data and the many uncertainties regarding the immunocompetence of these patients. 5,6 Our study is unique in that it was designed to specifically include severely immunocompromised hematology patients during or shortly after treatment or HCT, for whom vaccinations are often deemed futile. Moreover, we performed a quantitative analysis of SARS-CoV-2 binding antibody concentrations, calibrated against the WHO standard, 7 , 10-13 , 15 to identify those patients who despite their immunodeficiency did obtain adequate antibody concentrations. In collaboration with a number of other prospective cohort studies on the immunogenicity of COVID-19 vaccination among immunocompromised and healthy individuals conducted in The Netherlands, we set an S1 IgG concentration of 300 BAUs/mL as the lower threshold of an adequate COVID-19 vaccine response. 9,11 , 13-16 It is interesting to note that a lower threshold of 264 BAUs/mL was very recently, in an independent British cohort, estimated to correspond to a vaccine efficacy against symptomatic COVID-19 of 80%. 7 Even though we included only patients considered to be severely immunocompromised, 70% demonstrated significant increases in antibody concentrations, and >50% obtained adequate antibody concentrations. In particular, patients with sickle cell disease, CML on TKI, AML, and high-risk MDS on or <12 months after high-dose chemotherapy, multiple myeloma on IMiD or daratumumab therapy, patients with untreated CLL and patients with cGVHD had higher odds to obtain adequate S1 IgG concentrations than anticipated. Serum S1 IgG correlated strongly with in vitro antibody-mediated virus neutralization, confirming data obtained from studies in healthy individuals, patients with kidney disease, and oncology patients. 11,13,17,18
In general, S1 IgG responses were higher in our study than reported previously. For example, seroconversion was reached in 83% of untreated patients with CLL, compared with 55% in a cohort of therapy-naive patients with CLL vaccinated with BNT162b2. 19 Apart from the differences in vaccination schedule and age, which was lower in our cohort (median age 64 vs 71 years), type of vaccine is a possible determining factor. All participants in our study received mRNA-1273, which induces higher antibody concentrations than other vaccines. 20-23 Whether this corresponds to better protection against severe COVID-19 remains to be determined.
Vaccination guidelines for autologous HCT recipients do not typically differentiate between patient groups. 5 Our data demonstrate, however, that vaccine immunogenicity after autologous HCT depends on the underlying hematologic condition and/or treatment. In patients with multiple myeloma, adequate antibody responses were reached immediately after HCT, whereas patients with lymphoma did not obtain adequate S1 IgG titers before at least 8 months after HCT, similar as observed in patients with B-NHL after cessation of rituximab-containing therapy. These differences are probably related to differences in the degree of B-cell depletion between cohorts, that was much less stringent in patients with multiple myeloma. Of note, our results demonstrate that also low numbers of B cells can produce adequate levels of antibodies. In multivariable analyses, IgG4 concentration and absolute B- and NK-cell numbers predicted S1 IgG responses. Not only lower-than-normal B-cell numbers were associated with blunted antibody responses, but also higher-than-normal B-cell numbers reduced odds to obtain sufficient S1 IgG concentrations. As this correlation was no longer significant when patients with CLL were excluded from the analysis, this is most likely related to CLL-associated reduced numbers of normal B cells and/or immune paralysis. 24 SARS-CoV-2 vaccination responses were better in patients using the immunomodulator lenalidomide, as demonstrated previously for pneumococcal vaccination, 25 and in patients with CML using TKI. The latter is in contrast to previous reports demonstrating reduced immunogenicity of pneumococcal vaccination during TKI therapy, 26 which may be related to the distinctive pathways of immunity induced by messenger RNA vaccines compared with T-cell independent polysaccharide vaccines. 27 Together these data suggest that concerted immune responses that may include the help of cytokine-producing NK cells yield the best antibody concentrations after vaccination. 27,28
Almost 50% of patients did not reach S1 IgG ≥ 300 BAUs/mL. Many of these patients nevertheless demonstrated a clear increase in antibody concentrations after each vaccination, suggesting that antibody responses can be further enhanced with one or more extra vaccinations. 29-31 Ideally, such vaccination schedules are guided by S1 IgG concentrations, and we are currently testing the feasibility of such an approach. Other important questions presently under investigation by us and others refer to the functionality of T and NK cells and the durability and functionality of antibody and B-cell recall responses, in particular, in patients with a reduced B-cell repertoire, patients with quantitatively or qualitatively insufficient T-cell help, and patients who are receiving daratumumab to deplete malignant plasma cells. Importantly, blunted antibody responses in rituximab-treated patients with rheumatology did not rule out the generation of T-cell immunity against SARS-CoV-2, 32,33 but true vaccine efficacy in B-cell–depleted patients remains to be determined. We did observe breakthrough infections after completion of the primary vaccination schedule, but none of our study participants died of COVID-19. Although this may seem encouraging, it is important to emphasize that our study was not powered to investigate vaccine effectiveness. Indeed, an English population-based study demonstrated persistently increased risk of COVID-related death for patients with hematologic conditions despite vaccination. 34 It is advised to consider SARS-CoV-2 monoclonal antibody therapy for patients with impaired humoral immunity who tested positive for SARS-CoV-2. 35,36 Specific recommendations may vary depending on the dominant variant of concern in a particular region (see, for up-to-date guidelines, eg, www.covid19treatmentguidelines.nih.gov/therapies/ ).
Taken together, we observed that the majority of severely immunocompromised hematology patients on or early after (immuno-)chemotherapy or HCT obtained adequate antibody concentrations after 2-dose mRNA-1273 vaccination. Clinical consequences of these findings are summarized in Table 2 . The most important message is that immunocompromised hematology patients should not be precluded from vaccination, even though full antibody-mediated protection cannot be guaranteed for all.
Implications of findings for COVID-19 vaccination guidelines
Conclusions are based on S1 IgG concentrations obtained 4 wk after the standard 2-dose mRNA-1273 vaccination schedule given 28 d apart. Other markers of immunogenicity, such as antigen-specific T-cell responses, are not taken into consideration here.
The authors extend their deepest gratitude to all patients, colleagues, students, and volunteers from participating institutes, The Netherlands Cancer Registry, and the National Institute for Public Health and the Environment, who made this study possible.
This study was financially supported by the Dutch Research Council (NWO/ZonMW, grant 10430072010009) and Amsterdam UMC.
Funding support for this article was provided by the Dutch Research Council (NWO / ZonMW) (10430072010009)
Contribution: S.Z., A.P.K., B.I.L.-W., A.G., C.E.R., I.S.N., and M.D.H. developed the study protocol, with contributions from N.Y.R.; S.H., R.S.v.B., G.d.H., M.S.B., N.J.E.H., D.M.d.R., J.v.M., J.C., N.A.K., D.W., S.S.W., E.M.M.v.L., H.J.B., S.T.-A., E.R.-K., Q.H., K.G., L.C., L.S., Y.M.d.H., B.T., I.M.J.K., E.d.P., W.A.D., M.D.E., T.G., R.C.N.P., S.R.J., E.v.D., M.P., J.A.B., J.H.B., G.S., I.S.N., M.D.H., T.v.M., P.G.N.J.M., J.A.v.D., A.E.C.B., and C.E.R. recruited and vaccinated patients, performed experiments, and collected data; S.H., B.I.L.-W., R.S.v.B., G.d.H., M.J.v.G., I.S.N., and M.D.H. analyzed and interpreted data and wrote the manuscript; and M.H.M.H., R.W.S., Q.H., S.Z., A.P.K., T.v.M., P.G.N.J.M., J.A.v.D., A.E.C.B., A.G., and C.E.R. contributed to the manuscript and approved its final version.
Conflict-of-interest disclosure: J.C. is consultant for Novartis and received research funding from Novartis, Merus, Takeda, Genentech, and BD Biosciences and royalties from Navigate and BD Biosciences. A.P.K. is consultant for Abbvie, Janssen, Astra Zeneca, BMS, Roche, LAVA and received speakers fees from Abbvie and research funding from Janssen, Abbvie, Astra Zeneca, BMS, Celgene and Roche. S.Z. received research funding from Takeda and Janssen and participated on advisory boards of Takeda, Janssen, BMS/Celgene, Sanofi, and Oncopeptides. T.v.M. is a consultant for Janssen and Kite and received research funding and honoraria from BMS/Celgene, Genentech, and Kite. P.G.N.J.M. received research funding from Astra Zenica and is consultant for GSK and BMS. I.S.N. is consultant for Janssen, BMS/Celgene, Amgen, and Sanofi. Amsterdam UMC (M.J.v.G. and R.W.S.) filed a patent application on SARS-CoV-2 monoclonal antibodies. None of these are conflicts of interest in the present study. The remaining authors declare no competing financial interests.
Correspondence: Mette D. Hazenberg, Department of Hematology, Amsterdam University Medical Center, location AMC, Meibergdreef 9, 1105 AZ Amsterdam, The Netherlands; e-mail: [email protected] .
Author notes
M.D.H. and I.S.N. contributed equally to this study.
Presented at the 63rd annual meeting of the American Society of Hematology, Atlanta, GA, 13 December 2021.
Deidentified individual participant data that underlie the reported results will be made available 3 months after publication for a period of 5 years after the publication date. Proposals for access should be sent to [email protected] .
The full-text version of this article contains a data supplement.
Supplemental data
- Previous Article
- Next Article
Email alerts
Affiliations.
- Current Issue
- Latest Articles
- Collections
- Community Conversations
- Blood Advance Talks
- Permissions
- Submit to Blood Advances
- Order Reprints
- Advertising in Blood Advances
American Society of Hematology
- 2021 L Street NW, Suite 900
- Washington, DC 20036
- TEL +1 202-776-0544
- FAX +1 202-776-0545
ASH Publications
- Blood Advances
- Blood Neoplasia
- Blood Vessels, Thrombosis & Hemostasis
- Hematology, ASH Education Program
- ASH Clinical News
- The Hematologist
- Publications
- Privacy Policy
- Cookie Policy
- Terms of Use
This Feature Is Available To Subscribers Only
Sign In or Create an Account

An official website of the United States government
The .gov means it’s official. Federal government websites often end in .gov or .mil. Before sharing sensitive information, make sure you’re on a federal government site.
The site is secure. The https:// ensures that you are connecting to the official website and that any information you provide is encrypted and transmitted securely.
- Publications
- Account settings
Preview improvements coming to the PMC website in October 2024. Learn More or Try it out now .
- Advanced Search
- Journal List
- BMJ - PMC COVID-19 Collection

Original research
Perspectives on the covid-19 vaccine uptake: a qualitative study of community members and health workers in zambia, cephas sialubanje.
1 School of Public Health, Levy Mwanawasa Medical University, Lusaka, Zambia
Nawa Mukumbuta
2 COVID-19 Advisory Centre for Local Authorities, Local Gover Government of Association of Zambia, Lusaka, Zambia
Mary Ng'andu
3 Health Sciences, Levy Mwanawasa Medical University, Lusaka, Zambia
Ernest Malangizo Sumani
Mpala nkonkomalimba.
4 Administrative unit, Local Government Association of Zambia, Lusaka, Zambia
Daniel EM Lyatumba
Alick mwale, francis mpiana, joseph makadani zulu, basil mweempwa.
5 Decentralisation for Development (D4D), Deutsche Gesellschaft für Internationale Zusammenarbeit (GIZ) GmbH /German Cooperation, Lusaka, Zambia
Denise Endres
Maurice mbolela, mpatanji namumba, wolff-christian peters, associated data.
bmjopen-2021-058028supp001.pdf
bmjopen-2021-058028supp002.pdf
bmjopen-2021-058028supp003.pdf
Data are available on reasonable request. Data are available on reasonable request from the corresponding author and with permission of the UNZABREC Institutional review board.
Since introduction of the programme in April 2021, COVID-19 vaccine uptake has been low at less than 20%. This study explored community members’ and health workers’ perspectives on the COVID-19 vaccine uptake and its influencing factors in Zambia.
Study design
A qualitative study employing focus group discussions (FGDs) and in-depth interviews (IDIs).
Study setting
Sixteen primary healthcare facilities selected from Lusaka, Copperbelt, Central and Southern provinces.
Participants
A total of 32 FGDs comprising local community members and 30 IDIs including health workers, traditional, religious and civic leaders (n=272). FGDs were separated based on age (youth and adults), sex (male and female) and place of residence (urban and rural).
Both FGD and IDI participants agreed that vaccine uptake was low. Limited knowledge, access to information, myths and misconceptions, negative attitude, low-risk perception and supply in remote areas affected vaccine uptake. Overall, FGD participants expressed limited knowledge about the COVID-19 vaccine compared with health workers. Further, FGD participants from urban sites were more aware about the vaccine than those from rural areas. Health workers perceived the vaccine to be beneficial; the benefits included prevention of infection and limiting the severity of the disease. Moreover, FGD participants from urban sites expressed a negative attitude towards the vaccine. They believed the vaccine conferred no benefits. By contrast, participants from rural communities had mixed views; they needed more information about the vaccine benefits. Participants’ attitude seems to have been influenced by personal or family experience with the COVID-19 disease or vaccination; those who had experienced the disease had a more positive attitude. In contrast, most young people believed they were not at risk of the COVID-19 disease. Misinformation from social media influenced their attitude.
These results provide starting points for future policies and interventions for increasing COVID-19 vaccine uptake.
STRENGTHS AND LIMITATIONS OF THIS STUDY
- Purposive sampling of participants comprising health workers and community members with different demographic and socioeconomic characteristics (sex: male and female; age; place and province of residence: urban and rural settings) allows for comparing and contrasting of participant views, which in turn provides an in-depth understanding of the subject under investigation.
- Use of different data collection techniques (focus group discussions and in-depth interviews) as well as data sources allow for triangulation of findings and increases internal validity of the study.
- Training data collectors and use of an inductive approach to data analysis increase the internal validity of study findings.
- Conducting the study at the beginning of the COVID-19 national mass vaccination when the programme was still new in the country may have affected the views of the participants.
- Use of the qualitative design affects external validity and limits generalisation of the study findings.
Introduction
SARS-CoV-2 has spread to most parts of the world, including Zambia. 1 2 As at the end of August, 2022, a total of 332, 058 cases and 4016 deaths had been reported in the country. 3 To prevent further spread of the virus and increased mortality in the country, the Zambian government enacted the public health statutory instrument number 22, 4 5 which instituted preventive and control measures—restricting social gatherings (ie, work places, church services, weddings, kitchen parties, casinos, funerals), local and international travel, and closing public institutions such as schools and markets. 6 In addition, the Zambian government implemented a national mass vaccination campaign 7 following approval of the COVID-19 vaccines in developed countries. 8–10 Initially, the COVID-19 national vaccination programme targeted the health workers and persons aged above 65 years. 10 The criteria were later revised to include all persons aged 18 years and above. 10 A second revision was made to include children aged 10 years and above. Three types of COVID-19 vaccines have been administered: Oxford/AstraZeneca, Sinopharm, John and Johnson and Pfizer vaccines. Access to the COVID-19 vaccine is voluntary and free in the country; people do not need to pay anything. 11 To be protected, one needs to receive two doses of the Oxford/AstraZeneca vaccine−8 weeks apart. On the contrary, only one dose of Johnson & Johnson vaccine is needed to be fully immunised. 12 13
Over the centuries, vaccines have been shown to be an effective way to combat outbreaks and the only efficient and reliable method for disease prevention. 14 15 COVID-19 vaccines—with an efficacy ranging from 70% to 95%, have been shown to provide protection against the virus 15 by preventing its spread in the community, mitigating the severity of the disease and reducing mortality among the infected people. 16 17 Studies and ongoing clinical trials 18–22 have shown that COVID-19 vaccines offer the best means to control the ongoing pandemic. They are effective in preventing a wide range of COVID-19-related outcomes, reduce symptomatic cases, hospitalisation, disease severity and death among the infected individuals. Nevertheless, national reports show that the vaccine uptake has been low in the country. At the end of August 2022, a total of 5 576 827 have been fully vaccinated with the AstraZeneca, Sinopharm and Johnson & Johnson vaccine since the commencement of the programme in April 2021. This represents a national vaccine coverage of 52.9% of the eligible population. 23
Limited supply and vaccine hesitance—the delay in acceptance or refusal of vaccination despite availability of vaccination services 24 —have been attributed to the low vaccine coverage in the country. Vaccine hesitance has been reported both in Zambia and other countries, and has been shown to be an important obstacle to the fight against COVID-19. 25–27 For example, in their recently published article, Mutombo et al 28 observed that the gradual effort to distribute COVID-19 vaccines to low-income and middle-income countries (LMICs) is threatened by the ubiquitous vaccine hesitancy, especially in Africa, where it undermines efforts to fight the COVID-19 pandemic. A qualitative study 29 using focus group discussions (FGDs) with mothers who brought their children for measles vaccination in southern Zambia a few months before the COVID-19 national vaccination programme was implemented, reported that, although parents were willing to allow their children to receive the vaccine, majority expressed substantial uncertainty and hesitancy about receiving the vaccine themselves. The study also revealed beliefs around COVID-19 risk and severity, as well as vaccine safety and effectiveness affected the participants’ intention to be vaccinated. However, vaccine hesitance is not peculiar to the COVID-19 vaccine. For example, Garcia and others 30 reported low levels of Cholera vaccine acceptance among community members and health workers in the slums of Lusaka, Zambia. The authors also showed that religious beliefs and distrust towards western medicine, fear of injections and adverse events, low perceived need for immunisation and limited understanding of how vaccines work were important factors affecting acceptance of the cholera vaccine.
Although these studies provided important insights on vaccine hesitance and its contributing factors, most were conducted outside the country. The one conducted in Zambia explored mothers’ intentions to receive the COVID-19 vaccine and not the actual behaviour. Moreover, the study was conducted before the COVID-19 was implemented. The other Zambian study focused on the Cholera vaccine. It thus, not clear how the Zambian population perceive the COVID-19 vaccination programme. Available evidence suggests that vaccine hesitance is complex and context specific, varying across time, place and vaccines. A study is therefore needed to explore people’s perspectives and attitude towards COVID-19 vaccine uptake in the country. The aim of this study, therefore, was to explore community members’ and health workers’ perspectives on the COVID-19 vaccine uptake and the reasons that affect its uptake in Zambia. This information can provide insight to the COVID-19 vaccine hesitancy and the contributing factors, which in turn, can inform design of interventions to increase vaccine intentions and uptake in the general population. To date, no such study has been conducted in Zambia.
This study employed a qualitative design comprising FGDs and in-depth interviews (IDIs) as the data collection techniques. FGDs have been used in public health research for over three decades now. 31 They aim to explore participants’ experiences, beliefs and attitudes towards a target behaviour, by using group processes to stimulate responses and gain insights through participants’ exchanging views, questioning and challenging one another. 32 IDIs enable the researcher to understand participants' lived experiences through their own words and perspectives. 32 33 Use of both FGDs and IDIs allows for in-depth exploration and understanding of various aspects regarding the subject under investigation. The approach also allows for triangulation and corroboration of the FGD and IDI findings, which, in turn, increases the internal validity of the study. 34 35
The study was conducted in 16 primary health facilities and their catchment communities—3 from Lusaka city, 2 each from Chongwe, Ndola, Masaiti, Kabwe, Chibombo and Kafue, 1 one from Mazabuka districts. Selection of health facilities was done in consultation with various stakeholders including health staff working in the COVID-19 vaccination programme and health promotion departments at the provincial and district health offices, Zambia National Public Health Institute and Ministry of Health (MoH) headquarters. To be selected, health facilities needed to have been providing COVID-19 vaccination services as well as other COVID-19 prevention, control and care services including screening, contact tracing, isolation and treatment facilities. In addition, health facilities needed to be accessible by road during the study period. Health facilities from Lusaka and Copperbelt provinces were selected because they were COVID-19 epi-centres due to huge populations and commercial activities; Central province is a transit area for traffic from Tanzania and the Democratic Republic of Congo in the north and north-east, respectively; Southern province is a transit region for travellers from the Southern region (South Africa, Zimbabwe, Namibia and Botswana)—all of which reported high numbers of COVID-19 cases. 36 37
From each health facility one community was selected, in consultation with the health facility managers and local community leaders. Efforts were made to ensure an equable distribution of urban and rural communities in the study. To be included in the study, communities needed to be accessible with passable roads, and within 2 hours drive from the health facility. In addition, the local community leaders needed to authorise the team to conduct the study in the community.

Participants and sampling technique
Fgd participants.
A total of 32 FGD (n=242) were conducted with community members. Each FGD comprised between 6 and 10 participants (n=242). To gain insight into the differences and similarities in the participants’ views, FGDs were separated based on age, sex and place of residence. Half of the FGDs (50%) were held in urban communities and the other half in rural settings. To compare and contrast the views based on sex, separate FGDs were held with male and female participants. In addition, a total of eight FGDs were separately held with the youth to gain insight into their perspectives on the subject. Efforts were made to balance the eight FGDs on sex (four male and four female) and place of residence. Initially, a total of 40 FGDs (10 per province) were planned by the research team. However, after conducting 10 FGDs in Lusaka province (men=4, women=4, youth=2), the point of saturation was achieved (ie, no further substantial information was obtained from the participants). At this point, the research team decided to reduce the number of FGDs and conduct only a few more FGDs in the other districts. At the end, 24 FGDs were conducted with the adults (males=12, females=12) and 8 with the youth (18–24 years) (see table 1 ).
Distribution of FGDs
FGDs, focus group discussions.
Using a purposive sampling technique, community health workers assisted by local community leaders (traditional and civic) conducted the recruitment of FGD participants from the local communities. Purposive sampling allows for selection of participants with similar experiences regarding the health behaviour under investigation (ie, perspective and attitude towards COVID-19 vaccine), while, at the same time, allowing for recruitment of participants with different demographic and socioeconomic characteristics—such as age, sex and place of residence and occupation. This, in turn, helps provide insight into the similarities and differences in the participants’ experiences with regard to the health problem under investigation.
Recruitment of the participants was done in multiple steps. First, the research field supervisor together with the district health promotion officer held meetings with the local health facility staff, community health workers and community leaders to inform them about the study and its objectives, and to identify the communities where the FGD participants would be recruited from. Following this meeting, community health workers and local leaders called for a meeting to inform the community members about the study. Community members willing to participate in the study were asked to register with the community health workers and leave their details (place of residence and phone number). A few days after the meeting, the community health workers contacted the potential participants to provide more details about the study and assess their suitability to participate in the study. The assessment was based on the participant eligibility criteria described below. Next, the community health workers compiled a comprehensive list of community members who were eligible to participate in the FGDs and made arrangements for the selected participants to come for the actual discussion to an agreed on place (usually the headman’s place, school or church) on the set time.
In-depth Interviews
To gain insight into the views of the local health staff and community leaders, a total of 30 IDIs (n=30) were conducted. IDI participants were recruited from their places of work and homes. Health promotion officers helped recruit the health staff; community health workers recruited the community leaders. Following identification of the potential participants, the recruiting staff compiled a comprehensive list of IDI participants and contacted them before the day of the interview. Health staff were selected from the district health offices and local health facilities and comprised public health officers, clinicians, nurses and community health workers (n=16). Community leaders were selected from the local communities and included traditional, religious and civic leaders (n=10). In addition, staff (n=4) from non-governmental organisations (NGOs) working with the MoH in the provision of COVID-19 services at the local district level were included in the study.
Eligibility criteria
To participate in both the FGDs and IDIs, participants needed to be:
- Aged 18 years and above.
- Residing or working in the study area for not less than 3 months.
- Health workers involved in the COVID-19 vaccination programme from the selected primary health facilities.
Prisoners and mentally ill people were not included in the study.
Before each FGD/ IDI, written informed consent was obtained from each participant; those who could not read or write were asked to mark with an ‘X’. To make it easy for the study participants to understand, the consent form ( online supplemental material 1 ) was translated into the local language (Bemba, Nyanja and Tonga). After signing the consent form ( online supplemental material 2 ), each FGD/IDI participant was asked to complete a short demographic questionnaire ( online supplemental material 3 ). To make it easy for those who could not read or write, research assistants read the consent form and the short questionnaire and filled it in for them.
Supplementary data
Training of data collectors.
Research assistants were a group of six (three male and three female) final year Master of Public Health (MPH) students recruited from the Schools of Public Health at the University of Zambia (n=3) and Levy Mwanawasa Medical University (n=3) in Lusaka. MPH students were selected because they were skilled and experienced in qualitative research methods: facilitating FGDs and conducting IDIs. To avoid information concealment during FGDs and IDIs, efforts were made to select students who spoke both English and one or two of the local languages, Nyanja, Bemba or Tonga. Before commencement of the data collection process, research assistants underwent a 5-day training in FDG facilitation and interviewing techniques: 3 days of theory and 2 days of practical fieldwork. Topics covered during the 3 days theoretical training included: (1) basic principles of qualitative research, (2) objectives of the study, (3) FGD facilitation techniques, (4) interviewing techniques, (5) research ethics and informed consent in human subjects’ research and (6) FGD and IDI interview guide. Phase 2 of the study was a practical exercise in FGD facilitation techniques, conducting interviews and obtaining informed consent. On the first day of the pactical phase, the research assistants worked in pairs and took turns to facilitate an FGD with their fellow trainees. They also took turns interviewing each other. At the end of eachFGD/IDI, research assistants were asked to provide feedback on each other’s performance. At the end, the trainer also provided feedback and guidance on group dynamics, participant interaction, body language, avoiding conflict and managing it when it arises. On the second day, the team was taken into the nearby neighbourhood to facilitate FGDs with community members and conduct IDIs with health staff and community leaders. The FGDs and IDIs were transcribed and analysed, after which the FGD and interview guides were revised based on the analysed data and feedback from the data collectors.
Data collection
Each FGD was facilitated by a pair of research assistants: one facilitated the discussion and took notes, the other one was in charge of the digital voice recorder. As they facilitated the FDGs, research assistants ensured that each participant was given an opportunity to speak; they also tactfully controlled the dominant individuals and prompted the passive ones to speak. They also ensured that the discussion flowed smoothly among the participants without turning it into an interview, personal attack or conflict. Where necessary, the facilitator asked for elaboration, clarification or probed for detail. Interviews were also conducted by a pair of research assistants: one conducted the interview and took notes; the other recorded the interview with a digital audio recorder. IDIs were conducted at the participant’s preferred place including the office or home. On average, each FGD lasted between 1 hour and 1.5hrours. IDIs lasted between 30 and 45 min. To ensure quality in data collection, a digital voice recorder was used for both IDIs and FDGs.
Data collection tools
FGDs/IDIs were conducted using a paper-based, FGD/interview guide ( online supplemental material 2 ). The FGD/interview guide had predetermined themes, including: (1) perspectives on acceptance of the COVID-19 vaccine and (2) factors affecting vaccine uptake. The second theme had several probes including knowledge and information sources, and attitude towards COVID-19 vaccine. The same interview guide was used for both the FGDs and IDIs, with minor elaboration for the IDIs to elicit some detail. During the FGDs, the focus was on the community perspectives; for IDIs the focus was more on the participant’s perspectives regarding the issues under investigation. In addition, a short questionnaire ( online supplemental material 3 ) was prepared to collect FGD and KII participants’ demographic, socioeconomic and vaccination data. To ensure internal validity, the FGD/interview guide went through a rigorous development process. First, the principal investigator with vast experience in qualitative research and familiar with the subject, drafted the initial version. The themes in the FGD/interview guide were adapted from various sources, including review of the available literature on COVID-19 vaccine and the researchers’ experience in qualitative research methods. Next, the document was shared with the research team members for their comments and feedback. The document was revised based on the research team’s comments. Two independent bilingual experts translated the document into the local languages, Bemba, Tonga and Nyanga. The translated document was pretested in an urban slum of Lusaka during the research assistant training, after which both the KII and FGD recordings were transcribed and analysed. The tool was then revised based on the pre-test findings and feedback from the data collectors.
The data management and analysis
Audiorecordings from the FGDs and IDIs were transcribed and translated into English by four independent people who never participated in the data collection, and were proficient in English and the local language. To check for accuracy, 10% of the transcripts were back-translated into the local language. NVivo V.11 MAC was used for coding and analysis. To make it easy to compare differences and similarities in the participants’ perspectives by different attributes, a separate codebook was created for FGD and IDI data using a framework based on the FGD/interview guide. An inductive approach to data analysis was used, ensuring that subthemes were derived from the predetermined themes and grouping all similar statements concerning particular themes. In order to determine similarities and differences in the responses, findings for the FGDs and IDIs were analysed separately, according to the FGD participants: age (adults vs youth), sex (male vs female) and place of residence (urban vs rural). Summary and descriptive statistics were computed for FGD and IDI participants' demographic characteristics using SPSS V.25 (IBM SPSS Statistics 25)
Quality assurance and control
The team ensured the quality of data collection by: (A) recruiting skilled and experienced data collectors who were trained for 5 days on the theoretical and practical aspects of the study, (B) ensuring that data collectors worked in pairs, (C) using an interview guide (translated into the local language), (D) using a digital voice recorder and taking extensive notes during the FGDs and interviews, (E) by comparing notes and voice recordings each day after the interviews, (F) using experienced and independent staff to transcribe the recordings from the FGDs and KIIs.
Patient and public involvement
Study participants and the public were not directly involved in the design of the study. Rather, the study was designed in response to the call for consultancy for a research proposal on COVID-19 vaccine issued by the COVID-19 Centre, funded by GIZ in Lusaka. However, selection of the primary health facilities, communities and study participants was done in collaboration with stakeholders from the provincial, district and primary health facility and community levels. First, prefield meetings were held with the provincial and district managers to select primary healthcare facilities and local communities to be included in the study. Next, local district managers selected the primary health facilities to be included in the study. In turn, primary healthcare facilities together with the local community leaders recruited the FGD participants and made arrangements for them to come for the actual discussion; they also contacted and prepared a comprehensive list of IDI participants. Finally, a report was written and shared with the funding organisation, GIZ and the COVID-19 centre for dissemination of study findings.
Demographics
Our sample comprised a total of 272 respondents (FGD=242 and IDIs=30). The majority (51.5%) of the participants were female, with a mean age just above 34.04 years and between 2 and 3 children. Almost half (47.1%) of the participants were married. Most participants (55.2%) had secondary school education, 18% had tertiary level education and 1.5% had never attended school. Majority (62.1%) of the participants had an average income of less than K500 per month. Most of the participants (69.5%) mentioned that they were aware about COVID-19, and 52.9% reported that the COVID-19 vaccine was beneficial. Less than 1/10th (9.9%) of the respondents were vaccinated (see table 2 ).
Demographic characteristics of the respondents (n=272)
Theme 1: perspectives on acceptance of the COVID-19 vaccine
Analysis of the findings from the short demographic questionnaire administered to the respondents before each FGD and IDI showed that less than 1/10th (9.9%) of the total sample (FGD and IDI participants) had received the vaccine ( table 2 ). Out of the 27 (9.9%) that reported being vaccinated, 18 (66.7%) were health workers. Our analysis of IDIs also confirmed that most health workers and participants from the NGOs had a positive attitude towards the COVID-19 vaccine and were willing to be vaccinated. Both the community leaders and participants from the NGOs confirmed that they had accepted the vaccine and that many people were willing to be vaccinated. They clarified that the low vaccine coverage reported, especially in rural areas, was a result of the limited access, low supply and stock-out of the vaccine. They mentioned that the vaccine was mainly available and administered in the urban health facilities. Those that lived in remote areas, far from the health facilities, had difficulties accessing the vaccine.
The vaccine has been accepted…. because people have been vaccinated; if they had not accepted the vaccine, they wouldn't have been vaccinated ( IDI informant, health worker, Ndola district ). They aren’t so many people that have been vaccinated. It is because the people vaccinating are rarely seen here ( Community leader, IDI participant, Pemba district ). For those who live in far-flung places, we don’t know if they get vaccinated. I think it would be best to ask them ( Community leader, IDI participant, Mazabuka district )
Most adult participants (both male and female) confirmed that they had not been vaccinated. However, most expressed willingness to be vaccinated. Especially, participants from the rural sites mentioned that many people would accept the vaccine if they had adequate information about its benefits and if it were made available in the health facilities. Our analysis showed no much difference between male and female FGD participants with regard to their attitude towards the COVID-19 vaccine and their intention to be vaccinated.
We can accept the vaccine, but we need sensitisation because even when we were going to school, our parents would tell us whether to accept the vaccine or not ( FGD participant, Lusaka ).
In contrast, analysis of FGD findings showed a striking difference in perspectives between the youth and adult participants. Most youth participants (from both the urban and rural areas) believed that the vaccine was not beneficial and confirmed that most young people had not accepted it.
We have not accepted the vaccine because we don’t know how it’s going to affect the life of someone in future. In short, we don’t know what the life span of people will be. This is the reason why we have not accepted it in our communities ( Youth FGD participant, Kabwe district ).
Theme 2: factors affecting acceptance of the COVID-19 vaccine
Our analysis of both FGD and IDI data showed various factors contributing to the low acceptance of the vaccine among study participant including lack of knowledge and information, myths and misconceptions, and negative attitude towards the vaccine. These factors are presented below.
Knowledge about COVID-19 vaccines
Overall, IDI participants (ie, health workers and participants from the NGOs) expressed better knowledge about COVID-19 vaccine than the FGD participants. Health workers and participants from NGOs knew the types of the COVID-19 vaccines, their mode of administration, benefits and side effects. They also knew about the COVID-19 national vaccination programme. Although most community members (both IDI and FGD participants) perceived the vaccines to be beneficial, majority lacked information about the vaccine-–the various types, mode and frequency of administration. They explained that many would accept the vaccine if they had adequate information. Limited access to information, especially in rural areas, was cited as the main reason for the low acceptance of the vaccine. Community leaders and health workers were unanimous on the information gaps in their communities.
People don't have the truth about the vaccine. The health team should come to educate us on how the vaccine works. They should come to communities, gather people and teach them about the COVID-19 vaccine ( Female FGD participant, Masaiti district )
A contrast was noted among the FGD participants with regard to their knowledge about the vaccine. In general, participants from urban areas expressed better knowledge than those from rural communities. Differences were also noted between the adults and youth FGD participants. Although most youth confessed that they did not know much about the vaccines, they explained that young people had heard about the vaccine, especially those from urban areas.
We know about the COVID-vaccine…most of us youth have heard about COVID-19 and know about the new vaccine ( Youth FGD Participant, Chainda, Lusaka city )
Limited access to information about the COVID-19 vaccine
Overall, our findings show that there was limited access to correct and quality information about the COVID-19 vaccine among most community members who participated in both the FGDs and IDIs. Limited access to information was mentioned as a major reason for the low vaccine acceptance among the participants. Participants from urban settings had better access to information than those from rural areas. Both the IDI and FGD participants confirmed that mass media (radio, televion (TV)), internet and social media were the main sources of information on the COVID-19 vaccine.
A contrast was observed in perspectives on the access to information between the FGD participants from urban and rural settings. Most adult FGD participants from urban sites confirmed that they had access to the major sources of information—media (radio and TV). However, they complained that they could not understand most messages on TV and radio because they were in English. They observed that broadcasting the same messages in local languages would greatly help increase community awareness about the vaccine.
Many people get the information from the radio and TV. They listen to the radio and TV to hear what the Minister is saying. ( Community leader, IDI participant, Chongwe district )
Participants from rural sites did not perceive the media (TV and radio) and internet to be the main sources of COVID-19 information. Poor TV and radio signal reception limited their access to information. Rather, they received information from the health staff, schools, churches, community health workers and community leaders (during community meetings). Health staff disseminated the information about the COVID-19 vaccine when people visited health facilities for various health needs. Community health workers shared the information during community meetings; community members, in turn, would share the information with their families and social networks.
Many people don’t watch TV here….they try to listen to the radio… They have TVs but they can’t see anything….the signal is poor. Government needs to improve TV and radio signal here ( Health worke, IDI participant, Pemba district )
Although community health workers played an important role in disseminating information about the COVID-19 vaccine in the community, most IDI and FGD participants (mainly community leaders and health staff) expressed concerns about the accuracy of the information. They complained that most community health workers did not have adequate knowledge about the vaccine and that, in some instances, the information was incorrect and distorted. As a result, most people did not trust the information they received from the community health workers. They suggested that people in their communities needed more sensitisation and education about the COVID-19 vaccine. They bemoaned that the local health facility staff did not do much to disseminate the information about the COVID-19 vaccine in their communities. Asked on the kind of information their communities needed, most community health workers and leaders mentioned information on the vaccine benefits, safety and the associated risks or side effects. They believed that accurate and adequate information would help the community members make an informed decision about taking the vaccine.
People in this area know nothing about the vaccine because they have never been sensitised. We need to be told what we can do so that we have an idea, but the way it is at the moment, we don't have any idea ( Community leaders, IDI participant, Pemba district ) We just hear from others in the community, because here most of the things we just hear them from these health workers when they pass and tell us, so we also believe what they tell us ( Female FGD participant, Masaiti district ).
In contrast, most young people, especially from urban sites, cited the internet and social media accessed through their phones as their main sources of information for COVID-19-related matters including the vaccine. However, poor internet and mobile phone signals in rural and remote areas made it difficult for most young people to access information.
Most of us use our phones to get information….we get everything from social media on our phones ( Youth FGD participant, Ndola city )
Myths and misconceptions about the COVID-19 vaccine
Our findings elicited many myths and misconceptions about the COVID-19 vaccine among both the IDI and FGD participants. Especially FGD participants were unanimous on the existence of various myths and misconceptions concerning the vaccine. These myths and misconceptions had a negative influence on people’s attitude towards the COVID-19 vaccine and seem to be some of the most important reasons for the high vaccine hesitance.
One of the myths held among most FGD participants (especially in urban settings) was that western countries brought the vaccine in order to eliminate the African population. According to them, westerners brought the vaccine because they wanted to collect people’s blood and kill them. They were concerned why certain vaccines given to the Africans had been rejected in Western countries.
There is a rumour that people in our community are spreading that the medicine [vaccine] was made to kill us Africans because we are too many. So even as we accept that this must be true ( Community leader, IDI participant, Ndola ).
The other strongly held belief by both male and female FGD participants from urban and rural sites (but not the youth) was that the vaccine was brought into the country for political reasons. They explained that politicians had gone into some contractual agreement with western countries to administer vaccines to their people in exchange for money. The money would then be used for political campaigns since it was a presidential and parliamentary election year (2021) in the country.
Some people are saying that they have brought the vaccine in an election period because they want them [community members] to die after giving them the injection so that they are sacrificed ( Female FGD participant, Lusaka )
The other firmly held belief (especially among rural participants) seemed to be influenced by participants’ religious background or inclination. They explained that people believed that the COVID-19 vaccine was part of the mark of the beast (666) mentioned in the Bible (see Revelation 13) and that those who receive the vaccine are initiated into the ritual.
Some people say this is 666, that’s what I heard others say. So that’s why we are scared, because we think that they will initiate us into the 666 rituals ( Female FGD participant, Kabwe ).
Other beliefs seem to be influenced by health reasons. For example, both the FGD and IDI participants from rural and urban settings explained that people in their communities believed that the vaccine is a slow poison which health staff introduced into the body. They believed that the vaccine dries up and makes the blood clot and that one will die after several months or years. Some participants also believed that after receiving the vaccine, one would start fitting and die immediately after being vaccinated; those who survived would only live for a few years afterwards.
Some say when you get vaccinated, you will just live for a few years, and then you get sick and die; that is why we are scared of getting vaccinated ( Traditional leader, IDI participant, Mazabuka district ).
Attitude towards the COVID-19 vaccine
Overall, our analysis of the data from the short demographic questionnaire showed that half (52.9%) of participants (both the FGD and IDI participants) perceived the vaccine to be beneficial ( table 2 ). However, analysis of FGD and IDI data shows that participants expressed different types of attitude towards the COVID-19 vaccine: positive, negative and ambivalent. The detailed findings on these attitudes are presented below.
Most health workers and some FGD participants from both urban and rural settings expressed a positive attitude towards the COVID-19 vaccine and perceived it to be beneficial. Perceived benefits were that the vaccine confers protection against the coronavirus infection among the vaccinated individuals. They also believed that the vaccine reduces the chances of a vaccinated individual to transmit the virus to other people. The other cited benefit was that the vaccine reduces the risk of developing severe disease. If one got infected, the disease would not be as severe as it is among those who are not vaccinated.
Most FGD participants expressed a negative attitude towards the COVID-19 vaccine. Interestingly, our findings did not show differences in attitude between male and female participants. Rather, rampant myths and misconceptions about the COVID-19 vaccine and personal or family’s previous experience with the COVID-19 disease or vaccination seemed to have influenced the participants’ attitude. In general, individuals or families who had not experienced the disease or seen someone suffer or die from COVID-19 disease expressed a negative attitude. They believed that the vaccine was not beneficial. Further, lack of information (especially in rural areas) and wide spread misinformation about the COVID-19 vaccine—such as exaggeration of the vaccine side effects—seemed to influence participants’ attitude towards the COVID-19 vaccine.
I know the benefits are building our immunity and we don’t get a chance to catch COVID-19, though people are saying even those that got the jab have tested positive, they don't have severe disease (IDI participant, Health worker, Ndola district).
Moreover, cultural beliefs and stigma about COVID-19 seem to have affected many people’s attitude and prevented them from accepting the vaccine. Especially health staff explained that some people did not believe in the existence of COVID-19. They cited examples of communities where a family member would suffer and die from COVID-19, but relatives would hide the information and mention another disease, such as asthma, as the cause of death. Because of denial and low-risk perception, such people refused to take the vaccine.
Lack of confidence in the health workers (who came from outside their communities) was perceived as an important factor influencing participants’ attitude towards the vaccine, especially among the community leaders and FGD participants from rural communities. They argued that people in their communities would only be convinced to take the vaccine if the health workers from their local communities administered the vaccine.
The people to vaccinate us must be from our community; otherwise, when an outsider comes to vaccinate us, we will be sceptical because we don't know them ( Village headman/IDI participant, Chongwe district )
In addition, most participants from rural towns and communities expressed an ambivalent attitude towards the vaccine; they were not sure about the benefits of receiving the vaccine. They argued that they did not know the vaccine benefits because they had not seen anyone take it. They explained that they would only believe in the vaccine benefits if someone or a group of people who had taken the vaccine went to explain how they felt after receiving it.
We do not know the truth, and we are scared, that is why we don't go for the vaccine injection. We have been told that the vaccine injection is harmful to consumers ( Male FGD participant, Masait district ). What we are saying is that they should bring us someone who has been vaccinated so that they tell us about the goodness of being vaccinated ( Male FGD participant, Chongwe district) We don't know how these things came, we are scared that we may die, and we can also be infected with other diseases. We don't see people who have been vaccinated, to tell us how they feel ( Civic leader, IDI participant, Masaiti district).
In general, young FGD participants from both urban and rural communities had a negative attitude towards the COVID-19 vaccine. Low-risk perception seemed to influence their attitude towards the vaccine. They believed that they were not at risk of getting the infection and that those who got infected would have mild or no symptoms at all. They also believed that the vaccine was not beneficial. Access to the internet and use of social media among the young people (especially from the urban communities with good internet connectivity) seems to have exposed them to incorrect information regarding the benefits and side effects of the vaccine. This, in turn, influenced their attitude. In addition, poor mobile phone signals, TV and radio reception in rural areas made it difficult for most young people to access information about the COVID-19 vaccine.
The aim of this study was to explore community members’ and health workers’ perspectives on the COVID-19 vaccine and the reasons that affect its uptake in Zambia. Overall, our findings showed low vaccine uptake among the participants. Several factors including limited knowledge, access to information, myths and misconceptions, negative attitude towards the vaccine and low-risk perception about the COVID-19 disease contributed to vaccine hesitance among the participants.
Our finding corroborates previous studies from LMICs and elsewhere which reported vaccine hesitance among health staff and community members. For example, a study conducted in Zambia 29 reported substantial uncertainty and hesitancy about receiving the vaccine among parents, despite expressing high intentions to have their children receive the COVID-19 vaccine. Similar findings were reported by Botwe et al 38 in Ghana who reported a vaccine hesitance of 44% among the health staff. These findings are also consistent with those by Baniak et al 39 who reported vaccine hesitance among nursing staff in the USA. The authors concluded that, despite the increase in vaccine uptake during the active vaccine rollout, there was still widespread and sustained hesitancy and unwillingness to take the vaccine. Other authors, Wong et al 40 and Luk et al 41 in Hong Kong also reported a low intention to vaccinate. They concluded that vaccine hesitance was a major challenge to effective programming and implementation. Thus, formulation and implementation of evidence-based vaccination strategies focusing on increasing the intention to take the vaccine has a potential to mitigate vaccine hesitance
Limited knowledge about the COVID-19 vaccine, its benefits and potential harms, was found to be one of the important barriers to effective vaccine uptake. The media (TV, radio and internet) play an important role in informing people about the vaccine. However, poor TV and radio signal reception in rural and remote areas limit access people’s access to these important sources of information. This explains the stark contrast in the levels of knowledge about the COVID-19 vaccine between participants from rural and urban communities. Moreover, our findings suggest that social media accessed through the internet on mobile phones is a major source of information among young people. However, poor internet and mobile phone signals in rural areas make it difficult for young people to access information. This finding is consistent with previous studies 42–46 which reported low knowledge levels concerning the COVID-19 vaccine. Interestingly, these studies showed that knowledge about the vaccine was positively correlated with one’s vaccine uptake. This finding suggests that information is an important factor influencing vaccine acceptance, and that lack of information affects peoples' willingness to take the vaccine. This result is consistent with the theory of reasoned action which highlights the importance of background factors such as knowledge and access to information in influencing people’s intention to adopt a health behaviour such as COVID-19 vaccination. 47–49 Public health interventions aiming at mitigating vaccine hesitancy and increasing vaccine uptake could benefit from focusing on knowledge and access to information about the COVID-19 vaccine, its benefits and safety.
Widespread myths and misconceptions about the reality of the COVID-19 disease and the benefits of the vaccine appear to be an important factor contributing to vaccine hesitance among our sample. These myths and misconceptions seem to be more rampant in rural communities where there is limited or no access to accurate information about the benefits and safety of the vaccine. For example, due to limited access to accurate information, many people in rural communities depend on the information from health workers and traditional leaders. Our findings suggest that such information, though important, is either inadequate or inaccurate with a potential to be misinterpreted. When people discover that such information is inaccurate untrustworthy, they seek alternative sources such as social media−which may also be misleading, resulting in the emergency of conspiracy and rampant myths and misconceptions. 49 However, especially in urban areas the situation is different; most myths and misconceptions seem to be influenced by the incorrect information spread by social media users, especially young people, with ready access to the phone and internet. For example, many participants (both FGDs and IDIs) believed that the vaccine is a poison: it dries up one’s blood, causes it to clot and eventually kills the victim. Our findings suggest that these strongly held beliefs have a negative influence on people’s intention to take the vaccine. These findings corroborate those reported elsewhere 50–53 regarding the importance of social media in propagating myths and misconceptions about the vaccine. These findings are also consistent with previous studies, for example, Bertin et al , 54 which reported that myths and misconceptions do not only instil fear among the people, but also influence them not to take the vaccine. Public health interventions can benefit from provision of correct and accessible information to prevent and address myths and misconceptions which negatively influence people’s perspectives and adoption of health behaviour, such as vaccine uptake. Thus, increasing access to correct information in the community has the potential to prevent and address the widespread myths and misconceptions about the vaccine and help mitigate vaccine hesitance. 55
Our findings suggest that attitude towards the COVID-19 vaccine has an important influence on the intention to take the vaccine. Although half of the participants perceived the COVID-19 vaccine to be beneficial, most had mixed attitudes towards the vaccine: positive, negative and ambivalent. Participants’ attitude seems to have been influenced by various factors including place of residence, age, access to information, myths and misconceptions about the vaccine, and one’s experience with the COVID-19 disease and the vaccine. Participants who had either experienced the disease, seen a friend or family member suffer from the disease expressed a positive attitude towards the vaccine compared with those who had not. Similarly, those who had either been vaccinated, seen or heard about someone who had been vaccinated appreciated the benefits of the vaccine and expressed a more positive attitude than those who had no such experience. Protection against COVID-19 and reduction in the severity of the disease if one got infected were the main perceived benefits. Perceived benefits appear to play an important role in influencing people’s attitude towards the vaccine. Participants who perceived no benefits from the vaccine expressed a negative attitude. This finding is in keeping with the reasoned action approach which postulates that, before engaging in a healthy behaviour, people evaluate the benefits against the risks. 56 An individual’s attitude, therefore, will depend on their evaluation of the perceived benefits compared with the risks. Those who perceive more benefits are likely to have a positive attitude towards the target behaviour, and possibly adopt it. This finding is also consistent with those reported by Elhadi et al in Libya. 57 These authors found that people who had a family member or friend infected with COVID-19 were more likely to accept the vaccine. Strategies that use a collaborative approach with community role models who have either experienced the disease or received the vaccine have the potential to change community attitudes towards the vaccine and possibly increase vaccine uptake.
Finally, our findings on low-risk perception and personal susceptibility to the COVID-19 disease, especially among young people, are worthy noting. It appears that young people’s ‘false sense of safety’—that they are not susceptible to the COVID-19 disease and that, if they get infected, the disease would not be severe—seem to influence their attitude towards the vaccine. Access to social media and incorrect information from the internet, especially among the young participants from urban communities, appears to contribute to the low-risk perception and vaccine hesitance. Interestingly, we did not find a striking difference in risk perception between the male and female participants or according to place of residence (urban or rural). This finding contradicts Elhadi et al 57 who reported low vaccine hesitance among young people—that, compared with older people, young people were more likely to accept the vaccine. However, this finding is in line with Lazarus et al 58 who (in their survey of over 13 420 people from 19 countries) reported that young people were less likely to accept the vaccine than older people. The finding is consistent with previous studies that reported that age, and not sex, had a significant association with one’s attitude towards acceptance of the vaccine. These studies also reported a positive correlation between age and vaccine acceptance. They also showed that high risk perception about the severity and one’s personal susceptibility to the disease, benefits from the vaccine, cues to action and trust in the healthcare system or vaccine manufacturers were positive correlates of vaccine acceptance. Interventions that use social media to provide correct information to young people—about their personal risk and susceptibility to the disease—has a potential to mitigate vaccine hesitance among this age group. To be successful, such interventions should focus on addressing behavioural beliefs, risk perception and outcome expectancy. 59
Study limitations
Potential limitations of our study should be noted. First, this study was conducted at the beginning of the COVID-19 national mass vaccination programme in the country when people’s knowledge about the vaccine was still limited; it is not clear how knowledge in the community has evolved over time. Second, like other qualitative study designs, this study could not establish a causal link between knowledge and attitude, and vaccine uptake. Further research with a longitudinal quantitative design is required to measure knowledge and attitude, and test their relationship with vaccine uptake in order to establish the causal pathway.
Nevertheless, we believe that use of FGDs and IDIs comprising adult male and female as well as young participants from both urban and rural settings provided in-depth information on vaccine uptake and the influencing factors, based on the views of the health workers and community members. We believe this study design increased the validity of our findings. Furthermore, selecting participants (both community members and health staff) from both urban and rural settings, increased the internal validity of the study. It also provides a balanced view of the Zambian people’s perspectives on the subject under investigation. Our study also highlights the importance of using an integrated community-based approach to maximise vaccine uptake. This approach is in accordance with the WHO guidelines, 60 which suggest that a comprehensive approach, targeting multiple facets of social interaction, is more likely to dispel COVID-19 myths and misconceptions, and address vaccine hesitancy. Thus, our findings can save as basis for policy and intervention design to mitigate vaccine hesitance and increase vaccine uptake. To our knowledge, no such study has been conducted in Zambia; this is the first one.
Our findings demonstrate low vaccine uptake among our participants; it also highlights several factors—including limited knowledge and access to information, myths and misconceptions, negative attitude towards the vaccine and low-risk perception about COVID-19 disease–which affect vaccine uptake. These results can provide starting points for future Public health policies and interventions which, in our opinion, should focus on: (A) increasing access to information and knowledge about the benefits and safety of the vaccine; (B) addressing myths and misconceptions about the vaccine; (C) increasing risk perception and perceived personal susceptibility to the COVID-19 and its severity, especially among young people; (D) making the vaccine accessible, especially in the rural and remote areas; (E) identifying role models in the community who have either experienced the disease or received the vaccine; (F) establishing linkages and collaboration between health workers and role models; (G) establishing a community operational and vaccine delivery mechanism through strengthened linkages with key community leaders such as local traditional, civic and religious leaders, and (H) addressing systemic barriers such as human resource shortage and stock-outs of the vaccine to increase access to the vaccine in the rural and remote communities.
Supplementary Material
Acknowledgments.
We thank Levy Mwanawasa medical University, Mary Ng’andu for supervising the data collection and the research assistants who helped with the data collection. Our gratitude also goes to the study participants for their valuable time and input into the study.
Contributors: All authors contributed substantially to the development of the manuscript. CS designed the study. Under the oversight of CS, MN supervised the data collection process. CS and NM conducted data analysis. CS wrote the first draft of the manuscript. NM, EMS, W-CP, JMZ, DEML, AM, BM, DE and MM read and provided feedback on the draft manuscript. CS, NM and EMS revised the manuscript. All other coauthors advised on the final draft of the manuscript. All authors read, commented on and approved the final manuscript. CS had access to the data, controlled the decision to publish and is the study guarantor.
Funding: The study was made possible by grant number 20.2095.6-001.00 from Deutsche Gesellschaft für Internationale Zusammenarbeit (GIZ)/German Cooperation through the Decentralisation for Development (Zambia).
Competing interests: None declared.
Patient and public involvement: Patients and/or the public were involved in the design, or conduct, or reporting, or dissemination plans of this research. Refer to the Methods section for further details.
Provenance and peer review: Not commissioned; externally peer reviewed.
Supplemental material: This content has been supplied by the author(s). It has not been vetted by BMJ Publishing Group Limited (BMJ) and may not have been peer-reviewed. Any opinions or recommendations discussed are solely those of the author(s) and are not endorsed by BMJ. BMJ disclaims all liability and responsibility arising from any reliance placed on the content. Where the content includes any translated material, BMJ does not warrant the accuracy and reliability of the translations (including but not limited to local regulations, clinical guidelines, terminology, drug names and drug dosages), and is not responsible for any error and/or omissions arising from translation and adaptation or otherwise.
Data availability statement
Ethics statements, patient consent for publication.
Not applicable.
Ethics approval
This study involves human participants and was approved by University of Zambia Biomedical Research Ethics Committee (ref: 1774-2021). Participants gave informed consent to participate in the study before taking part.
- Research article
- Open access
- Published: 04 June 2021
Coronavirus disease (COVID-19) pandemic: an overview of systematic reviews
- Israel Júnior Borges do Nascimento 1 , 2 ,
- Dónal P. O’Mathúna 3 , 4 ,
- Thilo Caspar von Groote 5 ,
- Hebatullah Mohamed Abdulazeem 6 ,
- Ishanka Weerasekara 7 , 8 ,
- Ana Marusic 9 ,
- Livia Puljak ORCID: orcid.org/0000-0002-8467-6061 10 ,
- Vinicius Tassoni Civile 11 ,
- Irena Zakarija-Grkovic 9 ,
- Tina Poklepovic Pericic 9 ,
- Alvaro Nagib Atallah 11 ,
- Santino Filoso 12 ,
- Nicola Luigi Bragazzi 13 &
- Milena Soriano Marcolino 1
On behalf of the International Network of Coronavirus Disease 2019 (InterNetCOVID-19)
BMC Infectious Diseases volume 21 , Article number: 525 ( 2021 ) Cite this article
16k Accesses
28 Citations
13 Altmetric
Metrics details
Navigating the rapidly growing body of scientific literature on the SARS-CoV-2 pandemic is challenging, and ongoing critical appraisal of this output is essential. We aimed to summarize and critically appraise systematic reviews of coronavirus disease (COVID-19) in humans that were available at the beginning of the pandemic.
Nine databases (Medline, EMBASE, Cochrane Library, CINAHL, Web of Sciences, PDQ-Evidence, WHO’s Global Research, LILACS, and Epistemonikos) were searched from December 1, 2019, to March 24, 2020. Systematic reviews analyzing primary studies of COVID-19 were included. Two authors independently undertook screening, selection, extraction (data on clinical symptoms, prevalence, pharmacological and non-pharmacological interventions, diagnostic test assessment, laboratory, and radiological findings), and quality assessment (AMSTAR 2). A meta-analysis was performed of the prevalence of clinical outcomes.
Eighteen systematic reviews were included; one was empty (did not identify any relevant study). Using AMSTAR 2, confidence in the results of all 18 reviews was rated as “critically low”. Identified symptoms of COVID-19 were (range values of point estimates): fever (82–95%), cough with or without sputum (58–72%), dyspnea (26–59%), myalgia or muscle fatigue (29–51%), sore throat (10–13%), headache (8–12%) and gastrointestinal complaints (5–9%). Severe symptoms were more common in men. Elevated C-reactive protein and lactate dehydrogenase, and slightly elevated aspartate and alanine aminotransferase, were commonly described. Thrombocytopenia and elevated levels of procalcitonin and cardiac troponin I were associated with severe disease. A frequent finding on chest imaging was uni- or bilateral multilobar ground-glass opacity. A single review investigated the impact of medication (chloroquine) but found no verifiable clinical data. All-cause mortality ranged from 0.3 to 13.9%.
Conclusions
In this overview of systematic reviews, we analyzed evidence from the first 18 systematic reviews that were published after the emergence of COVID-19. However, confidence in the results of all reviews was “critically low”. Thus, systematic reviews that were published early on in the pandemic were of questionable usefulness. Even during public health emergencies, studies and systematic reviews should adhere to established methodological standards.
Peer Review reports
The spread of the “Severe Acute Respiratory Coronavirus 2” (SARS-CoV-2), the causal agent of COVID-19, was characterized as a pandemic by the World Health Organization (WHO) in March 2020 and has triggered an international public health emergency [ 1 ]. The numbers of confirmed cases and deaths due to COVID-19 are rapidly escalating, counting in millions [ 2 ], causing massive economic strain, and escalating healthcare and public health expenses [ 3 , 4 ].
The research community has responded by publishing an impressive number of scientific reports related to COVID-19. The world was alerted to the new disease at the beginning of 2020 [ 1 ], and by mid-March 2020, more than 2000 articles had been published on COVID-19 in scholarly journals, with 25% of them containing original data [ 5 ]. The living map of COVID-19 evidence, curated by the Evidence for Policy and Practice Information and Co-ordinating Centre (EPPI-Centre), contained more than 40,000 records by February 2021 [ 6 ]. More than 100,000 records on PubMed were labeled as “SARS-CoV-2 literature, sequence, and clinical content” by February 2021 [ 7 ].
Due to publication speed, the research community has voiced concerns regarding the quality and reproducibility of evidence produced during the COVID-19 pandemic, warning of the potential damaging approach of “publish first, retract later” [ 8 ]. It appears that these concerns are not unfounded, as it has been reported that COVID-19 articles were overrepresented in the pool of retracted articles in 2020 [ 9 ]. These concerns about inadequate evidence are of major importance because they can lead to poor clinical practice and inappropriate policies [ 10 ].
Systematic reviews are a cornerstone of today’s evidence-informed decision-making. By synthesizing all relevant evidence regarding a particular topic, systematic reviews reflect the current scientific knowledge. Systematic reviews are considered to be at the highest level in the hierarchy of evidence and should be used to make informed decisions. However, with high numbers of systematic reviews of different scope and methodological quality being published, overviews of multiple systematic reviews that assess their methodological quality are essential [ 11 , 12 , 13 ]. An overview of systematic reviews helps identify and organize the literature and highlights areas of priority in decision-making.
In this overview of systematic reviews, we aimed to summarize and critically appraise systematic reviews of coronavirus disease (COVID-19) in humans that were available at the beginning of the pandemic.
Methodology
Research question.
This overview’s primary objective was to summarize and critically appraise systematic reviews that assessed any type of primary clinical data from patients infected with SARS-CoV-2. Our research question was purposefully broad because we wanted to analyze as many systematic reviews as possible that were available early following the COVID-19 outbreak.
Study design
We conducted an overview of systematic reviews. The idea for this overview originated in a protocol for a systematic review submitted to PROSPERO (CRD42020170623), which indicated a plan to conduct an overview.
Overviews of systematic reviews use explicit and systematic methods for searching and identifying multiple systematic reviews addressing related research questions in the same field to extract and analyze evidence across important outcomes. Overviews of systematic reviews are in principle similar to systematic reviews of interventions, but the unit of analysis is a systematic review [ 14 , 15 , 16 ].
We used the overview methodology instead of other evidence synthesis methods to allow us to collate and appraise multiple systematic reviews on this topic, and to extract and analyze their results across relevant topics [ 17 ]. The overview and meta-analysis of systematic reviews allowed us to investigate the methodological quality of included studies, summarize results, and identify specific areas of available or limited evidence, thereby strengthening the current understanding of this novel disease and guiding future research [ 13 ].
A reporting guideline for overviews of reviews is currently under development, i.e., Preferred Reporting Items for Overviews of Reviews (PRIOR) [ 18 ]. As the PRIOR checklist is still not published, this study was reported following the Preferred Reporting Items for Systematic Reviews and Meta-Analyses (PRISMA) 2009 statement [ 19 ]. The methodology used in this review was adapted from the Cochrane Handbook for Systematic Reviews of Interventions and also followed established methodological considerations for analyzing existing systematic reviews [ 14 ].
Approval of a research ethics committee was not necessary as the study analyzed only publicly available articles.
Eligibility criteria
Systematic reviews were included if they analyzed primary data from patients infected with SARS-CoV-2 as confirmed by RT-PCR or another pre-specified diagnostic technique. Eligible reviews covered all topics related to COVID-19 including, but not limited to, those that reported clinical symptoms, diagnostic methods, therapeutic interventions, laboratory findings, or radiological results. Both full manuscripts and abbreviated versions, such as letters, were eligible.
No restrictions were imposed on the design of the primary studies included within the systematic reviews, the last search date, whether the review included meta-analyses or language. Reviews related to SARS-CoV-2 and other coronaviruses were eligible, but from those reviews, we analyzed only data related to SARS-CoV-2.
No consensus definition exists for a systematic review [ 20 ], and debates continue about the defining characteristics of a systematic review [ 21 ]. Cochrane’s guidance for overviews of reviews recommends setting pre-established criteria for making decisions around inclusion [ 14 ]. That is supported by a recent scoping review about guidance for overviews of systematic reviews [ 22 ].
Thus, for this study, we defined a systematic review as a research report which searched for primary research studies on a specific topic using an explicit search strategy, had a detailed description of the methods with explicit inclusion criteria provided, and provided a summary of the included studies either in narrative or quantitative format (such as a meta-analysis). Cochrane and non-Cochrane systematic reviews were considered eligible for inclusion, with or without meta-analysis, and regardless of the study design, language restriction and methodology of the included primary studies. To be eligible for inclusion, reviews had to be clearly analyzing data related to SARS-CoV-2 (associated or not with other viruses). We excluded narrative reviews without those characteristics as these are less likely to be replicable and are more prone to bias.
Scoping reviews and rapid reviews were eligible for inclusion in this overview if they met our pre-defined inclusion criteria noted above. We included reviews that addressed SARS-CoV-2 and other coronaviruses if they reported separate data regarding SARS-CoV-2.
Information sources
Nine databases were searched for eligible records published between December 1, 2019, and March 24, 2020: Cochrane Database of Systematic Reviews via Cochrane Library, PubMed, EMBASE, CINAHL (Cumulative Index to Nursing and Allied Health Literature), Web of Sciences, LILACS (Latin American and Caribbean Health Sciences Literature), PDQ-Evidence, WHO’s Global Research on Coronavirus Disease (COVID-19), and Epistemonikos.
The comprehensive search strategy for each database is provided in Additional file 1 and was designed and conducted in collaboration with an information specialist. All retrieved records were primarily processed in EndNote, where duplicates were removed, and records were then imported into the Covidence platform [ 23 ]. In addition to database searches, we screened reference lists of reviews included after screening records retrieved via databases.
Study selection
All searches, screening of titles and abstracts, and record selection, were performed independently by two investigators using the Covidence platform [ 23 ]. Articles deemed potentially eligible were retrieved for full-text screening carried out independently by two investigators. Discrepancies at all stages were resolved by consensus. During the screening, records published in languages other than English were translated by a native/fluent speaker.
Data collection process
We custom designed a data extraction table for this study, which was piloted by two authors independently. Data extraction was performed independently by two authors. Conflicts were resolved by consensus or by consulting a third researcher.
We extracted the following data: article identification data (authors’ name and journal of publication), search period, number of databases searched, population or settings considered, main results and outcomes observed, and number of participants. From Web of Science (Clarivate Analytics, Philadelphia, PA, USA), we extracted journal rank (quartile) and Journal Impact Factor (JIF).
We categorized the following as primary outcomes: all-cause mortality, need for and length of mechanical ventilation, length of hospitalization (in days), admission to intensive care unit (yes/no), and length of stay in the intensive care unit.
The following outcomes were categorized as exploratory: diagnostic methods used for detection of the virus, male to female ratio, clinical symptoms, pharmacological and non-pharmacological interventions, laboratory findings (full blood count, liver enzymes, C-reactive protein, d-dimer, albumin, lipid profile, serum electrolytes, blood vitamin levels, glucose levels, and any other important biomarkers), and radiological findings (using radiography, computed tomography, magnetic resonance imaging or ultrasound).
We also collected data on reporting guidelines and requirements for the publication of systematic reviews and meta-analyses from journal websites where included reviews were published.
Quality assessment in individual reviews
Two researchers independently assessed the reviews’ quality using the “A MeaSurement Tool to Assess Systematic Reviews 2 (AMSTAR 2)”. We acknowledge that the AMSTAR 2 was created as “a critical appraisal tool for systematic reviews that include randomized or non-randomized studies of healthcare interventions, or both” [ 24 ]. However, since AMSTAR 2 was designed for systematic reviews of intervention trials, and we included additional types of systematic reviews, we adjusted some AMSTAR 2 ratings and reported these in Additional file 2 .
Adherence to each item was rated as follows: yes, partial yes, no, or not applicable (such as when a meta-analysis was not conducted). The overall confidence in the results of the review is rated as “critically low”, “low”, “moderate” or “high”, according to the AMSTAR 2 guidance based on seven critical domains, which are items 2, 4, 7, 9, 11, 13, 15 as defined by AMSTAR 2 authors [ 24 ]. We reported our adherence ratings for transparency of our decision with accompanying explanations, for each item, in each included review.
One of the included systematic reviews was conducted by some members of this author team [ 25 ]. This review was initially assessed independently by two authors who were not co-authors of that review to prevent the risk of bias in assessing this study.
Synthesis of results
For data synthesis, we prepared a table summarizing each systematic review. Graphs illustrating the mortality rate and clinical symptoms were created. We then prepared a narrative summary of the methods, findings, study strengths, and limitations.
For analysis of the prevalence of clinical outcomes, we extracted data on the number of events and the total number of patients to perform proportional meta-analysis using RStudio© software, with the “meta” package (version 4.9–6), using the “metaprop” function for reviews that did not perform a meta-analysis, excluding case studies because of the absence of variance. For reviews that did not perform a meta-analysis, we presented pooled results of proportions with their respective confidence intervals (95%) by the inverse variance method with a random-effects model, using the DerSimonian-Laird estimator for τ 2 . We adjusted data using Freeman-Tukey double arcosen transformation. Confidence intervals were calculated using the Clopper-Pearson method for individual studies. We created forest plots using the RStudio© software, with the “metafor” package (version 2.1–0) and “forest” function.
Managing overlapping systematic reviews
Some of the included systematic reviews that address the same or similar research questions may include the same primary studies in overviews. Including such overlapping reviews may introduce bias when outcome data from the same primary study are included in the analyses of an overview multiple times. Thus, in summaries of evidence, multiple-counting of the same outcome data will give data from some primary studies too much influence [ 14 ]. In this overview, we did not exclude overlapping systematic reviews because, according to Cochrane’s guidance, it may be appropriate to include all relevant reviews’ results if the purpose of the overview is to present and describe the current body of evidence on a topic [ 14 ]. To avoid any bias in summary estimates associated with overlapping reviews, we generated forest plots showing data from individual systematic reviews, but the results were not pooled because some primary studies were included in multiple reviews.
Our search retrieved 1063 publications, of which 175 were duplicates. Most publications were excluded after the title and abstract analysis ( n = 860). Among the 28 studies selected for full-text screening, 10 were excluded for the reasons described in Additional file 3 , and 18 were included in the final analysis (Fig. 1 ) [ 25 , 26 , 27 , 28 , 29 , 30 , 31 , 32 , 33 , 34 , 35 , 36 , 37 , 38 , 39 , 40 , 41 , 42 ]. Reference list screening did not retrieve any additional systematic reviews.
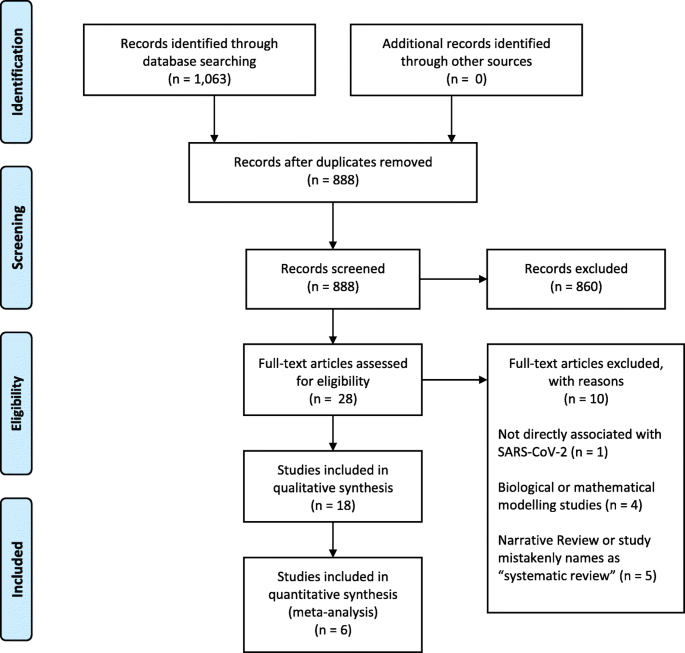
PRISMA flow diagram
Characteristics of included reviews
Summary features of 18 systematic reviews are presented in Table 1 . They were published in 14 different journals. Only four of these journals had specific requirements for systematic reviews (with or without meta-analysis): European Journal of Internal Medicine, Journal of Clinical Medicine, Ultrasound in Obstetrics and Gynecology, and Clinical Research in Cardiology . Two journals reported that they published only invited reviews ( Journal of Medical Virology and Clinica Chimica Acta ). Three systematic reviews in our study were published as letters; one was labeled as a scoping review and another as a rapid review (Table 2 ).
All reviews were published in English, in first quartile (Q1) journals, with JIF ranging from 1.692 to 6.062. One review was empty, meaning that its search did not identify any relevant studies; i.e., no primary studies were included [ 36 ]. The remaining 17 reviews included 269 unique studies; the majority ( N = 211; 78%) were included in only a single review included in our study (range: 1 to 12). Primary studies included in the reviews were published between December 2019 and March 18, 2020, and comprised case reports, case series, cohorts, and other observational studies. We found only one review that included randomized clinical trials [ 38 ]. In the included reviews, systematic literature searches were performed from 2019 (entire year) up to March 9, 2020. Ten systematic reviews included meta-analyses. The list of primary studies found in the included systematic reviews is shown in Additional file 4 , as well as the number of reviews in which each primary study was included.
Population and study designs
Most of the reviews analyzed data from patients with COVID-19 who developed pneumonia, acute respiratory distress syndrome (ARDS), or any other correlated complication. One review aimed to evaluate the effectiveness of using surgical masks on preventing transmission of the virus [ 36 ], one review was focused on pediatric patients [ 34 ], and one review investigated COVID-19 in pregnant women [ 37 ]. Most reviews assessed clinical symptoms, laboratory findings, or radiological results.
Systematic review findings
The summary of findings from individual reviews is shown in Table 2 . Overall, all-cause mortality ranged from 0.3 to 13.9% (Fig. 2 ).
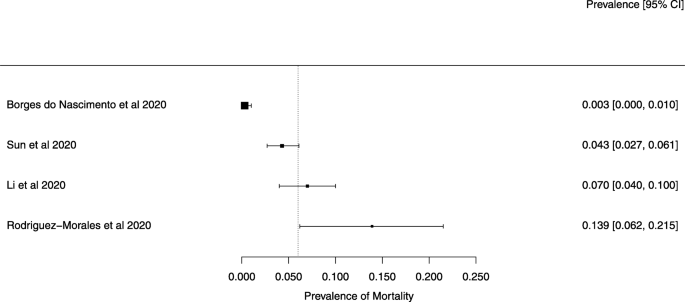
A meta-analysis of the prevalence of mortality
Clinical symptoms
Seven reviews described the main clinical manifestations of COVID-19 [ 26 , 28 , 29 , 34 , 35 , 39 , 41 ]. Three of them provided only a narrative discussion of symptoms [ 26 , 34 , 35 ]. In the reviews that performed a statistical analysis of the incidence of different clinical symptoms, symptoms in patients with COVID-19 were (range values of point estimates): fever (82–95%), cough with or without sputum (58–72%), dyspnea (26–59%), myalgia or muscle fatigue (29–51%), sore throat (10–13%), headache (8–12%), gastrointestinal disorders, such as diarrhea, nausea or vomiting (5.0–9.0%), and others (including, in one study only: dizziness 12.1%) (Figs. 3 , 4 , 5 , 6 , 7 , 8 and 9 ). Three reviews assessed cough with and without sputum together; only one review assessed sputum production itself (28.5%).
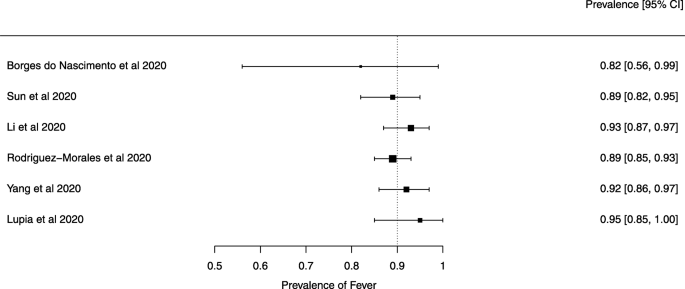
A meta-analysis of the prevalence of fever
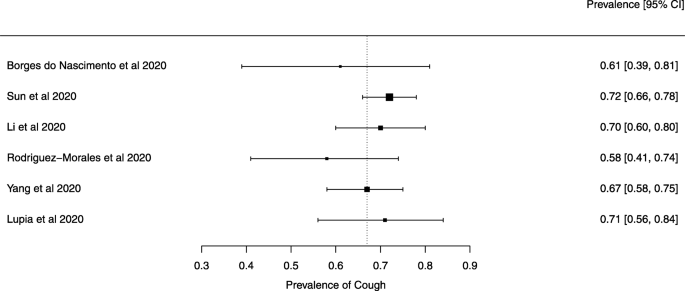
A meta-analysis of the prevalence of cough
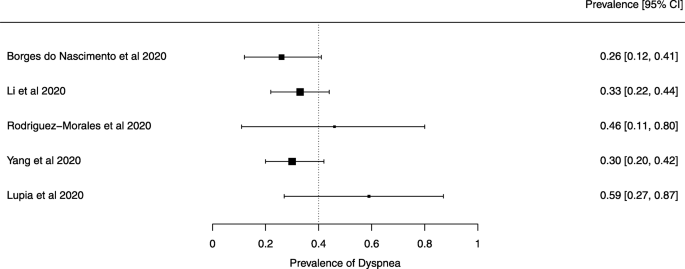
A meta-analysis of the prevalence of dyspnea
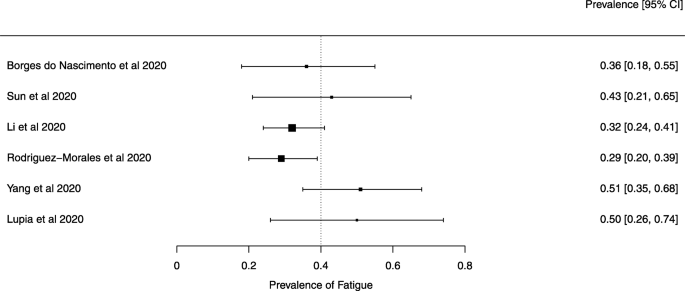
A meta-analysis of the prevalence of fatigue or myalgia
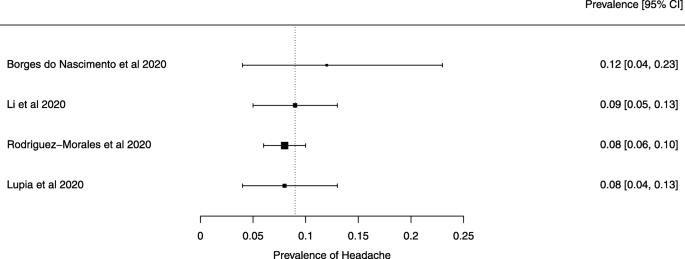
A meta-analysis of the prevalence of headache
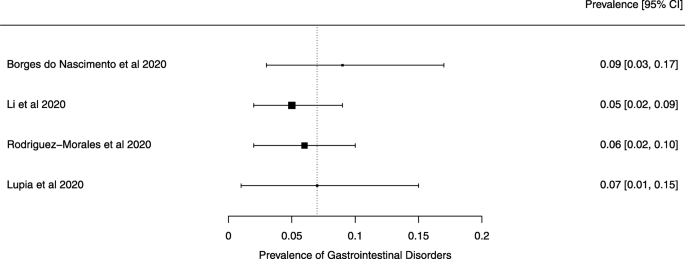
A meta-analysis of the prevalence of gastrointestinal disorders
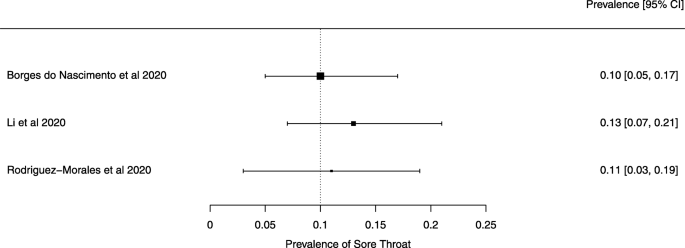
A meta-analysis of the prevalence of sore throat
Diagnostic aspects
Three reviews described methodologies, protocols, and tools used for establishing the diagnosis of COVID-19 [ 26 , 34 , 38 ]. The use of respiratory swabs (nasal or pharyngeal) or blood specimens to assess the presence of SARS-CoV-2 nucleic acid using RT-PCR assays was the most commonly used diagnostic method mentioned in the included studies. These diagnostic tests have been widely used, but their precise sensitivity and specificity remain unknown. One review included a Chinese study with clinical diagnosis with no confirmation of SARS-CoV-2 infection (patients were diagnosed with COVID-19 if they presented with at least two symptoms suggestive of COVID-19, together with laboratory and chest radiography abnormalities) [ 34 ].
Therapeutic possibilities
Pharmacological and non-pharmacological interventions (supportive therapies) used in treating patients with COVID-19 were reported in five reviews [ 25 , 27 , 34 , 35 , 38 ]. Antivirals used empirically for COVID-19 treatment were reported in seven reviews [ 25 , 27 , 34 , 35 , 37 , 38 , 41 ]; most commonly used were protease inhibitors (lopinavir, ritonavir, darunavir), nucleoside reverse transcriptase inhibitor (tenofovir), nucleotide analogs (remdesivir, galidesivir, ganciclovir), and neuraminidase inhibitors (oseltamivir). Umifenovir, a membrane fusion inhibitor, was investigated in two studies [ 25 , 35 ]. Possible supportive interventions analyzed were different types of oxygen supplementation and breathing support (invasive or non-invasive ventilation) [ 25 ]. The use of antibiotics, both empirically and to treat secondary pneumonia, was reported in six studies [ 25 , 26 , 27 , 34 , 35 , 38 ]. One review specifically assessed evidence on the efficacy and safety of the anti-malaria drug chloroquine [ 27 ]. It identified 23 ongoing trials investigating the potential of chloroquine as a therapeutic option for COVID-19, but no verifiable clinical outcomes data. The use of mesenchymal stem cells, antifungals, and glucocorticoids were described in four reviews [ 25 , 34 , 35 , 38 ].
Laboratory and radiological findings
Of the 18 reviews included in this overview, eight analyzed laboratory parameters in patients with COVID-19 [ 25 , 29 , 30 , 32 , 33 , 34 , 35 , 39 ]; elevated C-reactive protein levels, associated with lymphocytopenia, elevated lactate dehydrogenase, as well as slightly elevated aspartate and alanine aminotransferase (AST, ALT) were commonly described in those eight reviews. Lippi et al. assessed cardiac troponin I (cTnI) [ 25 ], procalcitonin [ 32 ], and platelet count [ 33 ] in COVID-19 patients. Elevated levels of procalcitonin [ 32 ] and cTnI [ 30 ] were more likely to be associated with a severe disease course (requiring intensive care unit admission and intubation). Furthermore, thrombocytopenia was frequently observed in patients with complicated COVID-19 infections [ 33 ].
Chest imaging (chest radiography and/or computed tomography) features were assessed in six reviews, all of which described a frequent pattern of local or bilateral multilobar ground-glass opacity [ 25 , 34 , 35 , 39 , 40 , 41 ]. Those six reviews showed that septal thickening, bronchiectasis, pleural and cardiac effusions, halo signs, and pneumothorax were observed in patients suffering from COVID-19.
Quality of evidence in individual systematic reviews
Table 3 shows the detailed results of the quality assessment of 18 systematic reviews, including the assessment of individual items and summary assessment. A detailed explanation for each decision in each review is available in Additional file 5 .
Using AMSTAR 2 criteria, confidence in the results of all 18 reviews was rated as “critically low” (Table 3 ). Common methodological drawbacks were: omission of prospective protocol submission or publication; use of inappropriate search strategy: lack of independent and dual literature screening and data-extraction (or methodology unclear); absence of an explanation for heterogeneity among the studies included; lack of reasons for study exclusion (or rationale unclear).
Risk of bias assessment, based on a reported methodological tool, and quality of evidence appraisal, in line with the Grading of Recommendations Assessment, Development, and Evaluation (GRADE) method, were reported only in one review [ 25 ]. Five reviews presented a table summarizing bias, using various risk of bias tools [ 25 , 29 , 39 , 40 , 41 ]. One review analyzed “study quality” [ 37 ]. One review mentioned the risk of bias assessment in the methodology but did not provide any related analysis [ 28 ].
This overview of systematic reviews analyzed the first 18 systematic reviews published after the onset of the COVID-19 pandemic, up to March 24, 2020, with primary studies involving more than 60,000 patients. Using AMSTAR-2, we judged that our confidence in all those reviews was “critically low”. Ten reviews included meta-analyses. The reviews presented data on clinical manifestations, laboratory and radiological findings, and interventions. We found no systematic reviews on the utility of diagnostic tests.
Symptoms were reported in seven reviews; most of the patients had a fever, cough, dyspnea, myalgia or muscle fatigue, and gastrointestinal disorders such as diarrhea, nausea, or vomiting. Olfactory dysfunction (anosmia or dysosmia) has been described in patients infected with COVID-19 [ 43 ]; however, this was not reported in any of the reviews included in this overview. During the SARS outbreak in 2002, there were reports of impairment of the sense of smell associated with the disease [ 44 , 45 ].
The reported mortality rates ranged from 0.3 to 14% in the included reviews. Mortality estimates are influenced by the transmissibility rate (basic reproduction number), availability of diagnostic tools, notification policies, asymptomatic presentations of the disease, resources for disease prevention and control, and treatment facilities; variability in the mortality rate fits the pattern of emerging infectious diseases [ 46 ]. Furthermore, the reported cases did not consider asymptomatic cases, mild cases where individuals have not sought medical treatment, and the fact that many countries had limited access to diagnostic tests or have implemented testing policies later than the others. Considering the lack of reviews assessing diagnostic testing (sensitivity, specificity, and predictive values of RT-PCT or immunoglobulin tests), and the preponderance of studies that assessed only symptomatic individuals, considerable imprecision around the calculated mortality rates existed in the early stage of the COVID-19 pandemic.
Few reviews included treatment data. Those reviews described studies considered to be at a very low level of evidence: usually small, retrospective studies with very heterogeneous populations. Seven reviews analyzed laboratory parameters; those reviews could have been useful for clinicians who attend patients suspected of COVID-19 in emergency services worldwide, such as assessing which patients need to be reassessed more frequently.
All systematic reviews scored poorly on the AMSTAR 2 critical appraisal tool for systematic reviews. Most of the original studies included in the reviews were case series and case reports, impacting the quality of evidence. Such evidence has major implications for clinical practice and the use of these reviews in evidence-based practice and policy. Clinicians, patients, and policymakers can only have the highest confidence in systematic review findings if high-quality systematic review methodologies are employed. The urgent need for information during a pandemic does not justify poor quality reporting.
We acknowledge that there are numerous challenges associated with analyzing COVID-19 data during a pandemic [ 47 ]. High-quality evidence syntheses are needed for decision-making, but each type of evidence syntheses is associated with its inherent challenges.
The creation of classic systematic reviews requires considerable time and effort; with massive research output, they quickly become outdated, and preparing updated versions also requires considerable time. A recent study showed that updates of non-Cochrane systematic reviews are published a median of 5 years after the publication of the previous version [ 48 ].
Authors may register a review and then abandon it [ 49 ], but the existence of a public record that is not updated may lead other authors to believe that the review is still ongoing. A quarter of Cochrane review protocols remains unpublished as completed systematic reviews 8 years after protocol publication [ 50 ].
Rapid reviews can be used to summarize the evidence, but they involve methodological sacrifices and simplifications to produce information promptly, with inconsistent methodological approaches [ 51 ]. However, rapid reviews are justified in times of public health emergencies, and even Cochrane has resorted to publishing rapid reviews in response to the COVID-19 crisis [ 52 ]. Rapid reviews were eligible for inclusion in this overview, but only one of the 18 reviews included in this study was labeled as a rapid review.
Ideally, COVID-19 evidence would be continually summarized in a series of high-quality living systematic reviews, types of evidence synthesis defined as “ a systematic review which is continually updated, incorporating relevant new evidence as it becomes available ” [ 53 ]. However, conducting living systematic reviews requires considerable resources, calling into question the sustainability of such evidence synthesis over long periods [ 54 ].
Research reports about COVID-19 will contribute to research waste if they are poorly designed, poorly reported, or simply not necessary. In principle, systematic reviews should help reduce research waste as they usually provide recommendations for further research that is needed or may advise that sufficient evidence exists on a particular topic [ 55 ]. However, systematic reviews can also contribute to growing research waste when they are not needed, or poorly conducted and reported. Our present study clearly shows that most of the systematic reviews that were published early on in the COVID-19 pandemic could be categorized as research waste, as our confidence in their results is critically low.
Our study has some limitations. One is that for AMSTAR 2 assessment we relied on information available in publications; we did not attempt to contact study authors for clarifications or additional data. In three reviews, the methodological quality appraisal was challenging because they were published as letters, or labeled as rapid communications. As a result, various details about their review process were not included, leading to AMSTAR 2 questions being answered as “not reported”, resulting in low confidence scores. Full manuscripts might have provided additional information that could have led to higher confidence in the results. In other words, low scores could reflect incomplete reporting, not necessarily low-quality review methods. To make their review available more rapidly and more concisely, the authors may have omitted methodological details. A general issue during a crisis is that speed and completeness must be balanced. However, maintaining high standards requires proper resourcing and commitment to ensure that the users of systematic reviews can have high confidence in the results.
Furthermore, we used adjusted AMSTAR 2 scoring, as the tool was designed for critical appraisal of reviews of interventions. Some reviews may have received lower scores than actually warranted in spite of these adjustments.
Another limitation of our study may be the inclusion of multiple overlapping reviews, as some included reviews included the same primary studies. According to the Cochrane Handbook, including overlapping reviews may be appropriate when the review’s aim is “ to present and describe the current body of systematic review evidence on a topic ” [ 12 ], which was our aim. To avoid bias with summarizing evidence from overlapping reviews, we presented the forest plots without summary estimates. The forest plots serve to inform readers about the effect sizes for outcomes that were reported in each review.
Several authors from this study have contributed to one of the reviews identified [ 25 ]. To reduce the risk of any bias, two authors who did not co-author the review in question initially assessed its quality and limitations.
Finally, we note that the systematic reviews included in our overview may have had issues that our analysis did not identify because we did not analyze their primary studies to verify the accuracy of the data and information they presented. We give two examples to substantiate this possibility. Lovato et al. wrote a commentary on the review of Sun et al. [ 41 ], in which they criticized the authors’ conclusion that sore throat is rare in COVID-19 patients [ 56 ]. Lovato et al. highlighted that multiple studies included in Sun et al. did not accurately describe participants’ clinical presentations, warning that only three studies clearly reported data on sore throat [ 56 ].
In another example, Leung [ 57 ] warned about the review of Li, L.Q. et al. [ 29 ]: “ it is possible that this statistic was computed using overlapped samples, therefore some patients were double counted ”. Li et al. responded to Leung that it is uncertain whether the data overlapped, as they used data from published articles and did not have access to the original data; they also reported that they requested original data and that they plan to re-do their analyses once they receive them; they also urged readers to treat the data with caution [ 58 ]. This points to the evolving nature of evidence during a crisis.
Our study’s strength is that this overview adds to the current knowledge by providing a comprehensive summary of all the evidence synthesis about COVID-19 available early after the onset of the pandemic. This overview followed strict methodological criteria, including a comprehensive and sensitive search strategy and a standard tool for methodological appraisal of systematic reviews.
In conclusion, in this overview of systematic reviews, we analyzed evidence from the first 18 systematic reviews that were published after the emergence of COVID-19. However, confidence in the results of all the reviews was “critically low”. Thus, systematic reviews that were published early on in the pandemic could be categorized as research waste. Even during public health emergencies, studies and systematic reviews should adhere to established methodological standards to provide patients, clinicians, and decision-makers trustworthy evidence.
Availability of data and materials
All data collected and analyzed within this study are available from the corresponding author on reasonable request.
World Health Organization. Timeline - COVID-19: Available at: https://www.who.int/news/item/29-06-2020-covidtimeline . Accessed 1 June 2021.
COVID-19 Dashboard by the Center for Systems Science and Engineering (CSSE) at Johns Hopkins University (JHU). Available at: https://coronavirus.jhu.edu/map.html . Accessed 1 June 2021.
Anzai A, Kobayashi T, Linton NM, Kinoshita R, Hayashi K, Suzuki A, et al. Assessing the Impact of Reduced Travel on Exportation Dynamics of Novel Coronavirus Infection (COVID-19). J Clin Med. 2020;9(2):601.
Chinazzi M, Davis JT, Ajelli M, Gioannini C, Litvinova M, Merler S, et al. The effect of travel restrictions on the spread of the 2019 novel coronavirus (COVID-19) outbreak. Science. 2020;368(6489):395–400. https://doi.org/10.1126/science.aba9757 .
Article CAS PubMed PubMed Central Google Scholar
Fidahic M, Nujic D, Runjic R, Civljak M, Markotic F, Lovric Makaric Z, et al. Research methodology and characteristics of journal articles with original data, preprint articles and registered clinical trial protocols about COVID-19. BMC Med Res Methodol. 2020;20(1):161. https://doi.org/10.1186/s12874-020-01047-2 .
EPPI Centre . COVID-19: a living systematic map of the evidence. Available at: http://eppi.ioe.ac.uk/cms/Projects/DepartmentofHealthandSocialCare/Publishedreviews/COVID-19Livingsystematicmapoftheevidence/tabid/3765/Default.aspx . Accessed 1 June 2021.
NCBI SARS-CoV-2 Resources. Available at: https://www.ncbi.nlm.nih.gov/sars-cov-2/ . Accessed 1 June 2021.
Gustot T. Quality and reproducibility during the COVID-19 pandemic. JHEP Rep. 2020;2(4):100141. https://doi.org/10.1016/j.jhepr.2020.100141 .
Article PubMed PubMed Central Google Scholar
Kodvanj, I., et al., Publishing of COVID-19 Preprints in Peer-reviewed Journals, Preprinting Trends, Public Discussion and Quality Issues. Preprint article. bioRxiv 2020.11.23.394577; doi: https://doi.org/10.1101/2020.11.23.394577 .
Dobler CC. Poor quality research and clinical practice during COVID-19. Breathe (Sheff). 2020;16(2):200112. https://doi.org/10.1183/20734735.0112-2020 .
Article Google Scholar
Bastian H, Glasziou P, Chalmers I. Seventy-five trials and eleven systematic reviews a day: how will we ever keep up? PLoS Med. 2010;7(9):e1000326. https://doi.org/10.1371/journal.pmed.1000326 .
Lunny C, Brennan SE, McDonald S, McKenzie JE. Toward a comprehensive evidence map of overview of systematic review methods: paper 1-purpose, eligibility, search and data extraction. Syst Rev. 2017;6(1):231. https://doi.org/10.1186/s13643-017-0617-1 .
Pollock M, Fernandes RM, Becker LA, Pieper D, Hartling L. Chapter V: Overviews of Reviews. In: Higgins JPT, Thomas J, Chandler J, Cumpston M, Li T, Page MJ, Welch VA (editors). Cochrane Handbook for Systematic Reviews of Interventions version 6.1 (updated September 2020). Cochrane. 2020. Available from www.training.cochrane.org/handbook .
Higgins JPT, Thomas J, Chandler J, Cumpston M, Li T, Page MJ, et al. Cochrane handbook for systematic reviews of interventions version 6.1 (updated September 2020). Cochrane. 2020; Available from www.training.cochrane.org/handbook .
Pollock M, Fernandes RM, Newton AS, Scott SD, Hartling L. The impact of different inclusion decisions on the comprehensiveness and complexity of overviews of reviews of healthcare interventions. Syst Rev. 2019;8(1):18. https://doi.org/10.1186/s13643-018-0914-3 .
Pollock M, Fernandes RM, Newton AS, Scott SD, Hartling L. A decision tool to help researchers make decisions about including systematic reviews in overviews of reviews of healthcare interventions. Syst Rev. 2019;8(1):29. https://doi.org/10.1186/s13643-018-0768-8 .
Hunt H, Pollock A, Campbell P, Estcourt L, Brunton G. An introduction to overviews of reviews: planning a relevant research question and objective for an overview. Syst Rev. 2018;7(1):39. https://doi.org/10.1186/s13643-018-0695-8 .
Pollock M, Fernandes RM, Pieper D, Tricco AC, Gates M, Gates A, et al. Preferred reporting items for overviews of reviews (PRIOR): a protocol for development of a reporting guideline for overviews of reviews of healthcare interventions. Syst Rev. 2019;8(1):335. https://doi.org/10.1186/s13643-019-1252-9 .
Moher D, Liberati A, Tetzlaff J, Altman DG, PRISMA Group. Preferred reporting items for systematic reviews and meta-analyses: the PRISMA statement. Open Med. 2009;3(3):e123–30.
Krnic Martinic M, Pieper D, Glatt A, Puljak L. Definition of a systematic review used in overviews of systematic reviews, meta-epidemiological studies and textbooks. BMC Med Res Methodol. 2019;19(1):203. https://doi.org/10.1186/s12874-019-0855-0 .
Puljak L. If there is only one author or only one database was searched, a study should not be called a systematic review. J Clin Epidemiol. 2017;91:4–5. https://doi.org/10.1016/j.jclinepi.2017.08.002 .
Article PubMed Google Scholar
Gates M, Gates A, Guitard S, Pollock M, Hartling L. Guidance for overviews of reviews continues to accumulate, but important challenges remain: a scoping review. Syst Rev. 2020;9(1):254. https://doi.org/10.1186/s13643-020-01509-0 .
Covidence - systematic review software. Available at: https://www.covidence.org/ . Accessed 1 June 2021.
Shea BJ, Reeves BC, Wells G, Thuku M, Hamel C, Moran J, et al. AMSTAR 2: a critical appraisal tool for systematic reviews that include randomised or non-randomised studies of healthcare interventions, or both. BMJ. 2017;358:j4008.
Borges do Nascimento IJ, et al. Novel Coronavirus Infection (COVID-19) in Humans: A Scoping Review and Meta-Analysis. J Clin Med. 2020;9(4):941.
Article PubMed Central Google Scholar
Adhikari SP, Meng S, Wu YJ, Mao YP, Ye RX, Wang QZ, et al. Epidemiology, causes, clinical manifestation and diagnosis, prevention and control of coronavirus disease (COVID-19) during the early outbreak period: a scoping review. Infect Dis Poverty. 2020;9(1):29. https://doi.org/10.1186/s40249-020-00646-x .
Cortegiani A, Ingoglia G, Ippolito M, Giarratano A, Einav S. A systematic review on the efficacy and safety of chloroquine for the treatment of COVID-19. J Crit Care. 2020;57:279–83. https://doi.org/10.1016/j.jcrc.2020.03.005 .
Li B, Yang J, Zhao F, Zhi L, Wang X, Liu L, et al. Prevalence and impact of cardiovascular metabolic diseases on COVID-19 in China. Clin Res Cardiol. 2020;109(5):531–8. https://doi.org/10.1007/s00392-020-01626-9 .
Article CAS PubMed Google Scholar
Li LQ, Huang T, Wang YQ, Wang ZP, Liang Y, Huang TB, et al. COVID-19 patients’ clinical characteristics, discharge rate, and fatality rate of meta-analysis. J Med Virol. 2020;92(6):577–83. https://doi.org/10.1002/jmv.25757 .
Lippi G, Lavie CJ, Sanchis-Gomar F. Cardiac troponin I in patients with coronavirus disease 2019 (COVID-19): evidence from a meta-analysis. Prog Cardiovasc Dis. 2020;63(3):390–1. https://doi.org/10.1016/j.pcad.2020.03.001 .
Lippi G, Henry BM. Active smoking is not associated with severity of coronavirus disease 2019 (COVID-19). Eur J Intern Med. 2020;75:107–8. https://doi.org/10.1016/j.ejim.2020.03.014 .
Lippi G, Plebani M. Procalcitonin in patients with severe coronavirus disease 2019 (COVID-19): a meta-analysis. Clin Chim Acta. 2020;505:190–1. https://doi.org/10.1016/j.cca.2020.03.004 .
Lippi G, Plebani M, Henry BM. Thrombocytopenia is associated with severe coronavirus disease 2019 (COVID-19) infections: a meta-analysis. Clin Chim Acta. 2020;506:145–8. https://doi.org/10.1016/j.cca.2020.03.022 .
Ludvigsson JF. Systematic review of COVID-19 in children shows milder cases and a better prognosis than adults. Acta Paediatr. 2020;109(6):1088–95. https://doi.org/10.1111/apa.15270 .
Lupia T, Scabini S, Mornese Pinna S, di Perri G, de Rosa FG, Corcione S. 2019 novel coronavirus (2019-nCoV) outbreak: a new challenge. J Glob Antimicrob Resist. 2020;21:22–7. https://doi.org/10.1016/j.jgar.2020.02.021 .
Marasinghe, K.M., A systematic review investigating the effectiveness of face mask use in limiting the spread of COVID-19 among medically not diagnosed individuals: shedding light on current recommendations provided to individuals not medically diagnosed with COVID-19. Research Square. Preprint article. doi : https://doi.org/10.21203/rs.3.rs-16701/v1 . 2020 .
Mullins E, Evans D, Viner RM, O’Brien P, Morris E. Coronavirus in pregnancy and delivery: rapid review. Ultrasound Obstet Gynecol. 2020;55(5):586–92. https://doi.org/10.1002/uog.22014 .
Pang J, Wang MX, Ang IYH, Tan SHX, Lewis RF, Chen JIP, et al. Potential Rapid Diagnostics, Vaccine and Therapeutics for 2019 Novel coronavirus (2019-nCoV): a systematic review. J Clin Med. 2020;9(3):623.
Rodriguez-Morales AJ, Cardona-Ospina JA, Gutiérrez-Ocampo E, Villamizar-Peña R, Holguin-Rivera Y, Escalera-Antezana JP, et al. Clinical, laboratory and imaging features of COVID-19: a systematic review and meta-analysis. Travel Med Infect Dis. 2020;34:101623. https://doi.org/10.1016/j.tmaid.2020.101623 .
Salehi S, Abedi A, Balakrishnan S, Gholamrezanezhad A. Coronavirus disease 2019 (COVID-19): a systematic review of imaging findings in 919 patients. AJR Am J Roentgenol. 2020;215(1):87–93. https://doi.org/10.2214/AJR.20.23034 .
Sun P, Qie S, Liu Z, Ren J, Li K, Xi J. Clinical characteristics of hospitalized patients with SARS-CoV-2 infection: a single arm meta-analysis. J Med Virol. 2020;92(6):612–7. https://doi.org/10.1002/jmv.25735 .
Yang J, Zheng Y, Gou X, Pu K, Chen Z, Guo Q, et al. Prevalence of comorbidities and its effects in patients infected with SARS-CoV-2: a systematic review and meta-analysis. Int J Infect Dis. 2020;94:91–5. https://doi.org/10.1016/j.ijid.2020.03.017 .
Bassetti M, Vena A, Giacobbe DR. The novel Chinese coronavirus (2019-nCoV) infections: challenges for fighting the storm. Eur J Clin Investig. 2020;50(3):e13209. https://doi.org/10.1111/eci.13209 .
Article CAS Google Scholar
Hwang CS. Olfactory neuropathy in severe acute respiratory syndrome: report of a case. Acta Neurol Taiwanica. 2006;15(1):26–8.
Google Scholar
Suzuki M, Saito K, Min WP, Vladau C, Toida K, Itoh H, et al. Identification of viruses in patients with postviral olfactory dysfunction. Laryngoscope. 2007;117(2):272–7. https://doi.org/10.1097/01.mlg.0000249922.37381.1e .
Rajgor DD, Lee MH, Archuleta S, Bagdasarian N, Quek SC. The many estimates of the COVID-19 case fatality rate. Lancet Infect Dis. 2020;20(7):776–7. https://doi.org/10.1016/S1473-3099(20)30244-9 .
Wolkewitz M, Puljak L. Methodological challenges of analysing COVID-19 data during the pandemic. BMC Med Res Methodol. 2020;20(1):81. https://doi.org/10.1186/s12874-020-00972-6 .
Rombey T, Lochner V, Puljak L, Könsgen N, Mathes T, Pieper D. Epidemiology and reporting characteristics of non-Cochrane updates of systematic reviews: a cross-sectional study. Res Synth Methods. 2020;11(3):471–83. https://doi.org/10.1002/jrsm.1409 .
Runjic E, Rombey T, Pieper D, Puljak L. Half of systematic reviews about pain registered in PROSPERO were not published and the majority had inaccurate status. J Clin Epidemiol. 2019;116:114–21. https://doi.org/10.1016/j.jclinepi.2019.08.010 .
Runjic E, Behmen D, Pieper D, Mathes T, Tricco AC, Moher D, et al. Following Cochrane review protocols to completion 10 years later: a retrospective cohort study and author survey. J Clin Epidemiol. 2019;111:41–8. https://doi.org/10.1016/j.jclinepi.2019.03.006 .
Tricco AC, Antony J, Zarin W, Strifler L, Ghassemi M, Ivory J, et al. A scoping review of rapid review methods. BMC Med. 2015;13(1):224. https://doi.org/10.1186/s12916-015-0465-6 .
COVID-19 Rapid Reviews: Cochrane’s response so far. Available at: https://training.cochrane.org/resource/covid-19-rapid-reviews-cochrane-response-so-far . Accessed 1 June 2021.
Cochrane. Living systematic reviews. Available at: https://community.cochrane.org/review-production/production-resources/living-systematic-reviews . Accessed 1 June 2021.
Millard T, Synnot A, Elliott J, Green S, McDonald S, Turner T. Feasibility and acceptability of living systematic reviews: results from a mixed-methods evaluation. Syst Rev. 2019;8(1):325. https://doi.org/10.1186/s13643-019-1248-5 .
Babic A, Poklepovic Pericic T, Pieper D, Puljak L. How to decide whether a systematic review is stable and not in need of updating: analysis of Cochrane reviews. Res Synth Methods. 2020;11(6):884–90. https://doi.org/10.1002/jrsm.1451 .
Lovato A, Rossettini G, de Filippis C. Sore throat in COVID-19: comment on “clinical characteristics of hospitalized patients with SARS-CoV-2 infection: a single arm meta-analysis”. J Med Virol. 2020;92(7):714–5. https://doi.org/10.1002/jmv.25815 .
Leung C. Comment on Li et al: COVID-19 patients’ clinical characteristics, discharge rate, and fatality rate of meta-analysis. J Med Virol. 2020;92(9):1431–2. https://doi.org/10.1002/jmv.25912 .
Li LQ, Huang T, Wang YQ, Wang ZP, Liang Y, Huang TB, et al. Response to Char’s comment: comment on Li et al: COVID-19 patients’ clinical characteristics, discharge rate, and fatality rate of meta-analysis. J Med Virol. 2020;92(9):1433. https://doi.org/10.1002/jmv.25924 .
Download references
Acknowledgments
We thank Catherine Henderson DPhil from Swanscoe Communications for pro bono medical writing and editing support. We acknowledge support from the Covidence Team, specifically Anneliese Arno. We thank the whole International Network of Coronavirus Disease 2019 (InterNetCOVID-19) for their commitment and involvement. Members of the InterNetCOVID-19 are listed in Additional file 6 . We thank Pavel Cerny and Roger Crosthwaite for guiding the team supervisor (IJBN) on human resources management.
This research received no external funding.
Author information
Authors and affiliations.
University Hospital and School of Medicine, Universidade Federal de Minas Gerais, Belo Horizonte, Minas Gerais, Brazil
Israel Júnior Borges do Nascimento & Milena Soriano Marcolino
Medical College of Wisconsin, Milwaukee, WI, USA
Israel Júnior Borges do Nascimento
Helene Fuld Health Trust National Institute for Evidence-based Practice in Nursing and Healthcare, College of Nursing, The Ohio State University, Columbus, OH, USA
Dónal P. O’Mathúna
School of Nursing, Psychotherapy and Community Health, Dublin City University, Dublin, Ireland
Department of Anesthesiology, Intensive Care and Pain Medicine, University of Münster, Münster, Germany
Thilo Caspar von Groote
Department of Sport and Health Science, Technische Universität München, Munich, Germany
Hebatullah Mohamed Abdulazeem
School of Health Sciences, Faculty of Health and Medicine, The University of Newcastle, Callaghan, Australia
Ishanka Weerasekara
Department of Physiotherapy, Faculty of Allied Health Sciences, University of Peradeniya, Peradeniya, Sri Lanka
Cochrane Croatia, University of Split, School of Medicine, Split, Croatia
Ana Marusic, Irena Zakarija-Grkovic & Tina Poklepovic Pericic
Center for Evidence-Based Medicine and Health Care, Catholic University of Croatia, Ilica 242, 10000, Zagreb, Croatia
Livia Puljak
Cochrane Brazil, Evidence-Based Health Program, Universidade Federal de São Paulo, São Paulo, Brazil
Vinicius Tassoni Civile & Alvaro Nagib Atallah
Yorkville University, Fredericton, New Brunswick, Canada
Santino Filoso
Laboratory for Industrial and Applied Mathematics (LIAM), Department of Mathematics and Statistics, York University, Toronto, Ontario, Canada
Nicola Luigi Bragazzi
You can also search for this author in PubMed Google Scholar
Contributions
IJBN conceived the research idea and worked as a project coordinator. DPOM, TCVG, HMA, IW, AM, LP, VTC, IZG, TPP, ANA, SF, NLB and MSM were involved in data curation, formal analysis, investigation, methodology, and initial draft writing. All authors revised the manuscript critically for the content. The author(s) read and approved the final manuscript.
Corresponding author
Correspondence to Livia Puljak .
Ethics declarations
Ethics approval and consent to participate.
Not required as data was based on published studies.
Consent for publication
Not applicable.
Competing interests
The authors declare no conflict of interest.
Additional information
Publisher’s note.
Springer Nature remains neutral with regard to jurisdictional claims in published maps and institutional affiliations.
Supplementary Information
Additional file 1: appendix 1..
Search strategies used in the study.
Additional file 2: Appendix 2.
Adjusted scoring of AMSTAR 2 used in this study for systematic reviews of studies that did not analyze interventions.
Additional file 3: Appendix 3.
List of excluded studies, with reasons.
Additional file 4: Appendix 4.
Table of overlapping studies, containing the list of primary studies included, their visual overlap in individual systematic reviews, and the number in how many reviews each primary study was included.
Additional file 5: Appendix 5.
A detailed explanation of AMSTAR scoring for each item in each review.
Additional file 6: Appendix 6.
List of members and affiliates of International Network of Coronavirus Disease 2019 (InterNetCOVID-19).
Rights and permissions
Open Access This article is licensed under a Creative Commons Attribution 4.0 International License, which permits use, sharing, adaptation, distribution and reproduction in any medium or format, as long as you give appropriate credit to the original author(s) and the source, provide a link to the Creative Commons licence, and indicate if changes were made. The images or other third party material in this article are included in the article's Creative Commons licence, unless indicated otherwise in a credit line to the material. If material is not included in the article's Creative Commons licence and your intended use is not permitted by statutory regulation or exceeds the permitted use, you will need to obtain permission directly from the copyright holder. To view a copy of this licence, visit http://creativecommons.org/licenses/by/4.0/ . The Creative Commons Public Domain Dedication waiver ( http://creativecommons.org/publicdomain/zero/1.0/ ) applies to the data made available in this article, unless otherwise stated in a credit line to the data.
Reprints and permissions
About this article
Cite this article.
Borges do Nascimento, I.J., O’Mathúna, D.P., von Groote, T.C. et al. Coronavirus disease (COVID-19) pandemic: an overview of systematic reviews. BMC Infect Dis 21 , 525 (2021). https://doi.org/10.1186/s12879-021-06214-4
Download citation
Received : 12 April 2020
Accepted : 19 May 2021
Published : 04 June 2021
DOI : https://doi.org/10.1186/s12879-021-06214-4
Share this article
Anyone you share the following link with will be able to read this content:
Sorry, a shareable link is not currently available for this article.
Provided by the Springer Nature SharedIt content-sharing initiative
- Coronavirus
- Evidence-based medicine
- Infectious diseases
BMC Infectious Diseases
ISSN: 1471-2334
- General enquiries: [email protected]
- français
- español
- português
Related Links
Rapid qualitative research to increase covid-19 vaccination uptake: a research and intervention tool.

View Statistics
Description, document number, collections.
- Technical documents
Show Statistical Information
- 5. Regional Office for Europe
Click through the PLOS taxonomy to find articles in your field.
For more information about PLOS Subject Areas, click here .
Loading metrics
Open Access
Peer-reviewed
Research Article
Public decisions about COVID-19 vaccines: A UK-based qualitative study
Roles Conceptualization, Data curation, Formal analysis, Funding acquisition, Investigation, Methodology, Project administration, Resources, Writing – original draft, Writing – review & editing
* E-mail: [email protected]
Affiliations School of Psychology, Swansea University, Swansea, Wales, United Kingdom, Department of Medical Social Sciences, Feinberg School of Medicine, Northwestern University, Chicago, Illinois, United States of America

Roles Conceptualization, Funding acquisition, Investigation, Methodology, Writing – review & editing
Affiliations Manchester Centre for Health Psychology, University of Manchester, Manchester, United Kingdom, Manchester University NHS Foundation Trust, Manchester Academic Health Science Centre, Manchester, United Kingdom, NIHR Greater Manchester Patient Safety Translational Research Centre, University of Manchester, Manchester, United Kingdom
Roles Conceptualization, Data curation, Formal analysis, Funding acquisition, Investigation, Methodology, Project administration, Writing – review & editing
Affiliations School of Psychology, Swansea University, Swansea, Wales, United Kingdom, Manchester Centre for Health Psychology, University of Manchester, Manchester, United Kingdom
Roles Conceptualization, Supervision, Writing – review & editing
Affiliation University of Sussex, School of Psychology, Falmer, United Kingdom
Roles Conceptualization, Writing – review & editing
Affiliation Independent Researcher, Kassel, Germany
- Simon N. Williams,
- Christopher J. Armitage,
- Kimberly Dienes,
- John Drury,
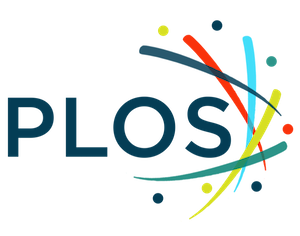
- Published: March 6, 2023
- https://doi.org/10.1371/journal.pone.0277360
- Peer Review
- Reader Comments
To explore UK public decisions around whether or not to get COVID-19 vaccines, and the facilitators and barriers behind participants’ decisions.
This qualitative study consisted of six online focus groups conducted between 15 th March and 22 nd April 2021. Data were analysed using a framework approach.
Focus groups took place via online videoconferencing (Zoom).
Participants
Participants (n = 29) were a diverse group (by ethnicity, age and gender) UK residents aged 18 years and older.
We used the World Health Organization’s vaccine hesitancy continuum model to look for, and explore, three main types of decisions related to COVID-19 vaccines: vaccine acceptance, vaccine refusal and vaccine hesitancy (or vaccine delay). Two reasons for vaccine delay were identified: delay due to a perceived need for more information and delay until vaccine was “required” in the future. Nine themes were identified: three main facilitators (Vaccination as a social norm; Vaccination as a necessity; Trust in science) and six main barriers (Preference for “natural immunity”; Concerns over possible side effects; Perceived lack of information; Distrust in government;; Conspiracy theories; “Covid echo chambers”) to vaccine uptake.
In order to address vaccine uptake and vaccine hesitancy, it is useful to understand the reasons behind people’s decisions to accept or refuse an offer of a vaccine, and to listen to them and engage with, rather than dismiss, these reasons. Those working in public health or health communication around vaccines, including COVID-19 vaccines, in and beyond the UK, might benefit from incorporating the facilitators and barriers found in this study.
Citation: Williams SN, Armitage CJ, Dienes K, Drury J, Tampe T (2023) Public decisions about COVID-19 vaccines: A UK-based qualitative study. PLoS ONE 18(3): e0277360. https://doi.org/10.1371/journal.pone.0277360
Editor: Mohamed F. Jalloh, Centers for Disease Control and Prevention, UNITED STATES
Received: March 22, 2022; Accepted: October 26, 2022; Published: March 6, 2023
Copyright: © 2023 Williams et al. This is an open access article distributed under the terms of the Creative Commons Attribution License , which permits unrestricted use, distribution, and reproduction in any medium, provided the original author and source are credited.
Data Availability: Ethics was approved by Swansea University’s Department of Psychology Ethics Committee. As part of the ethics review process, participant confidentiality restrictions prohibit the authors from making the data set publicly available. During the consent process, participants were explicitly guaranteed that the data would only be seen my members of the study team. For any discussions about the data set please contact Swansea University’s Research Governance: [email protected] .
Funding: This research was supported by the Manchester Centre for Health Psychology based at the University of Manchester (£2000) and Swansea University’s ‘Greatest Need Fund’ (£3000). This research was supported by the Manchester Centre for Health Psychology based at the University of Manchester (£2000) and Swansea University’s ‘Greatest Need Fund’ (£3000). The funders had no role in study design, data collection and analysis, decision to publish, or preparation of the manuscript.
Competing interests: CJA is supported by NIHR Manchester Biomedical Research Centre and NIHR Greater Manchester Patient Safety Translational Research Centre. This does not alter our adherence to PLOS ONE policies on sharing data and materials. JD sits on SAGE SPI-B subgroup, and Independent Sage. TT currently works for the World Health Organization, but contributed to this paper as an independent researcher. This does not alter our adherence to PLOS ONE policies on sharing data and materials.” (as detailed online in our guide for authors http://journals.plos.org/plosone/s/competing-interests The authors have no other relationships or activities that could appear to have influenced the submitted work.
Introduction
Vaccine hesitancy is a complex and multifaceted problem, and one that is influenced by a range of contextual (e.g. historical, institutional, political) factors, as well as individual-level and vaccine specific factors (e.g. costs or design of a given vaccination program) [ 1 ]. Individual-level factors, include health-system and providers, knowledge and beliefs about health and prevention, personal perceptions about risk versus benefit and personal and family experiences with vaccination (including pain and side effects from past vaccines) [ 1 , 2 ]. Although they are shaped by contextual factors, this research is primarily interested in individual perceptions of UK residents around the decision to get vaccinated against COVID-19.
Vaccine hesitancy can be defined as “the delay in acceptance or refusal of vaccination despite availability of vaccination services” [ 2 ]. In this paper, we draw on The World Health Organization’s (WHO) SAGE Working Group on Vaccine Hesitancy’s ‘continuum of vaccine hesitancy’ model, which sees vaccine views to be set on a continuum between full acceptance of vaccines with no doubts, through to complete refusal with no doubts [ 1 , 2 ]. Vaccine hesitancy is seen as a heterogenous group in-between these diametric positions, including those who “delay” acceptance (i.e. do not get it when first offered or according to schedule).
Although the WHO continuum of vaccine hesitancy is a simple and useful heuristic to categorise individuals, especially in relation to individual-level perceptions or attitudes, it is important to acknowledge that these attitudes do not occur in a vacuum but are shaped and influenced by many social and contextual factors. Intentions and decisions around vaccine acceptance or refusal, and the reasons behind them, can also be understood in terms of the ‘5 C’s’ model of vaccine hesitancy. This model suggests that vaccine hesitancy is influenced by: Complacency —for example where the perceived risk of harm from the disease is low; convenience —for example where there are practical or logistical barriers to access vaccination; confidence —for example where there is a lack of trust in the safety or efficacy of the vaccine; communication –for example where misinformation can create distrust or confusion; and, context –for example certain social groups, including some ethnic minority communities might encounter additional barriers, including structural racism, which might affect vaccine uptake [ 1 , 3 ]. Another theoretical framework is the Behavioural and Social Drivers (BeSD) of vaccine uptake [ 4 ]. The BEsD framework suggests four main drivers of vaccination uptake: (1) people’s mental and emotional responses to vaccines ( thinking and feeling ); (2) social or group norms around vaccinations ( social processes ); (3) people’s willingness and intentions, or hesitancy, to get vaccinated ( motivation ); (4) contextual or structural barriers related to e.g costs or access ( practical issues ) [ 4 ]. It is important to note the inter-relatedness of the many drivers of vaccine acceptance or hesitancy. As such, a focus on individual level decisions or intentions around vaccines, as is the case in this study, needs to acknowledge the ways in which individual feelings, beliefs and motivations, are shaped by (and serve to shape) contextual and practical issues.
In terms of the reasons behind vaccine intentions and decisions, survey data on COVID-19 vaccine intentions and decisions suggests that most common reasons for vaccine hesitancy include: worries over side effects, worries over long term effects on health, as well as concerns over its efficacy [ 5 ]. Qualitative research on public views on COVID-19 vaccines is emerging. One study, from the UK, found that vaccine hesitancy was associated with three main factors: safety concerns, negative stories and personal knowledge, with those who were most confused, worried and mistrusting being the most hesitant [ 6 ]. Another study, from Canada, on overall attitudes to public health measures to reduce COVID-19 transmission found that many participants felt that vaccines were a means to “get back to normal life” while some were hesitant due to a lack of confidence in the potential efficacy of the vaccine and concerns over side-effects [ 7 ]. A study from Australia, with hesitant health or social care workers or clinically vulnerable adults, found that participants saw vaccination as beneficial for both individual and community protection, but also expressed safety concerns that made them feel like “guinea pigs” [ 8 ].
Ongoing research into vaccine hesitancy is needed to follow how attitudes and decisions around COVID-19 vaccines may be changing as the pandemic continues. Also, qualitative data can explore, in depth, the reasons behind why people are deciding to get vaccinated or not. In this paper, we explore participants’ decisions on COVID-19 vaccines in the UK during March and April 2021. For context, during this period the UK was experiencing a rapid roll out of COVID-19 vaccines (Astra Zeneca and Pfizer-BioNTech) via the National Health Service, with between January and 22nd April 2021, administered approximately 35 million total doses, with approximately 60% of the total population aged 16 and over having received at least one dose with doses being prioritised amongst older adults and those with certain underlying health conditions (clinically vulnerable and clinically extremely vulnerable adults) [ 9 ].
This paper explores participants’ intentions and decisions around whether or not to get vaccinated, and specifically the reasons behind them, thereby contributing to our understanding of the facilitators and barriers to vaccine uptake.
Materials and methods
Participants and data collection.
Data from this study came from the COVID Public Views (PVCOVID) study–a mixed-methods study using panel focus groups and surveys during the pandemic (commenced March 2020) [ 10 , 11 ]. Participants for the PVCOVID study were initially recruited to the study from March-July 2020, with a total of 53 participants initially enrolling into the study. Participants were all UK-based adults aged 18 years or older. Recruitment for the study took place primarily via non-probability, opportunity sampling. Recruitment included using social media advertising (Facebook ads and via posting ads on Twitter), other online advertising (e.g. online ‘free-ads’ such as Gumtree), as well as snowball sampling (e.g. asking participants who had taken part in a focus group to distribute the study ad to others they felt might be interested in participating). Recruitment sought as diverse a range of ages, genders, race/ethnicities, UK locations, and social backgrounds as possible (e.g. advertisements encouraged expressions of interest from individuals from Black and Asian Minority Ethnic (BAME) backgrounds; social media ads were designed to targeted users from across the UK and a wide age range). Although the study had a low number of individuals from older age groups (over 50 years of age) the over-representation of younger adults could be seen as beneficial because of their lower vaccination coverage [ 9 ].
Here we report on data from six online focus groups with 29 participants from within the overall PVCOVID study. Focus groups were not arranged according to any pre-existing views or decisions around vaccinations (i.e. we did not purposefully put those who were not intending on getting vaccinated in the same group for example). This was largely because of the longitudinal nature of the overall study, and our initial decision to try to keep the membership of each focus group the same over time in order to build rapport, familiarity and openness within the groups (we did not collect data on vaccine views during the initial recruitment and group allocation in March 2020). Each group contained a mixture of those who had already received at least one vaccine (n = 15) and those who had either already refused a vaccine or who were delaying their decision to get a vaccine (n = 14). One potential limitation of this focus group composition was that those who were refusing or delaying vaccination might have felt less comfortable expressing their opinion (since, generally getting vaccinated was seen by many as a social norm—see below). However, the focus group facilitator sought to ensure that all participants felt comfortable expressing their views, and that dialogue within the group was not hostile and as respectful and open as possible. Also, questions were phrased in non-leading, non-judgemental a way as possible.
In March 2021, participants were invited to take part in a rapid round of focus groups on the topic of vaccines. Participants took part in focus groups conducted between 15th March and 22nd April 2021. Further information about the participants discussed in the present paper are presented in Table 1 .
- PPT PowerPoint slide
- PNG larger image
- TIFF original image
https://doi.org/10.1371/journal.pone.0277360.t001
Online focus groups were necessary due to COVID-19 social distancing regulations, but have been seen to have benefits in general, as a means of eliciting public views from diverse and geographically dispersed participants [ 12 , 13 ]. Each focus group (of 4–6 participants) met virtually via the videoconferencing platform Zoom for approximately one hour. All focus groups discussed in the present paper were moderated by SW. Focus groups were recoded and transcribed. The topic guide for the focus groups (Appendix 1) was initially developed using existing literature on vaccination public attitudes and vaccine hesitancy discussed above, as well as rapidly emerging surveys on public attitudes to COVID-19 public attitudes.
Ethical approval was received by Swansea University’s School of Management Research Ethics Committee and Swansea University’s Department of Psychology Ethics Committee (Ref: 2020-4952-3957). All participants gave informed consent, both written and verbal. All data were kept securely and confidentially in line with ethical requirements, and where data is presented below, all quotes are anonymised to protect participants’ identities.
Data were analysed in accordance with a Framework Analysis (FA) approach [ 14 ]. FA is a flexible approach that is not aligned with a particular epistemological, philosophical or theoretical tradition. It can be either primarily inductive or deductive (or a combination thereof) and can be adapted with many qualitative approaches with the main aim being to generate themes [ 14 ]. Our use of FA combined elements of both induction and deduction in an abductive approach [ 15 , 16 ], whereby the researchers inductively coded for emergent facilitators and barriers to vaccine uptake as they emerged. The coding process was also broadly informed deductively by existing literature, including the WHO’s ‘continuum of vaccine hesitancy’ framework. Using the vaccine hesitancy continuum model [ 1 ], we analysed data to look for, and explore, three main types of vaccine decisions in the data: those who had accepted, or were planning on accepting, the vaccine; those who had refused, or were planning on refusing, the vaccine; and those who had not yet decided, or were delaying the decision of, whether or not to get the vaccine. As such, each participant was coded into one of these three main categories based on their decision or intention (e.g. statements that indicated they were not sure if they wanted a vaccine were coded into three main categories: accept, delay, refuse. Sub-coding sought to bring out the nuances within these simplified intention/decision types (e.g. “accept but unsure”). Inductive analysis was used to explore facilitators and barriers to vaccine acceptance (i.e. the reasons why people were getting or intending on accepting or refusing a vaccine or why they were unsure of or delaying their decision).
Two authors (SW and KD) primarily analysed the data, in consultation with the other three authors (CA, JD, TT). The first stage of FA is data familiarisation . SW and KD independently read three focus group transcripts initially, and independently assigned preliminary codes to specific lines or chunks of texts. The two authors met regularly (after each transcript) to identify commonalities and differences in their coding and discussed frequent or significant codes which were beginning to form emergent themes–i.e. patterns in the data, codes clustering around a common concept (e.g. ‘preference for natural immunity’) [ 16 , 17 ]. Following this, the third stage– data indexing– was performed, through which the two analysists systematically applied the thematic framework and the existing list of codes to the remaining transcripts. As FA is a flexible, iterative approach, new codes, and new themes (clusters of codes) were developed as they emerged. The fourth stage, data charting , enabled the analysts to refine, rearrange and focus (data reduction) on what they deemed the most prevalent and salient themes. The final stage– data mapping– enabled the analysts to review the main themes and organise them in relation to the study’s main objective–identifying facilitators and barriers to COVID-19 vaccine uptake using the accounts of the participants intentions and decisions around vaccines. The other three authors (CA, JD, TT) were not involved primarily in the data analysis but were consulted throughout the FA–and provided input into code definition and theme generation, they also provided comments which were used to resolve any disagreements between SW and KD’s coding.
Vaccine acceptance
One type of response, those who had already accepted or planned to accept (when they were offered one), a COVID-19 vaccine, held largely positive views around COVID-19 vaccines. In line with the vaccine hesitancy continuum [ 1 ], those accepting the vaccines fell into two subtypes: those who expressed little to no reservation about their decision to accept a vaccine (“full acceptance”) and those who had accepted, or were intending to accept, a vaccine, but who also expressed concerns or reservations about their decision (“accept but unsure”).
Those fully accepting tended to frame their decision as something that was quite “normal” to them (“I’ve had all my vaccines”—see vaccination as a social norm below):
“I had no problems getting the vaccines or any concerns about the efficacy of the vaccines … It was something I need to do to protect my family and loved ones … It was an easy decision to make, I’ve had all my vaccines all my life, because I see the value.” (Participant 44, Female 30s)
Those fully accepting also framed vaccination as a collective responsibility, and their decision as something that would “protect” others as well as themselves (especially vulnerable others) (“I don’t want to hurt anyone else” (Participant 19, Male, 20s)). This included some who saw themselves as not necessarily being at risk personally:
“I had my first one [vaccine dose] about a month ago … I was practically queuing up as soon as I heard … My biggest thing throughout the whole pandemic to protect others, not necessarily myself, because I’ve never seen myself as being particularly at risk but now, I am statistically less likely to catch and pass it on” (Participant 6, Male, 20s)
However, although some of those accepting were confident in their decision, others expressed concerns in spite of ultimately deciding to get vaccinated (akin to the Vaccine Hesitancy Continuum’s ‘accept but unsure’ vaccine attitude group) [ 1 , 2 ]:
“I was a bit suspicious. I suppose it’s only natural, everyone’s a little worried because you think it’s only been done in a year, but the science today compared to years ago is outstanding isn’t it, so they have more methods of testing to see if its effective, so I wasn’t really worried, and I’m glad I have got it” (Participant 42, Female 30s)
Those accepting a vaccine—including those in the “accept but unsure” category–expressed and emphasised a number of facilitators, including a trust in science and vaccination as a necessity (see below).
Vaccine refusal
A second type of response was from those who had already refused, or who were planning on refusing, a COVID-19 vaccine. Unlike those who were unsure of, or who were delaying, their decision of whether or not to get a vaccine (see below), those refusing explicitly stated that they were not going to have the vaccine (at least not for the “foreseeable future”):
“I’ve not had the vaccine and, to be honest with you, I don’t have any intention of getting the vaccine like in the foreseeable future, I just don’t see the point”. (Participant 3, Male, 20s)
Those refusing the vaccine tended to frame vaccination in terms of individual rather than collective responsibility; and as a personal choice (“It’s a choice I’ve made” (Participant 8, Female, 40s)). Those refusing were also less likely than others to frame their vaccination as something that would protect others and saw it as something that was primarily useful for those most vulnerable from serious COVID-19-related illness, for example older age groups (see preference for natural immunity below). Those refusing also emphasised the three main barriers, including a preference for natural immunity , concerns over side effects and distrust in government (see below).
Vaccine delay
A third type of response was from those who were still unsure of, or were delaying, the decision of whether or not to get the vaccine. These participants can be distinguished from those who had already made up their mind that they did not want to get vaccinated. These participants felt they needed more time to decide. Some of those delaying had already turned down or ignored their vaccination appointment invitations. However, they characterized their current position as subject to change–that is they did not want the vaccine yet but were aware that they might in future:
“I wouldn’t take it straight away… I’d rather just wait and make my own decision at my own pace really” (Participant 15, Male, 20s)
Some of those delaying tended to emphasise a need for more information about the efficacy and safety of the vaccine as a main reason for the delay in their decision (see perceived lack of information below) with some feeling they were being “pressured” (e.g. by the vaccination campaign and offer) into having to make a decision too quickly:
“I’m still holding out. I’ve been sent several letters and loads of text messages telling me that I needed to go for the vaccination, but I would rather wait at least four months to see exactly where it is all going… I don’t want to be sort of pressured into something that I might regret” (Participant 15, Male, 40s)
Like those refusing the vaccine, those delaying their decision were also concerned about the speed at which vaccines had been developed and were concerned over the possible longer-term side effects (see concerns over possible side effects below):
“I am a little bit nervous because I do feel like it’s all been rushed through incredibly quickly … have they done all the tests? I wanted to ensure that a lot more people had it before it was my turn, because I kind of figured that if there’s a problem, we’ll know” (Participant 50, Female, 50+)
Despite the feeling of “pressure”, some participants delaying tended to perceive themselves as having agency in their choice of whether to get vaccinated. However, others delaying tended to argue they felt as though they would be “forced to do it” (Participant 7, Female 20s):
“I don’t intend on taking it, unless it’s a must, unless, I heard you have [a vaccine] to go on holidays, but right now I am going to refuse it, until the very last moment. But I know at some point I will have to take it” (Participant 18, Female, 30s)
These participants tended to be quite hesitant about the idea of getting a COVID-19 vaccine but believed that they would “have” to get one in order to be able to engage in certain activities in future, particularly traveling internationally. Here, vaccination tended to be viewed as a personal choice rather than in terms of collective responsibility (“I have the say and right what I should accept into my body and nobody else should have a say” (Participant 22, Female, 20s)). They tended to perceive themselves as having little or no agency in regard to whether or not they would ultimately have a vaccine. The act of delaying may therefore be construed as a way to preserve agency while they could, or perhaps to recapture a sense of control over their lives that many felt they had lost during the pandemic [ 10 ].
Facilitators: Reasons for vaccine acceptance
Vaccination as a social norm..
Social norms are a means through which health behaviour and decisions can be influenced during the pandemic [ 18 ]. Those accepting a vaccine tended to discuss COVID-19 vaccination in relation to a personal willingness to be vaccinated in the past and in general (“I’ve had vaccines all my life for what is needed” (Participant 12, Male, 40s)), as well as in relation to a culture (the UK) within which vaccines were normative (including the fact that vaccinations were a “part of normal life” from a young age):
“For me, I’ve never been one to say no to a vaccine. Its part and parcel—as a child you are given various vaccines, and you don’t necessarily have a choice, but then when you have your boosters, you just go ahead and do it and to me that’s part of normal life” (Participant 10, Male, 40s)
For some, vaccination for COVID-19 was becoming more normative over the course of the pandemic. For some, hesitancy was decreasing, as more people started to get vaccinated, partly as a way to help get back to “normality” (see vaccination as a necessity ):
“Talking to people there’s a lot of people have taken it [the vaccine] and I’m surprised. They had said initially that they weren’t going to take it … [but] there’s just a lot of impatience and frustration at the minute you know people just want to get back to some sort of normality” (Participant 8, Female, 40s)
Others felt as though there was a pressure to get vaccinated, for example within some workplaces:
“Initially when it [vaccine] came out a lot of the [front line-workers] on my team were like ‘nope, not going to get it’ but that changed one-by-one, they started to go for it, and now I think there is only a handful who haven’t gone for it … its being monitored by senior management but they have got to ask have you had it or not, they are not asking why you haven’t had it, but I don’t know if that is something at some point, you know you have to justify your grounds as to why you haven’t” (Participant 5, Female, 30s)
For this participant, in their workplace, there was an implied expectation that they would, in the future, need to get the vaccine or justify why they hadn’t. This informal scrutiny of vaccine uptake may be functioning as an example of ‘norm-nudging’ [ 18 ]. In this participant’s workplace, although vaccination was not obligatory or coercive, and the reasons for ‘opting-out’ were not required, the implication was that opting-in was the default or normative position. The expectation that justification for opting out would be necessary in the future may have led to the change in decision (i.e. individuals opting to get vaccinated now, despite not initially wanting to, because they felt they would either be required to in the future or would have to justify their decision not to).
Vaccination as a necessity.
Vaccination as a social norm closely related to a second facilitator–vaccination a necessity. Participants discussed three main ways in which vaccination would be necessary or inevitable. These were framed either positively or negatively (related to whether participants were accepting of vaccines or not).
Firstly, those accepting a vaccine tended to refer to vaccination as being the way for them to “get back to normal” (Participant 20, Male, 20s) or “the only way forward, to get on with our lives” (Participant 12, Male, 40s). Secondly, a number of participants, including those accepting a vaccine and those delaying their decision, described an “acceptance” of the fact that they would need regular vaccinations or ‘boosters’ as a result of the continued emergence of new variants of the virus:
“My parents and I have already accepted that we will require boosters, they will be a fact of life, probably for the next four or five years, and it will be the same as our flu jab that we get every year” (Participant 2, Male, 40s)
In particular, concerns over new and emerging variants were cited as a reason as to why vaccination was not perceived to be a “one-off”, and so “regular” vaccination was seen to be necessary with “no other way out”.
“The virus is going to keep mutating, there is going to be different variants, I think that’s why the government is so hot on this vaccine because it is not going to stop. … It’s something that we’re going to have to live with this is not going to die out anytime soon… there is no other way out … we are going to have to go for our top-ups with the vaccine” (Participant 5, Female, 30s)
It is worth noting however, that refusers saw the possibility of regular vaccination as a deterrent to getting vaccinated currently:
“There’s a concern that, if one takes the vaccine have a variant of a new variant emerges. That could be resistant to the vaccine and then there’s a question of do you have to keep taking vaccines to protect yourself against each single various oh I don’t really have any intention of getting the vaccine” (Participant 3, Male, 20s)
Thirdly, immunity certificates or “vaccine passports” were also cited by some participants as a potential facilitator to vaccine uptake. One way in which they were framed was as a “necessary evil”—as something that were, begrudgingly, needed to help “save” the economy:
“I think it’s just it’s almost a necessary evil and it’s the way we’re going to go … I think it’s also going to save a lot of businesses and that will save you know; the economy and we all need the economy to be boisterous we all need a prominent economy”. (Participant 2, Male, 40s)
However, vaccine passports were a complex and controversial topic in the focus groups. Some delayers felt that vaccine passports were a reason why they would ultimately be “forced into” get a vaccine:
“I’m two minds really about whether to get the vaccine or not but I have a feeling people will be pressurised into having it through the vaccine passports or certificates, whether that could be for traveling or getting jobs, so I think people will be indirectly forced into getting it” (Participant 7, Male, 40s)
Trust in vaccine science.
Accepters expressed a higher trust in science, and to frame science as being relatively independent from government:
“I chose to be vaccinated because, not that I trust the government, but I trust the medicine and the science behind it. It’s not the government that produces the vaccine.” (Participant 11, Male, 50+)
Accepters were also more likely than others to associate the vaccination program with the health service (NHS):
“I know that the vaccine was developed really quickly … but I have faith in the health system and its testing” (Participant 10, Male, 40s)
Although some accepters expressed some concern at the speed at which the vaccines had been developed, they tended to contextualise this in terms of the fact that science was now more advanced than with many previous vaccines:
“I was a bit suspicious; I suppose it’s only natural, everyone’s a little worried because you think it’s only been don’t in a year, but the science today compared to years ago is outstanding isn’t it, so they have more methods of testing to see if its effective, so I wasn’t really worried, but I’m glad I have got it” (Participant 25, Female, 30s)
They also contextualised the speed of vaccine development in terms of what they perceived as rigorous testing, and the fact that there had been considerable scientific, medical and financial focus and investment in the vaccine development. This for them meant any potential risks from unforeseen issues due to safety or efficacy were likely to be minimal and outweighed by the benefits of the vaccination program:
“I believe it’s been so rigorously tested and it’s the only thing spoken about medically for the last 12 months and so the risk for me was just so minimal and benefits outweighed any risk for me” (Participant 6, Male, 20s)
Barriers: Reasons for vaccine delay or refusal
Preference for ‘natural immunity’..
Some participants argued that one of the reasons they were either hesitant about or did not want to get vaccinated was because they preferred to “fight” the virus “naturally”:
“For me personally I am not sure I would go for the vaccine… I just hope I have a strong immune system so I can fight the virus. We have this in-built immune system within our bodies…give them a chance to operate” (Participant 7, Male 40s)
In discussing their decision, refusers tended to frame COVID-19 as a disease which tended not to affect young and “healthy” people:
“I don’t have any intention of getting the vaccine in the foreseeable future, I just don’t see the point, because the virus is mainly fatal to those who are, like middle aged. (Participant 3, Male, 20s)
In doing so, they also emphasised their own healthiness as a reason as to why they didn’t need the vaccine, and drew comparisons to the fact they hadn’t needed vaccines in the past for other diseases:
“It just does not make sense to me to take a vaccine, it’s like a flu vaccine. I’ve never ever taken a flu vaccine, because I don’t get the flu.” (Participant 8, Female, 40s)
As noted above, refusers framed vaccination as an individual act rather than a collective act, and argued that the lack of personal benefit was outweighed by the perceived risks posed from potential side effects:
“I have no intentions of taking it, and I have focused a lot on my health over the years, I’m the healthiest I’ve ever been and I just don’t see I don’t the reason for me to take it … because from what I’ve read, there are risks with it so.” (Participant 8, Female, 40s)
Concerns over possible side effects.
One of the main reasons for vaccine hesitancy was a concern over side effects–something that accounted for why some people were delaying the decision to get vaccinated:
“I probably will have it [a vaccine], but I want to wait to see if people turn into zombies first. I’ll wait until a few hundred thousand have had it first” (Participant 28, Female, 20s).
Although the above quote was tongue-in-cheek, it was indicative of a wider concern over potentially unforeseen, longer-term, side effects. Delayers tended to frame these concerns in relation to what they knew about how vaccines were “normally” developed. They discussed how comparatively quickly COVID-19 vaccines had been developed and emphasized how not enough time had passed to be able to know long-term side effects:
“I do have like some concerns about how quickly they developed this vaccine, because most vaccines take like you know six five to six years to test and to make sure that you know they’ve seen all the side effects. But with this vaccine I still have that reservation that maybe it’s been too quick, and they’ve not really teased out all the long-term effects.” (Participant 3, Male, 20s)
Other delayers focused on short-term side effects or risks. In particular, recent reports of potential blood clots linked to vaccines were cited by a number of delayers. Interestingly, these participants were aware that any causal link between blood clots and vaccines had not been clearly demonstrated, or that any potential risk between vaccines and blood clots was considerably small. Nevertheless, they continued to cite blood clots as a cause for concern and framed their hesitancy in relation to it as an example of potential side effects (including unknown side effects that may emerge in the future):
“Right now, I am going to refuse it, until the very last moment. I feel like I’m a guinea pig. I don’t know if you heard the news that they have stopped one of the vaccines because there has [sic] been cases of blood clots of something. I know it’s a very, very, very, very tiny percentage but I feel like if I wait till the very last more [information] can come out”. (Participant 9, Female, 30s)
Some participants distinguished between vaccines, with those who did expressing personal concern, or observed concern in others, over the vaccine manufactured by the company AstraZeneca, which had been the focus of blood clot controversies in the media. For example, one participant, who was vaccine hesitant, stated that although they accepted the Pfizer vaccine (i.e. the vaccine manufactured by Pfizer-BioNTech), they would not have accepted the AstraZeneca vaccine:
“Now I’ve had it I feel okay about it, but I think that’s because with the Pfizer it doesn’t seem to be any negative reports, whereas with AstraZeneca there seems to be a lot of mixed communications, and I don’t think there is a lot of fault with the vaccine, it’s just I don’t think the company is very good at kind of being truthful and that makes people a bit doubtful … I don’t think I would have done it [had the AstraZeneca vaccine] (Participant 24, Female, 50+).
Another participant described how they had heard of others specifically opting out of vaccination, once they discovered they were to get the AstraZeneca vaccine:
“My aunt had hers and she said there was a huge queue in the surgery… but every single person that was offered the one that begins with an A [AstraZeneca] were actively declining it and walking out and she witnessed in ten minutes about 15 people turning up, being told what they were getting and walking out” (Participant 21, Female, 20s)
On the other hand, accepters, including those who had taken the vaccine with reservations (cf. The Vaccine Hesitancy Continuum’s [ 1 , 2 ] ‘accept but unsure’ group), were much less concerned over both short- and long-term side effects. They framed the issue in terms of a cost-benefit analysis, where for them, the perceived benefits (e.g. reducing the risk of “getting long Covid”) outweighed any potential risk of unknown, long-term side effects:
“I’m quite worried about, not any side effects now , but like maybe in ten years’ time … but then it seems like the risk from the vaccine is less than the risk of say getting long Covid … so the vaccine is the lesser of two evils” (Participant 24, Female, 50+)
They also made comparisons to what they saw as other equally rare side effects of common medications:
“I know there is this whole issue around blood clots, but people really need to get a grip, because you know, people die on a yearly basis from taking paracetamol, plenty of women die from blood clots as well …from taking the pill … that so the benefits outweigh the risks most definitely” (Participant 12, Male, 40s)
Perceived lack of information.
A perceived lack of information was a major factor for why some participants were either refusing or delaying vaccination:
“Whatever is going on with the vaccine, I don’t know, it really is a minefield of information” (Participant 8, Female, 40s)
Questions were scientific in nature and stemmed from the fact that COVID-19 was still such a new disease. For example, one participant (a refuser) questioned whether they would need to “keep taking” vaccines due to new variants (potentially “resistant” to the previous vaccines), something that deterred them from accepting their initial vaccine offer:
“There’s a concern that, if one takes the vaccine and a variant of a new variant emerges that could be resistant to the vaccine then there’s a question of do you have to keep taking vaccines to protect yourself against each single variant? I don’t really have any intention of getting the vaccine.” (Participant 3, Male, 20s)
Another participant (a delayer) questioned whether people still “need” a vaccine following infection with COVID-19:
“I am not against the vaccine, [but] for me there are so many unknowns, because It is so new there are so many questions that I want to ask, like if you have had Covid do you still need, in terms of the antibodies you have, or do you still need the protection from the vaccine? I’m very much in the middle, so once I get those answers, I will be leaning more towards getting it” (Participant 5, Female, 30s)
Distrust in government.
Refusers and some delayers tended to have less confidence in vaccine science and less trust in government (“I think a lot of my concerns are because of the government because I just don’t trust them at all” (Participant 29, Female, 50+)). They framed COVID-19 vaccine science as being closely linked to, or even compromised by, political or economic interests. Some justified their distrust in relation to historical controversies or examples of medical iatrogenesis:
“The government doesn’t have a very good track record with the sense that there was the Thalidomide tablets that were given to pregnant women back in the ‘60s, the blood transfusions that were imported from people volunteers in state penitentiaries in America that were contaminated, brought into the United Kingdom.… there’s been several vaccinations given to toddlers … that came from America—I think about 1000 children have died…. I’m not one to trust governments, they tend to rush into things.” (Participant 15, Male, 40s)
Although delayers generally recognised that the vaccines had been tested, they remained concerned or “sceptical” that testing had been done as extensively or for as long as was necessary. They tended to see vaccination as something that was still being tested (in the community):
“Even though there has been a lot of testing done, I still feel sceptical and quite scared to get it … technically it’s still in the testing phases even though it’s been approved, and so until I’m actually forced to do it, I don’t think I want to” (Participant 7, Female, 20s).
Although, as discussed above, some participants framed vaccine passports as a “necessary evil” to enable them to travel or to help the economy, others, including both refusers, framed them as “Orwellian” and argued that by using them, government were encroaching on their “freedom of choice” (Participant 13, Male, 40s) and “privacy”:
“[I’m] a hundred per cent against vaccine passports, I personally would rather just have the PCR [COVID-19 polymerase chain reaction] tests. … it is somewhat Orwellian … I ’m very, very concerned about things like surveillance and privacy” (Participant 3, Male, 20s)
Conspiracy theories and misinformation.
Those refusing a vaccine, and some of those delaying, linked their distrust in government to what they saw as conspiracies (things that “don’t seem to add up”), such as the perception that vaccines were driven by the “agenda” of the pharmaceutical companies involved in manufacturing them, in order to make profit.
“I mean distrust in government … the things that don’t seem to add up. I mean we have got the pharmaceutical companies, several of them creating a vaccine, some kind of race … and it’s just a win-win for them, if just everyone gets a vaccine and people can’t think for themselves … it a big agenda” (Participant 7, Male, 40s)
It is important to emphasise that, to the participants themselves, these were seen as plausible conspiracies.
Amongst the Black and Asian Minority Ethnic (BAME) participants in this study, most were critical of the circulation of conspiracy theories. However, many of them did also discuss how conspiracy theories and misinformation was quite prevalent in their communities (“It’s weird how it afflicts the Black community in terms of social media and WhatsApp conspiracy theories in circulation” (Participant 2, Male, 40s)). Some related the lower uptake in vaccination amongst BAME groups to the existence of ‘folk wisdom’ about what might help promote health or even protect against COVID-19:
“A few months back India was number two in the number of cases and deaths from Covid and one fine day it just vanished. So, everyone is trying to ask what is being done differently in India… and I guess that mindset is being transferred to racial communities here [in the UK]. So people are discussing our [Indian] food habits, and we do eat a lot of spicy food and spices so I’ve actually seen people talking about saying that ‘ok it’s our food which is different… There are people even saying ‘avoid the vaccines, stick to your spices, your curries and you’ll be fine’. So that is worrying but that is a topic which is being widely discussed in our Asian community” (Participant 4, Male, 30s)
However, a number of BAME participants linked the lower uptake in their communities to a distrust in government (see above) and thus a distrust in the vaccines. One example was the rumour that vaccines were being “tested” for side effects first amongst BAME patients:
“I think just from my experience, there’s a lot of conspiracies that I’ve heard about. Because I mean I identify myself as a Black British person and so within my community I’ve heard a lot of just not trusting the vaccine … somebody sent me a video about [UK Government Health Secretary] Matt Hancock …suggesting that the vaccine was tested first amongst the BAME group.” (Participant 5, Female)
These participants also discussed the issue of distrust as a wider issue, accounting for why there “is some cynicism in these communities” (Participant 2, Male, 40s). This lack of trust was seen to stem from a lack of information (cf. lack of information above), which in turn was seen to be the result of a lack of engagement with BAME communities, as well as a perceived lack of government accountability for the disparities that BAME communities have experienced during the pandemic:
“I just think within our community there needs to be a lot more education, especially if there are a lot of unknown questions that haven’t been answered … that needs to be advertised more as to where we can go to ask those questions to be more equipped with the knowledge around these vaccines, rather than listen to these conspiracies which a lot of them is [ sic ] fake news…. A lot of it [lack of trust] stems from the government; a lot of how it [pandemic] has been handled is embarrassing, a high number of deaths were from the Black and Asian community and so that mistrust in government along with them not really putting their hands up just makes us even more anxious” (Participant 5, Female)
Covid ‘echo chambers’.
One potential barrier to uptake is what might be seen as the emergence of ‘Covid echo-chambers’. Echo chambers can be defined as “polarized communities populated by like-minded” others and can be found particularly in online settings [ 19 ]:
“I also interact quite a lot on the internet and a lot of people that I speak to on the internet, they say the same thing that you know they just have this concern about the vaccine.” (Participant 3, Male, 20s)
The existence of polarized views, on an emotionally charged subject led some participants to argue that “everyone is extreme in their reactions” (Participant 26, Female, 20s):
“It really divides those who do have the vaccine and those who don’t, and I see quite a lot on social media people who post like very critical things of people who are on the opposite side” (Participant 15, Male, 20s)
As a result, some delayers felt ‘as though they didn’t ‘fit’ into either of the polarized attitude groups on vaccines, which may have been contributing to their uncertainty or hesitancy around whether or not to get vaccinated:
“Its either the people who [say] ‘don’t have the vaccine, it’s got 5G in it and the government are going to follow you and nobody should have it’ or you’ve got the people going ‘I’ve had the vaccine, and everyone should have the vaccine and you are stuffing it up for the rest of us” (Participant 26, Male, 30s)
Echo chambers are discrete and are characterised by a lack of communication across them. In our study we found evidence of a lack of communication between individuals with differing views on vaccines, for example between accepters and refusers. For example, one participant, an accepter, described how having conversations with a family member about vaccines was difficult given the latter’s opposing views on Covid:
“I’m happy to get the vaccine …but one family member isn’t keen on the vaccine, because they are just not convinced of the coronavirus in the first place…. I’ve not had a discussion with them about the vaccine because of his views on coronavirus in general” (Participant 6, Male, 20s)
Similarly, another participant, a refuser, described how she was reluctant to discuss the subject of vaccination with others, particularly those who she knew, or thought, may be in have strongly ‘pro-vaccination’ views:
“I don’t really bring it up now in conversation now with anyone … I don’t want to get into discussions. … I do find there is a lot more of two extreme sides (Participant 8, Female, 40s).
In keeping with the ‘continuum of vaccine hesitancy’ model [ 1 , 2 ], we found three main groups of participants, based on their decision or intention to receive a COVID-19 vaccine: Those who had accepted, or were planning on accepting, the vaccine; those who had refused, or were planning on refusing, the vaccine; and those who had not yet decided, or were delaying the decision of, whether or not to get the vaccine. In order to explain these different attitudes, we identified three facilitators (Vaccination as a social norm; Vaccination as a necessity; Trust in science) and six barriers (Preference for “natural immunity”; Concerns over possible side effects; Perceived lack of information; Distrust in government; Conspiracy theories; Covid echo chambers) to vaccine uptake. Although data on actual and eventual vaccine coverage changes rapidly [ 10 ], this study provides an in-depth account during the initial roll-out of the vaccines in the UK (during March-April 2021) into people’s decision-making process, including the reasons behind the decision to accept or refuse a vaccine and any hesitancy experienced.
The concept of ‘vaccine delay’ [ 1 , 2 ] can therefore be a useful concept (as a form of hesitancy, along with refusal) through which to understand why some are reluctant or unsure as to whether or when they will receive a COVID-19 vaccine, since many of those who were unsure, characterised their decision as one that was ongoing. We found two main reasons for the existence of vaccine delay. Firstly, some were delaying due to a perceived need for more information. Secondly, others were delaying until they were “required” to be vaccinated.
Our findings can be understood in relation to broader conceptual models of vaccine hesitancy, including the ‘Five C’s’ model [ 3 ] and the WHO SAGE Working Group on Vaccine Hesitancy Determinants Matrix [ 2 ], specifically to the case of COVID-19. Our findings suggest that, as per the ‘Five C’s’ model, particularly Confidence, and Complacency were major factors explaining vaccine hesitancy (concerns over convenience were not apparent in our study, perhaps due to the fact that at the time of data collection, the UK was seeing a rapid and lauded vaccine roll-out via its National Health Service (NHS) (the UK had between January and 22nd April 2021, administered approximately 35 million total doses, with approximately 60% of the total population aged 16 and over having received at least one dose [ 10 ]). For example, ‘confidence’ in the efficacy and safety of the vaccines was a major facilitator or barrier depending on a person’s perspective, especially in relation to the extent to which they trusted science or government (and the extent to which they saw the latter as influencing the former) [ 20 ]). Also, ‘complacency’ accounted for why some delayers and refusers were reluctant to be vaccinated. This complacency took the form of a perception of low personal risk and a valuing of “natural immunity” and might be better thought of as a form of ‘lay knowledge’ or ‘medical folk wisdom’ [ 21 , 22 ]. As such, rather than constructing it as ‘complacency’ per se, it might be more useful to understand how perceptions of low personal risk can be offset by constructing vaccination as a ‘collective responsibility–one that even those at relatively low personal risk from serious COVID-19 illness should do to protect others.
The Vaccine Hesitancy Determinants Matric holds that contextual, individual and group, and vaccine-specific issues all impact the extent to which people are accepting of or hesitant towards vaccination [ 1 ]. For instance, vaccination as a social norm was found to be an important individual and group influence. We found that a major barrier in the context of COVID-19 is the existence of conspiracy theories and Covid echo chambers. Thus, reducing the circulation and belief in conspiracy theories will likely help control the spread of COVID-19 [ 23 ], including in this case through potentially increasing vaccine uptake, perhaps particularly amongst BAME communities. Research suggests that people may be drawn to conspiracy theories when they promise to satisfy epistemic (e.g. desire for certainty), existential (e.g. a desire for control) and social (e.g. a desire to ‘fit in’ within a group) motives [ 24 ]. BAME communities may be particularly at risk from a lack of knowledge and safety, because of their historical marginalization in society and because of the fact that morbidity and mortality from COVID-19 has been higher. Research suggests that experiences of ostracism, including due to an individual’s race or ethnicity, may lead to greater belief in conspiracy theories, perhaps as a defence mechanism [ 24 , 25 ]. Further research on the role of ‘Covid echo chambers’ is needed. Our findings suggest that some people are reluctant to engage with others who hold, or may hold, differing opinions–particularly since COVID-19 policy is such a divisive and emotionally-charged issue. This lack of communication across echo chambers can have an ‘opinion reinforcing’ effect [ 25 ]. In the context of COVID-19 vaccination, strongly ‘pro-vaccination’ advertising or opinions may even be having a counterproductive effect for some, encouraging people to ‘double-down’ on their opposition or adding to their hesitancy. As such, working with individuals and communities, engaging with rather than criticising or dismissing their concerns (both legitimate and illegitimate) via mutually respectful dialogue is essential [ 26 ].
Vaccine delay may be usefully understood in relation to broader conceptual models on ‘patient delay’ [ 27 ]. In the context of COVID-19 vaccines, ‘appraisal delay’ (or decisional delay) [ 28 ] can be thought of as the question of ‘should I get vaccinated’? In order to reduce decisional delay, it is particularly important for policy and health organisations to address informational barriers, including some people’s perceived lack of information, the existence of conspiracy theories, and the existence of ‘Covid echo chambers’. For participants in this study, the lack of information was seen to be partly due to the fact that COVID-19 is a novel disease (i.e. scientists don’t yet have enough information) and due to the fact that insufficient or unclear information was being communicated to them (by e.g. medical or political agencies or individuals). Also, some felt that the information around vaccines and their efficacy or safety was at times too complex to understand, especially in light of new developments. This is perhaps another example of a phenomenon identified throughout the pandemic, referred to as ‘alert fatigue’. This is where frequently changing information (e.g. policies, guidelines, advisories) becomes increasingly difficult to interpret, comprehend and retain for members of the public [ 29 ]. Also, utilization delay (as a form of behavioural delay) can be understood as the time between when a person decides they will need medical intervention (i.e. a vaccine) and the deciding act on that decision (i.e. to get vaccinated). Delayers in this sense had already decided they were going to “need” to be vaccinated. Utilization delay entails the individual asking themselves, is the medical care (i.e. vaccination) worth the costs? In this case, many delayers had decided that the benefits of vaccination–as a ‘passport’ to international travel—were worth the perceived costs (e.g. the perceived infringement of their right to refuse a vaccine or concerns over potential future side effects). The decision to get vaccinated was perceived as not an entirely voluntary one, but one into which they were being ‘nudged’ or even indirectly forced—via the assumption that vaccine passports would be required in the future, especially for international travel.
Limitations
There are a number of limitations to note. Firstly, as with all qualitative studies, the generalizability of the findings is limited. As such, the study cannot be used to make generalisations about how prevalent vaccine acceptance or hesitancy is. Additionally, there was a particularly small number of those in our sample who had refused or were planning to refuse a vaccine and so further research specifically on a larger group of those who have refused the vaccine is needed.
Another limitation of the study is that although attempts were made to recruit and include as diverse a sample as possible, there is a relative underrepresentation of older adults (aged 50+ in the sample). However, for the purposes of the research question around vaccine uptake having a younger sample may be of benefit. Further strengths and limitations of the overall methodology and recruitment in the wider study are discussed in prior publications [ 10 , 11 ]. A further limitation is that the COVID-19 pandemic has (and at time of writing continues to) evolve rapidly, and so subsequent developments (e.g. new variants), may have affected peoples around vaccine uptake. Further research will explore evolving perceptions and any subsequent decisions. However, a particular strength of this study is its ability to provide in-depth and nuanced context as to the reasons behind vaccine acceptance, delay or refusal in the context of COVID-19 among residents in the UK.
This study has provided an in-depth examination of the reasons behind participants’ decisions around getting their initial COVID-19 vaccines. In order to address vaccine uptake and vaccine hesitancy, it is useful to understand the reasons behind people’s decisions to accept or refuse an offer of a vaccine. This qualitative study has suggested that, in the UK, three facilitators—Vaccination as a social norm; Vaccination as a necessity; Trust in science—and six barriers—Preference for “natural immunity”; Concerns over possible side effects; Distrust in government; Perceived lack of information; Conspiracy theories; Covid echo chambers–can help understand why people decide to get a COVID-19 vaccine or not. Future qualitative research looking at different countries is necessary to explore similarities and differences with this study’s findings, given the highly contextual nature of vaccine confidence and vaccine hesitancy.
The finding that convenience was not reported as an issue related to vaccine hesitancy in this study is also important to note. While there appears to have been good access to the vaccines among the general population during the study period, it is important for the key messaging to the community to be well thought out and in line with public belief systems, concerns, and potential misinformation. Findings from this study can help direct such messaging for clinicians/medical providers, community leaders, and public health practitioners. The COVID-19 pandemic, and its policy response, has, and will continue, to rapidly evolve. Further research, particularly longitudinal and comparative research, is needed to explore the evolution of attitudes to vaccines as the pandemic continues.
- View Article
- PubMed/NCBI
- Google Scholar
- 9. UK Government. Vaccinations in the United Coronavirus data: Vaccinations in the United Kingdom. Available at: https://coronavirus.data.gov.uk/details/vaccinations
Effect of methotrexate hold on COVID-19 vaccine response in the patients with autoimmune inflammatory disorders: a systematic review and meta-analysis
- REVIEW ARTICLE
- Published: 27 May 2024
Cite this article
- Muhammad Imran ORCID: orcid.org/0009-0004-2626-9718 1 ,
- Shujaat Ali 1 ,
- Ahmed A. Ibrahim 2 ,
- Areeb Amjad 1 ,
- Aiman Tanveer 1 ,
- Saba Khalil 3 ,
- Mansab Ali 1 &
- Mohamed Abuelazm 4
Immunosuppressants, such as methotrexate (MTX), can suppress the COVID-19 vaccine response in patients with autoimmune diseases. Thus, this study aims to evaluate the effects of MTX hold following COVID-19 vaccination on vaccine efficacy response. A systematic review and meta-analysis of relevant studies retrieved from Web of Science, SCOPUS, PubMed, and CENTRAL from inception until Oct 1, 2023, was conducted. Covidence was used to screen the eligible articles, and all relevant outcomes data were synthesized using risk ratios (RRs) or standardized mean differences (SMDs) with 95% confidence intervals (CIs) in meta-analysis models within RevMan 5.4. PROSPERO ID: CRD42024511628. Four studies with a total of 762 patients with autoimmune inflammatory disorders were included. Holding MTX following the COVID-19 vaccination for approximately 2 weeks was associated with significantly higher antibody titer (SMD: 0.70, 95% CI [0.54, 0.87], P < 0.00001). However, the flare rate was significantly higher in the MTX hold group based on CDAI > 10 or DAS28-CRP > 1.2 either after 1st dose (RR: 2.49 with 95% CI [1.39, 4.47], P = 0.002) or 2nd dose (RR: 2.16 with 95% CI [1.37, 3.41], P = 0.0009) and self-reported disease flare (RR: 1.71 with 95% CI [1.35, 2.17], P < 0.00001). Holding MTX for 2 weeks after the COVID-19 vaccination resulted in significantly higher antibody titer but also had a higher disease flare rate, necessitating cautious clinical monitoring during this period. There is still a need to investigate safer MTX hold duration, considering patients’ vulnerability to COVID-19, disease status, and demographics while adopting this strategy.
This is a preview of subscription content, log in via an institution to check access.
Access this article
Price includes VAT (Russian Federation)
Instant access to the full article PDF.
Rent this article via DeepDyve
Institutional subscriptions
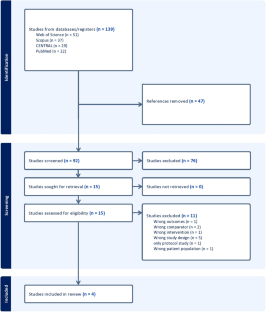
Data availability
The data is available upon reasonable request from the corresponding author.
Panahi Y, Gorabi AM, Talaei S, Beiraghdar F, Akbarzadeh A, Tarhriz V et al (2023) An overview on the treatments and prevention against COVID-19. Virol J 20(1):23. https://doi.org/10.1186/s12985-023-01973-9
Article PubMed PubMed Central Google Scholar
Abuelazm M, Ghanem A, Awad AK, Farahat RA, Labieb F, Katamesh BE et al (2022) The effect of Nitazoxanide on the clinical outcomes in patients with COVID-19: a systematic review and Meta-analysis of randomized controlled trials. Clin Drug Investig 42(12):1031–1047. https://doi.org/10.1007/s40261-022-01213-y
Article CAS PubMed PubMed Central Google Scholar
Tsai J-J, Liu L-T, Chen C-H, Chen L-J, Wang S-I, Wei J-C (2023) COVID-19 outcomes in patients with rheumatoid arthritis with biologic or targeted synthetic DMARDs. RMD Open 9(3):e003038. https://doi.org/10.1136/rmdopen-2023-003038
Bedoui Y, Guillot X, Sélambarom J, Guiraud P, Giry C, Jaffar-Bandjee MC et al (2019) Methotrexate an old drug with new tricks. Int J Mol Sci 20(20):5023. https://doi.org/10.3390/ijms20205023
Chang R, Yen-Ting Chen T, Wang S-I, Hung Y-M, Chen H-Y, Wei C-J (2023) Risk of autoimmune diseases in patients with COVID-19: a retrospective cohort study. EClinicalMedicine 56:101783. https://doi.org/10.1016/j.eclinm.2022.101783
Zhao Z, Hua Z, Luo X, Li Y, Yu L, Li M et al (2022) Application and pharmacological mechanism of methotrexate in rheumatoid arthritis. Biomedicine & Pharmacotherapy = Biomedecine & Pharmacotherapie 150:113074. https://doi.org/10.1016/j.biopha.2022.113074
Article CAS Google Scholar
Ota MOC, Badur S, Romano-Mazzotti L, Friedland LR (2021) Impact of COVID-19 pandemic on routine immunization. Ann Med 53(1):2286–2297. https://doi.org/10.1080/07853890.2021.2009128
Amjad A, Asfar Anwar SA, Waseem F, Khan H, Hidayat M (2022) The challenges for implementation of policies causing a delay in mass immunization against COVID-19: a cross sectional qualitative study. Pak J Med Health Sci 16(11):320
Google Scholar
Mahil SK, Bechman K, Raharja A, Domingo-Vila C, Baudry D, Brown MA et al (2021) The effect of methotrexate and targeted immunosuppression on humoral and cellular immune responses to the COVID-19 vaccine BNT162b2: a cohort study. The Lancet. Rheumatology 3(9):e627–e637. https://doi.org/10.1016/S2665-9913(21)00212-5
Al-Haideri MT, Mannani R, Kaboli R, Gharebakhshi F, Darvishzadehdeldari S, Tahmasebi S et al (2023) The effects of methotrexate on the immune responses to the COVID-19 vaccines in the patients with immune-mediated inflammatory disease: a systematic review of clinical evidence. Transpl Immunol 79:101858. https://doi.org/10.1016/j.trim.2023.101858
Park JK, Lee YJ, Shin K, Ha YJ, Lee EY, Song YW, Choi Y, Winthrop KL, Lee EB (2018) Impact of temporary methotrexate discontinuation for 2 weeks on immunogenicity of seasonal influenza vaccination in patients with rheumatoid arthritis: a randomised clinical trial. Ann Rheum Dis 77(6):898–904. https://doi.org/10.1136/annrheumdis-2018-213222
Article CAS PubMed Google Scholar
Abhishek A, Boyton RJ, Peckham N, McKnight Á, Coates LC, Bluett J et al (2022) Effect of a 2-week interruption in methotrexate treatment versus continued treatment on COVID-19 booster vaccine immunity in adults with inflammatory conditions (VROOM study): a randomised, open label, superiority trial. Lancet Respir Med 10(9):840–850. https://doi.org/10.1016/S2213-2600(22)00186-2
Page MJ, McKenzie JE, Bossuyt PM, Boutron I, Hoffmann TC, Mulrow CD et al (2021) The PRISMA 2020 statement: an updated guideline for reporting systematic reviews. Int J Surg (London, England) 88:105906. https://doi.org/10.1016/j.ijsu.2021.105906
Article Google Scholar
Higgins JP, Savović J, Page MJ, Elbers RG, Sterne JAC (2019) Assessing risk of bias in a randomized trial. In: Cochrane handbook for systematic reviews of interventions, 2nd edn. Wiley-Blackwell, pp 205–228. https://doi.org/10.1002/9781119536604.ch8
Chapter Google Scholar
Sterne JA, Savović J, Page MJ, Elbers RG, Blencowe NS, Boutron I et al (2019) RoB 2: a revised tool for assessing risk of bias in randomised trials. BMJ (Clinical Research Ed) 366:l4898. https://doi.org/10.1136/bmj.l4898
Article PubMed Google Scholar
Araujo CSR, Medeiros-Ribeiro AC, Saad CG, Bonfiglioli KR, Domiciano DS, Shimabuco AY et al (2022) Two-week methotrexate discontinuation in patients with rheumatoid arthritis vaccinated with inactivated SARS-CoV-2 vaccine: a randomised clinical trial. Ann Rheum Dis 81(6):889–897. https://doi.org/10.1136/annrheumdis-2021-221916
de Silva ANA, Frommert LM, Albach FN, Klotsche J, Scholz V, Jeworowski LM et al (2022) Pausing methotrexate improves immunogenicity of COVID-19 vaccination in elderly patients with rheumatic diseases. Ann Rheum Dis 81(6):881–888. https://doi.org/10.1136/annrheumdis-2021-221876
Skaria TG, Sreeprakash A, Umesh R, Joseph S, Mohan M, Ahmed S et al (2022) Withholding methotrexate after vaccination with ChAdOx1 nCov19 in patients with rheumatoid or psoriatic arthritis in India (MIVAC I and II): results of two, parallel, assessor-masked, randomised controlled trials. The Lancet. Rheumatology 4(11):e755–e764. https://doi.org/10.1016/S2665-9913(22)00228-4
Sathish M, Mithun M, Preethi S, Naveen J, Madhan J, Akshay S, Ravikant B (2023) Impact of COVID-19 vaccination in patients with autoimmune diseases-a nationwide survey from 842 autoimmune patients. Indian J Med Sci 75(3):114–120. https://doi.org/10.25259/IJMS_50_2023
Akiyama S, Hamdeh S, Micic D, Sakuraba A (2021) Prevalence and clinical outcomes of COVID-19 in patients with autoimmune diseases: a systematic review and meta-analysis. Ann Rheum Dis 80(3):384–391. https://doi.org/10.1136/annrheumdis-2020-218946
Feuchtenberger M, Kovacs MS, Eder A, Nigg A, Schäfer A (2022) Methotrexate significantly reduces the humoral vaccination response against SARS-CoV-2 in older but not younger patients with rheumatoid arthritis. Rheumatol Int 42(6):959–966. https://doi.org/10.1007/s00296-022-05123-2
Download references
Author information
Authors and affiliations.
University College of Medicine and Dentistry, The University of Lahore, Lahore, Pakistan
Muhammad Imran, Shujaat Ali, Areeb Amjad, Aiman Tanveer & Mansab Ali
Faculty of Medicine, Menoufia University, Menoufia, Egypt
Ahmed A. Ibrahim
Faculty of Medicine, Fatima Jinnah Medical University, Lahore, Pakistan
Saba Khalil
Faculty of Medicine, Tanta University, Tanta, Egypt
Mohamed Abuelazm
You can also search for this author in PubMed Google Scholar
Contributions
M.A. conceived the idea, and M.I. designed the research workflow. M.A. and M.I. searched the databases. S.A., A.A., S.K., A.T., and M.A. screened the retrieved records, extracted relevant data, and assessed the quality of evidence, and M.I. resolved the conflicts. A.A. performed the analysis. M.I., S.A., M.A., and M.A. wrote the final manuscript. M.A. supervised the project. All authors have read and agreed to the final version of the manuscript.
Corresponding author
Correspondence to Muhammad Imran .
Ethics declarations
Ethics approval and consent to participate.
Not applicable.
Consent for publication
Disclosures, additional information, publisher's note.
Springer Nature remains neutral with regard to jurisdictional claims in published maps and institutional affiliations.
Supplementary Information
Below is the link to the electronic supplementary material.
Supplementary file1 (DOCX 2375 KB)
Rights and permissions.
Springer Nature or its licensor (e.g. a society or other partner) holds exclusive rights to this article under a publishing agreement with the author(s) or other rightsholder(s); author self-archiving of the accepted manuscript version of this article is solely governed by the terms of such publishing agreement and applicable law.
Reprints and permissions
About this article
Imran, M., Ali, S., Ibrahim, A.A. et al. Effect of methotrexate hold on COVID-19 vaccine response in the patients with autoimmune inflammatory disorders: a systematic review and meta-analysis. Clin Rheumatol (2024). https://doi.org/10.1007/s10067-024-07013-3
Download citation
Received : 19 April 2024
Revised : 08 May 2024
Accepted : 15 May 2024
Published : 27 May 2024
DOI : https://doi.org/10.1007/s10067-024-07013-3
Share this article
Anyone you share the following link with will be able to read this content:
Sorry, a shareable link is not currently available for this article.
Provided by the Springer Nature SharedIt content-sharing initiative
- Autoimmune diseases
- Disease flare
- Methotrexate hold
- Vaccine response
- Find a journal
- Publish with us
- Track your research
Misinformation Swirled During Taiwan’s 2024 Elections
Study examines misinformation about the U.S. during Taiwan’s presidential election.
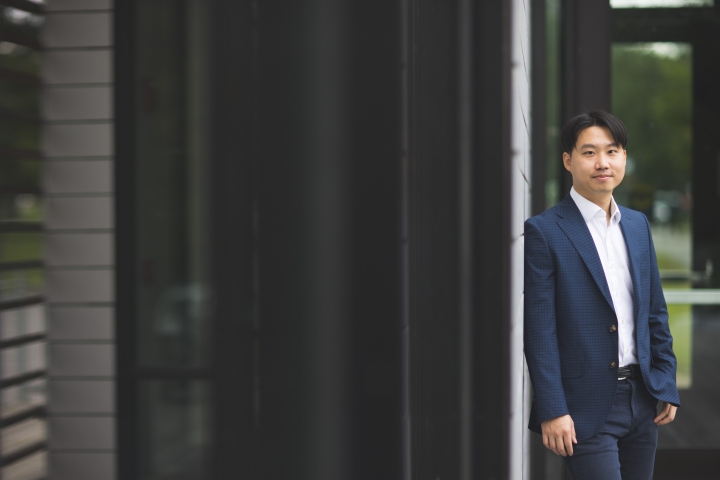
Americans Think AI Will Harm Privacy and Elections
With more than 70 countries hosting national elections, 2024 is the biggest election year in history, according to The Economist.
But how misinformation impacts elections, especially with the rise in content generated by artificial intelligence, continues to be of concern.
A research team examined misinformation narratives on social media in 2023 regarding the Taiwanese presidential election on January 13, 2024. They were especially interested in how narratives targeted relations between Taiwan and the United States.
Misinformation targeted mistrust and skepticism toward the U.S. rather than U.S. foreign policy directly, according to the study, which was published in the Harvard Kennedy School Misinformation Review .
To examine misinformation across three platforms: Line—the most popular messaging app in Taiwan; PTT—the Taiwanese equivalent of Reddit; and Facebook, the researchers applied large language models—AI algorithms like ChatGPT that can understand and generate text and other content.
When Line users come across content that may be dubious, they have the option to forward it to the Cofacts chatbot, a third-party, crowd-sourced platform in which content is fact-checked by volunteers.
Of the 140,300 Line posts relating to the Taiwanese election, which were fact-checked by Cofacts and analyzed by the researchers, nearly 11,000 posts focused on issues relating to Taiwan, the U.S., and China, making it the most prominent fact-checked topic.
The study found that misinformation toward the U.S. contained various narratives, such as the U.S. and the threat of war, especially regarding Ukraine; economic decline due to fiscal actions by the U.S.; and the vaccine supply and the U.S.
For example, some posts suggested that the U.S. deliberately limited the COVID-19 vaccine supply during the pandemic while others stated that U.S. vaccine doses were cheaper domestically than what Taiwan had to pay.
This misinformation may have been planted on social media to damage the United States’ reputation and credibility and “lower the Taiwanese audience’s belief that the U.S. will help,” according to the researchers, as prior research has provided evidence of such actions.
The team found that much of the misinformation on U.S.-Taiwan relations circulated most prominently within China-friendly political groups and referenced U.S.-based conspiracy groups.
“More than half of the misinformation content on the U.S. relating to Taiwan’s recent presidential election in our datasets was multimodal,” says lead author Herbert Chang ’18 , an assistant professor of quantitative social science at Dartmouth. “With so much visual content, our results contribute to the literature and concerns about the impact of AI-generated content on elections.”
By tracking misinformation activity and the location of where the content was posted, the data showed that some users in China and other areas in Asia deliberately masked their geolocations by using virtual private networks to make it look like they were based in the U.S., when in fact that was not the case.
Taiwan’s politics have typically been marked by two parties, the Nationalist Party led by Kuomintang, or KMT, and known as the blue camp, which reflects a more pro-integration sentiment towards China, and the Democratic Progressive Party, known as the green camp, which has wanted to change the country’s name from the Republic of China to Taiwan and modify the constitution. A third party, the Taiwan’s People’s Party, emerged in 2020 with a call for greater attention to domestic and social issues and less emphasis on foreign relations. The analysis builds on research studying social media discourse and support for the presidential candidates, published in PNAS Nexus earlier this year.
Lai Ching-te of the DPP was inaugurated on May 20 as Taiwan’s president, as the DPP won the election for a third consecutive presidency with 40% of the votes. The KMT received 33% of the votes and the TPP received 26% with strong support among 20-somethings.
“This election not only demonstrates the increased importance of international relations in voter choice around the world, at a time of heightened geopolitical conflict, but also how AI can be used to identify and fight misinformation, not just create it,” says Chang.
Austin Wang at the University of Nevada, Las Vegas, and Yu (Sunny) Fang at Barnard College also served as co-authors of the study.
Amy Olson can be reached at [email protected] .
- Society & Culture
- Quantitative Social Sciences
- Department of Mathematics
- Arts and Sciences
- International Relations
- Artificial Intelligence
- Social Media
I stressed earlier in the show that the core of ancient stoic ethics is about the essentially rational nature of the human being, but that doesn’t mean that we always behave in a sensible way—far from it.

IMAGES
VIDEO
COMMENTS
2.2. Research on the Transmission Characteristics of the SARS-CoV-2 Virus. When COVID-19 became a global hot topic, people put forward many speculations that could affect the transmission characteristics of the SARS-CoV-2, such as temperature [16,17,18], humidity [19,20], population density [21,22], age [23,24], and so on.In this regard, scholars have also conducted a lot of research, which ...
In conclusion, no modifying factors seem to modulate the efficacy of vaccines against COVID-19. This quantitative synthesis will need to be updated as soon as further clinical results on the efficacy profile are available from Phase III trials for further licensed COVID-19 vaccines. Keywords: COVID-19; SARS-Cov-2; efficacy; meta-regression ...
No vaccine was statistically significantly associated with a decreased risk for severe COVID-19 than other vaccines, although mRNA-1273 and Gam-COVID-Vac have the highest P-scores (0.899 and 0.816 ...
Participants were enrolled from December 28, 2020 (2 weeks after the introduction of a Covid-19 vaccine), through May 19, 2021, at 33 sites across 25 U.S. states, representing more than 500,000 ...
1. A phase 1/2 randomized, placebo-controlled, multicentre study to evaluate the safety and immunogenicity of COVID-19 vaccine in healthy adults. RBD SARS-CoV-2 HBsAg VLP vaccine, administered at two dose amounts 5 mcg and 25 mcg, by intramuscular injection by investigators during an in-clinic visit. RBD-HBsAg VLPs.
This may help us to understand the effect of contact among individuals and how transmits the virus more quickly. Recently, the serial interval has been calculated for the COVID-19, this estimates by 4.6 days . A reported study mentioned that the mean serial interval for COVID-19 was calculated as 3.96 days.
Background Vaccination has played a pivotal role in reducing the burden of COVID-19. Despite numerous studies highlighting its benefits in reducing the risk of severe disease and death, we still lack a quantitative understanding of how varying vaccination roll-out rates influence COVID-19 mortality. Methods We developed a framework for estimating the number of avertable COVID-19 deaths (ACDs ...
In this study, we perform a large-scale global exploratory study to examine the levels of COVID-19 vaccine acceptance and explore sociodemographic determinants of acceptance. Between October 31 ...
Global COVID-19 vaccine distribution has been inequitable. In this mathematical modelling study, the authors estimate the proportion of deaths that could have been averted in twenty low- and lower ...
COVID-19 vaccines reduce the risk of severe disease in young people, but the absolute risk is low, and side effects have been reported. Here, the authors use data on 5-17 year olds in England to ...
This chapter provides an update on COVID-19 vaccines, emphasizing their immunogenicity, safety, efficacy, and potential impact on vaccine hesitancy, inequity, and future epidemic preparedness. Various vaccine types, such as mRNA-based, DNA-based, viral vector, inactivated, and protein subunit vaccines, are explored, evaluating their mechanisms and advantages in eliciting robust immune responses.
COVID-19 vaccine hesitancy: the five Cs to tackle behavioural and sociodemographic factors. J R Soc Med 2021;114:295-298. Waning vaccine efficacy remains greatest in older individuals and higher-risk groups. Lin DY, Gu Y, Wheeler B, Young H, Holloway S, Sunny SK, et al. Effectiveness of COVID-19 vaccines over a 9-month period in North Carolina.
COVID-19 vaccine uptake varied across countries, in part due to vaccine hesitancy fueled by a lack of trustworthy information. To help health workers provide evidence-based answers to common questions about COVID-19 vaccines and vaccination, and thereby, assist individuals´ decisions on vaccine acceptance, COVID-19 InfoVaccines, a joint WHO-EU project, was launched in February 2021 to support ...
The COVID-19 pandemic has created a new reality where individuals are faced with a previously unknown disease and its effects, providing a unique opportunity to investigate vaccine attitudes during a period of heightened disease salience. The present research reports findings from a longitudinal study conducted during the COVID-19 health crisis ...
At the time COVID-19 vaccines became available, vaccination guidelines in hematology patients under active treatment were conservative, owing to the paucity of vaccine response data and the many uncertainties regarding the immunocompetence of these patients. 5,6 Our study is unique in that it was designed to specifically include severely ...
Access to the COVID-19 vaccine is voluntary and free in the country; people do not need to pay anything.11 To be protected, one needs to receive two doses of the Oxford ... Further research with a longitudinal quantitative design is required to measure knowledge and attitude, and test their relationship with vaccine uptake in order to establish ...
Now, given the urgent need for COVID-19 vaccines, unprecedented financial investments and scientific collaborations are changing how vaccines are developed. This means that some of the steps in the research and development process have been happening in parallel, while still maintaining strict clinical and safety standards.
The spread of the "Severe Acute Respiratory Coronavirus 2" (SARS-CoV-2), the causal agent of COVID-19, was characterized as a pandemic by the World Health Organization (WHO) in March 2020 and has triggered an international public health emergency [].The numbers of confirmed cases and deaths due to COVID-19 are rapidly escalating, counting in millions [], causing massive economic strain ...
By the end of November 2021, scientists estimate that mRNA COVID-19 vaccines had prevented at least 1 million deaths, 10 million hospitalizations, and 36 million SARS-CoV-2 infections in the United States. Sometimes people who are fully vaccinated get a breakthrough infection, meaning that they test positive for SARS-CoV-2 or become ill with ...
A global survey conducted in 19 countries to determine potential acceptance rates and factors influencing acceptance of a covid-19 vaccine found that, on average, 71.5% of respondents would be very or somewhat likely to take a covid-19 vaccine, and 48.1% reported that they would accept their employer's recommendation to do so [26].
The last section enquired about the responder's beliefs and attitudes toward the COVID-19 vaccine based on their previous history with vaccines, religious and social influences, and the usage pattern of Ehteraz through a seven-point Likert scale (Norman, Citation 2010). Surveys were then distributed by a Google Forms link for each language.
Abstract. This qualitative tool provides step by step guidance on how to design and conduct rapid qualitative research with different target groups to understand their barriers and drivers to COVID-19 vaccination. These insights can then be used to inform the development of interventions tailored to the target groups for successful COVID-19 ...
Psychological impacts in COVID - 19 based on survey and its impact. • Data analysis and data visualization algorithms have been applied on collected dataset to simulate the current conditions of psychological health. • Psychological breakdown of human from different ages, genders and professions with the impact of COVID - 19. •
Objective To explore UK public decisions around whether or not to get COVID-19 vaccines, and the facilitators and barriers behind participants' decisions. Design This qualitative study consisted of six online focus groups conducted between 15th March and 22nd April 2021. Data were analysed using a framework approach. Setting Focus groups took place via online videoconferencing (Zoom ...
Immunosuppressants, such as methotrexate (MTX), can suppress the COVID-19 vaccine response in patients with autoimmune diseases. Thus, this study aims to evaluate the effects of MTX hold following COVID-19 vaccination on vaccine efficacy response. A systematic review and meta-analysis of relevant studies retrieved from Web of Science, SCOPUS, PubMed, and CENTRAL from inception until Oct 1 ...
Vaccine Allocation and Distribution: A Review with a Focus on Quantitative Methodologies and Application to Equity, Hesitancy, and COVID-19 Pandemic. Emanuele Blasioli Bahareh Mansouri Srinivas Subramanya Tamvada E. Hassini
The emergence of post-COVID-19 vaccine autoimmune diseases has been co-reported with cases of neurological diseases or new-onset systemic lupus erythematosus which can evoke diverse NAEs [Citation 7]. NAEs are particularly concerning as their incidence continues to be difficult to quantify, and their underlying cause remains uncertain.
Due to the COVID-19 pandemic, many children missed their routine vaccinations globally. There is insufficient evidence on the trends in vaccination coverage in the private healthcare sector in South Africa. This study explored the changes in childhood vaccination patterns (non-COVID vaccines) in the private healthcare sector in South Africa using medicine claim data. Using the information on ...
The analysis builds on research studying social media discourse and support for the presidential candidates, published in PNAS Nexus earlier this year. Lai Ching-te of the DPP was inaugurated on May 20 as Taiwan's president, as the DPP won the election for a third consecutive presidency with 40% of the votes.
Higher rates of COVID-19 infections and lower rates of COVID-19 screening and vaccine uptake among Black Canadians are found due to pre-COVID-19 experiences of institutional and structural racism, health inequities and a mistrust of health care professionals that further impeded access to health care. Abstract Introduction: The COVID-19 pandemic exacerbated health inequities worldwide ...