- Research article
- Open access
- Published: 17 July 2020

The quality of drinking and domestic water from the surface water sources (lakes, rivers, irrigation canals and ponds) and springs in cholera prone communities of Uganda: an analysis of vital physicochemical parameters
- Godfrey Bwire ORCID: orcid.org/0000-0002-8376-2857 1 ,
- David A. Sack 2 ,
- Atek Kagirita 3 ,
- Tonny Obala 4 ,
- Amanda K. Debes 2 ,
- Malathi Ram 2 ,
- Henry Komakech 1 ,
- Christine Marie George 2 &
- Christopher Garimoi Orach 1
BMC Public Health volume 20 , Article number: 1128 ( 2020 ) Cite this article
16k Accesses
36 Citations
1 Altmetric
Metrics details
Water is the most abundant resource on earth, however water scarcity affects more than 40% of people worldwide. Access to safe drinking water is a basic human right and is a United Nations Sustainable Development Goal (SDG) 6. Globally, waterborne diseases such as cholera are responsible for over two million deaths annually. Cholera is a major cause of ill-health in Africa and Uganda. This study aimed to determine the physicochemical characteristics of the surface and spring water in cholera endemic communities of Uganda in order to promote access to safe drinking water.
A longitudinal study was carried out between February 2015 and January 2016 in cholera prone communities of Uganda. Surface and spring water used for domestic purposes including drinking from 27 sites (lakes, rivers, irrigation canal, springs and ponds) were tested monthly to determine the vital physicochemical parameters, namely pH, temperature, dissolved oxygen, conductivity and turbidity.
Overall, 318 water samples were tested. Twenty-six percent (36/135) of the tested samples had mean test results that were outside the World Health Organization (WHO) recommended drinking water range. All sites (100%, 27/27) had mean water turbidity values greater than the WHO drinking water recommended standards and the temperature of above 17 °C. In addition, 27% (3/11) of the lake sites and 2/5 of the ponds had pH and dissolved oxygen respectively outside the WHO recommended range of 6.5–8.5 for pH and less than 5 mg/L for dissolved oxygen. These physicochemical conditions were ideal for survival of Vibrio. cholerae .
Conclusions
This study showed that surface water and springs in the study area were unsafe for drinking and had favourable physicochemical parameters for propagation of waterborne diseases including cholera. Therefore, for Uganda to attain the SDG 6 targets and to eliminate cholera by 2030, more efforts are needed to promote access to safe drinking water. Also, since this study only established the vital water physicochemical parameters, further studies are recommended to determine the other water physicochemical parameters such as the nitrates and copper. Studies are also needed to establish the causal-effect relationship between V. cholerae and the physicochemical parameters.
Peer Review reports
Water is the most abundant resource on the planet earth [ 1 ], however its scarcity affects more than 40% of the people around the world [ 2 ]. Natural water is an important material for the life of both animals and plants on the earth [ 3 ]. Consequently, access to safe drinking water is essential for health and a basic human right that is integral to the United Nations Resolution 64/292 of 2010 [ 4 ]. The United Nations set 2030 as the timeline for all countries and people to have universal access to safe drinking water; this is a Sustainable Development Goal (SDG) 6 of the 17 SDGs [ 5 ]. The availability of and access to safe water is more important to existence in Africa than it is elsewhere in the world [ 6 ]. Least Developed Countries (LDCs) especially in sub-Saharan Africa have the lowest access to safe drinking water [ 7 ]. In Africa, rural residents have far less access to safe drinking water and sanitation than their urban counterparts [ 8 ].
Natural water exists in three forms namely; ground water, rain water and surface water. Of the three forms, surface water is the most accessible. Worldwide, 144 million people depend on surface water for their survival [ 9 ]. In Uganda, 7% of the population depends on surface water (lakes, rivers, irrigation canal, ponds) for drinking water [ 10 ]. The same surface water is a natural habitat for many living organisms [ 11 ] some of which are responsible for transmission of infectious diseases such as cholera, typhoid, dysentery, guinea worm among others [ 12 ]. Surface water sources include lakes, rivers, streams, canals, and ponds. These surface water sources are often vulnerable to contamination by human, animal activities and weather (storms or heavy rain) [ 13 , 14 ]. Globally, waterborne diseases such as diarrheal are responsible for more than two million deaths annually. The majority of these deaths occur among children under-5 years of age [ 15 ].
Cholera, a waterborne disease causes many deaths each year in Africa, Asia and Latin America [ 16 ]. In 2018 alone, a total of 120,652 cholera cases and 2436 deaths were reported from 17 African countries to World Health Organization [ 17 ]. Cholera is a major cause of morbidity and mortality in Uganda [ 18 ]. The fishing communities located along the major lakes and the rivers in the African Great Lakes basin of Uganda constitutes 5% of the Uganda’s population, however these communities were responsible for the majority (58%) of the reported cholera cases during the period 2011–2015 [ 19 ]. Cholera outbreaks affect predominantly communities using the surface water and the springs. There is also high risk of waterborne disease outbreaks in the communities using these types of water [ 20 , 21 ]. Studies of the surface water from water sources located in the lake basins of the five African Great Lakes in Uganda identified Vibrio. cholerae [ 22 , 23 ] though no study isolated the toxigenic V. cholerae O1 or O139 that cause epidemic cholera. Cholera outbreaks in the African Great Lakes basins in Uganda have been shown to be propagated through water contaminated with sewage [ 20 , 24 ]. Cholera is one of the diseases targeted for elimination globally by the WHO by 2030 [ 25 ]. Hence, to prevent and control cholera outbreaks in these communities, promotion of use of safe water (both quantity and quality), improved sanitation and hygiene are the interventions prioritized by the Uganda Ministry of Health [ 26 ]. Most importantly, provision of adequate safe water is a major pillar of an effective cholera prevention program given that water is the main mode of V. cholerae transmission [ 27 , 28 ].
Availability of adequate safe water is essential for prevention of enteric diseases including cholera [ 29 ]. Therefore, access to safe drinking and domestic water in terms of quantity and quality is key to cholera prevention. Water quality is defined in terms of three key quality parameters namely, physical, chemical and microbiological characteristics [ 30 ]. A less common but important parameter is the radiological characteristics [ 31 ]. In regards to the physicochemical parameters, there are five parameters that are essential and impacts life (both flora and or fauna) within the aquatic systems [ 32 ]. These vital physicochemical parameters include pH, temperature, dissolved oxygen, conductivity and turbidity [ 32 ].
pH is a value that is based on logarithm scale of 0–14 [ 33 ]. Aquatic organisms prefer pH range of 6.5–8.5 [ 34 , 35 , 36 ]. Low pH can cause the release of toxic elements or compounds into the water [ 37 ]. The optimal pH for V. cholerae survival is in basic range (above 7). Vibrio cholerae may not survive for long in acidic pH [ 38 ]. A solution of pH below 4.5 will kill V.cholerae bacteria [ 39 ].
Most aquatic organisms are adapted to live in a narrow temperature range and they die when the temperature is too low or too high [ 34 ]. Vibrio cholerae , bacteria proliferate during algae bloom resulting in cholera outbreaks [ 40 , 41 ]. This proliferation could be due favourable warm temperature [ 42 ]. Relatedly, V. cholerae isolation from natural water in endemic settings is strongly correlated with water temperature above 17 °C [ 43 ].
Dissolved oxygen is the oxygen present in water that is available to aquatic organisms [ 34 ]. Dissolved oxygen is measured in parts per million (ppm) or milligrams per litre (mg/L) [ 35 ]. Organisms in water need oxygen in order to survive [ 44 ]. Decomposition of organic materials and sewage are major causes of low dissolved oxygen in water [ 12 ].
Water conductivity is the ability of water to pass an electrical current and is expressed as millisiemens per metre (1 mS m- 1 = 10 μS cm − 1 ) [ 29 ]. Most aquatic organisms can only tolerate a specific conductivity range [ 45 ]. Water conductivity increases with raising temperature [ 46 ]. There is no set standard for water conductivity [ 45 ]. Freshwater sources have conductivity of 100 – 2000μS cm − 1 . High water conductivity may be due to inorganic dissolved solids [ 46 ].
Turbidity is an optical determination of water clarity [ 47 ]. Turbidity can come from suspended sediment such as silt or clay [ 48 ]. High levels of total suspended solids will increase water temperatures and decrease dissolved oxygen (DO) levels [ 12 ]. In addition, some pathogens like V. cholerae, Giardia lambdia and Cryptosporidia exploit the high water turbidity to hide from the effect of water treatment agents and cause waterborne diseases [ 49 ]. Consequently, high water turbidity can promotes the development of harmful algal blooms [ 41 , 50 ].
Given the importance of the water physicochemical parameters, in order to ensure that they are within the acceptable limits, the WHO recommends that they are monitored regularly [ 51 ]. The recommended physicochemical parameters range for raw water are for pH of 6.5–8.5, turbidity of less than 5Nephlometric Units (NTU) and dissolved oxygen of not less than 5 mg/L [ 51 ]. Surface and spring water with turbidity that exceeds 5NTU should be treated to remove suspended matter before disinfection by either sedimentation (coagulation and flocculation) and or filtration [ 52 ].
Water chlorination using chlorine tablets or other chlorine releasing reagent is one of the most common methods employed to disinfect drinking water [ 53 , 54 ]. Chlorination is an important component of cholera prevention and control program [ 55 ]. In addition to disinfection to kill the pathogens, drinking water should also be safe in terms of physicochemical parameters as recommended by WHO [ 51 ]. However, to effectively make the water safe using chlorine tablets and other reagents, knowledge of the physicochemical properties of the surface and spring water being disinfected is important as several of the parameters affect the active component in the chlorine tablets [ 56 ]. For example, chlorine is not effective for water with pH above 8.5 or turbidity of above 5NTU [ 53 ].
Generally, there is scarcity of information about the quality and safety of drinking water in Africa [ 57 ]. Similarly, few studies exist on the physicochemical characteristics of the drinking water and water in general in Uganda. Furthermore, information from such studies is inadequate for use to increase safe water in cholera prone districts of Uganda where the need is greatest. The cholera endemic communities of Uganda [ 19 , 21 , 24 ] have adequate quantities of water that is often collected from the Great lakes, rivers and other surface water sources located within the lake basins. However, the water is of poor quality in terms of physicochemical and microbiological characteristics. Several studies conducted in Uganda have documented microbiological contamination of drinking water [ 20 , 24 , 58 , 59 ]. However, few studies exist on the physicochemical characteristics of these water. Furthermore, these studies focused on few water sources, for example testing the lakes and omitted the rivers, springs and ponds or testing the rivers and omitted the other water types. One such study was carried out on the water from the three lakes in western Rift valley and Lake Victoria in Uganda [ 23 ], This study did not assess the other common water sources such as the rivers, ponds and springs that were used by the communities for drinking and other household purposes. Other studies on water physicochemical characteristics assessed heavy metal water pollution of River Rwizi (Mbarara district, Western Uganda) [ 60 ] and of the drinking water (bottled, ground and tap water) in Kampala City (Central Uganda) [ 61 ] and Bushenyi district (Western Uganda) [ 62 ]. These studies found high heavy metal water pollution in the drinking water tested. The information gathered from such studies is useful in specific study area and is inadequate to address the lack of safe water in the cholera endemic districts of Uganda where the need for safe drinking water is greatest. Several epidemiological studies in Uganda have attributed cholera outbreaks to use of contaminated surface water [ 20 , 21 , 24 , 63 ]. Furthermore, studies conducted on the surface water focus on pathogen identification [ 63 , 64 ] leaving out the water physicochemical parameters which are equally important in the provision of safe drinking water [ 53 ] and are necessary for survival of all living organisms (both animals and plants) [ 44 ].
Therefore, the aim of this study was to determine the physicochemical characteristics of the surface water sources and springs located in African Great Lakes basins in Uganda so as to guide the interventions for provision of safe water to cholera prone populations [ 19 , 20 , 21 , 24 , 58 ] of Uganda. This study in addition has the potential to guide Uganda to attain the United Nations SDG 6 target of universal access to safe drinking water [ 2 ] and the WHO cholera elimination Roadmap [ 25 ] by 2030. Furthermore, these findings may guide future studies including those on causal-effect relationship between physicochemical parameters and infectious agents (pathogens).
This was a longitudinal study that was conducted between February 2015 and January 2016 in six districts of Uganda that are located in the African Great Lakes basins of the five lakes (Victoria, Albert, Kyoga, Edward and George). These districts had ongoing cholera outbreaks or history of cholera outbreaks in the previous five to 10 years (2005–2015). In addition, the selected study districts had border access to the following major water bodies (lakes: Victoria, Albert, Edward, George and Kyoga). The study area was purposively selected because the communities residing along these major lakes contributed most (58%) of the reported cholera cases and deaths in Uganda [ 19 , 65 ] and in the sub-Saharan Africa region [ 66 ] in the past 10 years. Water samples were collected monthly from 27 sites used by the communities for household purposes that included drinking. Water samples were then tested to determine the vital physicochemical parameters. The water samples were collected from lakes, rivers, springs, ponds and an irrigation canal that were located in the lake basins of the five African Great Lakes in Uganda. In one site, water was also collected from a nearby drainage channel and tested for V. cholerae [ 22 ] and physicochemical parameters. However, because the channel was not used for drinking the results were omitted in this article. Water samples were analysed to determine the pH, temperature, dissolve oxygen, conductivity and turbidity. The study sites were located in the districts of Kampala and Kayunga in central region of Uganda; Kasese and Buliisa districts in western Uganda; Nebbi and Busia districts in northern and eastern Uganda respectively. The study sites were the same as for the simultaneous bacteriological V. cholerae detection study [ 22 ] and are shown in Fig. 1 .
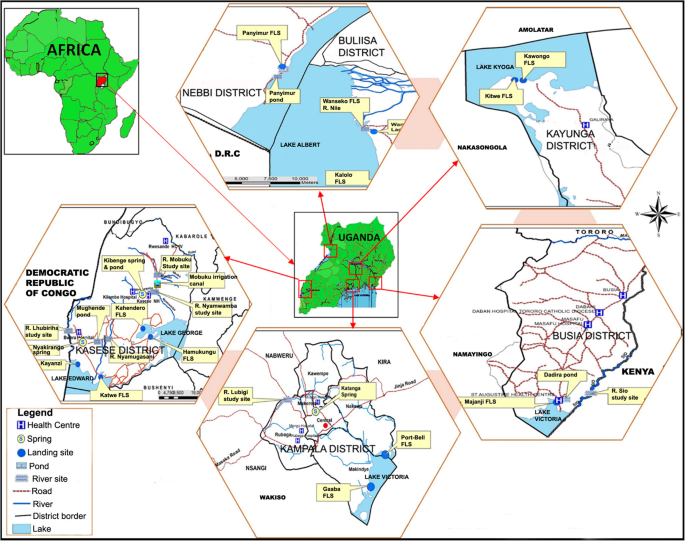
Map showing the location of Uganda, the study districts, major surface water sources and the study sites, February 2015 – January 2016. The blue shades are the African Great Lakes and their basins. (Map generated by ArcGIS version 10.2 [licenced] and assembled using Microsoft Office PowerPoint, Version 2016 [licenced] by the authors)
Rural-urban categorization of the study sites
The study sites were categorized as urban if they were found in Kampala district (the Capital City of Uganda) or rural if they were in the other five remote study districts (Kasese, Kayunga, Busia, Nebbi and Buliisa).
Identification of the study sites and water testing procedures
The sites for water testing were identified with the guidance of the local communities and after direct observation by the study team. Geo-coordinates of the sites were taken at the beginning of the study to ensure that subsequent water collection and measurements were done on water from specific points. Two water collection sites were selected on each of the African Great Lakes in Uganda. The selected sites were in different locations but within the communities with a history of cholera outbreaks in the previous 10 years prior to the study period. For each selected lake point, a site was also selected on a river, a spring and a pond located within the area and being used by the communities for domestic purposes that included drinking and preparation of food. A total of 27 sites, two of which were from each of the five lakes were selected and the water tested. The number of sites on each lake and their locations are shown in Additional file 1 .
Water samples were collected and tested monthly for 12 months by the research assistants who were health workers with background training in microbiology or environmental health. The research assistants received training on water collection and testing from a water engineer. The physicochemical parameters were measured by use of the digital meters namely the Hach meter HQ40d and digital turbidity meter.
Water samples were collected in five-litre containers, three litres were processed for V. cholerae detection by Polymerase Chain Reaction (PCR) test as previously described [ 67 ]. Vibrio cholerae Non O1/Non O139 pathogens were frequently detected in the water samples during the study period [ 22 ]. While the three litres of water were being processed for V. cholerae detection [ 22 ], the rest of the water (2 l), were simultaneously used for the onsite measurement of temperature, pH, conductivity and dissolved oxygen. The Hach meters , HQ40d used in the study, had three electrodes that were calibrated before each monthly testing according to the manufacturers’ manual [ 68 ]. The Hach meter calibrations were done using three specific standard buffer solutions that were for pH, dissolved oxygen and conductivity respectively. Turbidity (total suspended solids or water clarity) was measured using a turbidity meter according to previously published methods [ 49 ]. In addition, the research assistants were provided with Standard Operating Procedures (SOPs) and supervised monthly by the investigators before and during each scheduled monthly measurements.
Data management, analysis and statistical tests
Data were collected, entered, cleaned and stored in the spreadsheet. Errors in the recorded readings were removed using the correct records retrieved from the Hach meters’ HQ40d internal memory. Stata statistical package version 13 was used to analyse the data. Data were analysed to generate means and standard error of the mean for pH, temperature, dissolved oxygen (DO), conductivity (CD) and turbidity. Data were presented in the form of tables and graphs. Comparison for variations between the water samples were carried out using One-Way Analysis of Variance (ANOVA) test. Samples with significant One-Way ANOVA test were subjected to Turkey’s Post Hoc test to establish which of the variables were statistically significant.
The map was created using ArcGIS software, Version 10.2, licenced (ESRI, Redlands, California, USA). The graphs and figures were produced using Microsoft Excel and PowerPoints, Version 2016 (Microsoft, Redmond, Washington, USA). The administrative shapefiles used to create the map were obtained from open access domain, the Humanitarian Data Exchange: https://data.humdata.org/ . In order to generate the study locations on the map, Global Positioning System (GPS) coordinates for the study sites were converted to shapefiles that were combined with the administrative shapefiles corresponding to the locations.
A total of 318 water samples were tested from 27 sites as follows; lake water 40.9%, (130/318), rivers water 26.4% (84/318), ponds water 17.9% (57/318), spring water 11.0% (35/318) and canal water 3.8% (12/318).
Test results for the lake water collected at the fish landing sites (FLS)
The mean physicochemical test results for pH, temperature, dissolved oxygen, conductivity and turbidity are shown in Table 1 .
The mean physicochemical water characteristics of most of the sites were within the WHO recommended water safety range except for turbidity. Few sites had pH and dissolved oxygen outside the WHO recommended safety range.
Monthly variations of the lake water physicochemical characteristics
There were monthly variations in the physicochemical parameters between the water from the lake sites overtime. Most of the sites had steady pH overtime for the first half of the study period (February – August 2015). Thereafter, the pH reduced slightly during the second half (September, 2015 – January, 2016) of the study period. The highest pH fluctuations were in the months of October – December, 2015. The widest change in pH within the same site was observed at Gaaba Fish landing site, Lake Victoria basin, Kampala district.
There were differences in water temperature on the same lake but at different test sites. These differences were detectable mostly in the months of April, 2015. The lowest and highest water temperatures were both recorded on Lake Edward (Kasese district) at Kayanzi fish landing site of 18.9 °C and at Katwe FLS of 34 ° C in the period between April – August, 2015. Fluctuations in the dissolved oxygen were detectable throughout the study period. Kalolo Fish landing site on Lake Albert, Buliisa district showed the widest fluctuations in dissolved oxygen with the highest value of 10.73 mg/L and the lowest of 2.5 mg/L.
Most test sites had small conductivity fluctuations except for Panyimur and Kalolo both of which were located on Lake Albert in Nebbi and Buliisa districts These districts had high water conductivity fluctuations with arrange of 267.1 μS/cm – 2640 μS/cm at Kalolo (Buliisa district) FLS and 296 μS/cm – 2061 μS/cm at Panyimur (Nebbi district). Water turbidity for the majority of the sites changed overtime. Kahendero fish landing site (Lake George, Kasese district) had the highest turbidity which was most noticeable in the months of October 2015 to January 2016. Majanji fish landing site (Lake Victoria, Busia district) had the lowest and most stable water turbidity. Monthly variations of the lake water physicochemical parameters are shown in Fig. 2 .
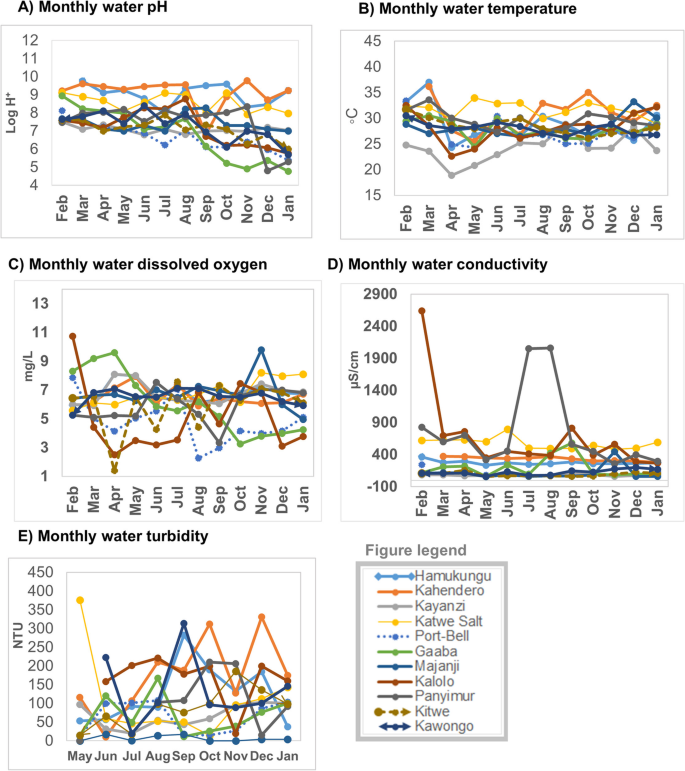
Monthly variations of lake water physicochemical characteristics (pH, temperature, dissolved oxygen, conductivity and turbidity), February 2015 – January 2016: Part a ) water pH variations; Part b ) water temperature variations; Part c ) water dissolved oxygen; Part d ) water conductivity variations; Part e ) water turbidity variations
River water physicochemical parameter test results
The mean physicochemical characteristics of water from the seven rivers studied are shown in Table 2 .
There were variations in the mean pH, temperature, dissolved oxygen and conductivity between study sites on the rivers. However, these mean parameter variations were in WHO acceptable drinking water safety limit except for River Lubigi, Kampala district which had mean dissolved oxygen below the recommended WHO range. At one time (February, 2015) River Lubigi had dissolved oxygen of 0.45 mg/L. The river water turbidity for all the test sites were above that recommended by WHO of less than 5NTU.
Monthly variations of the river water physicochemical characteristics
Monthly variations in the water physicochemical characteristics of the seven river test sites are shown in Fig. 3 .
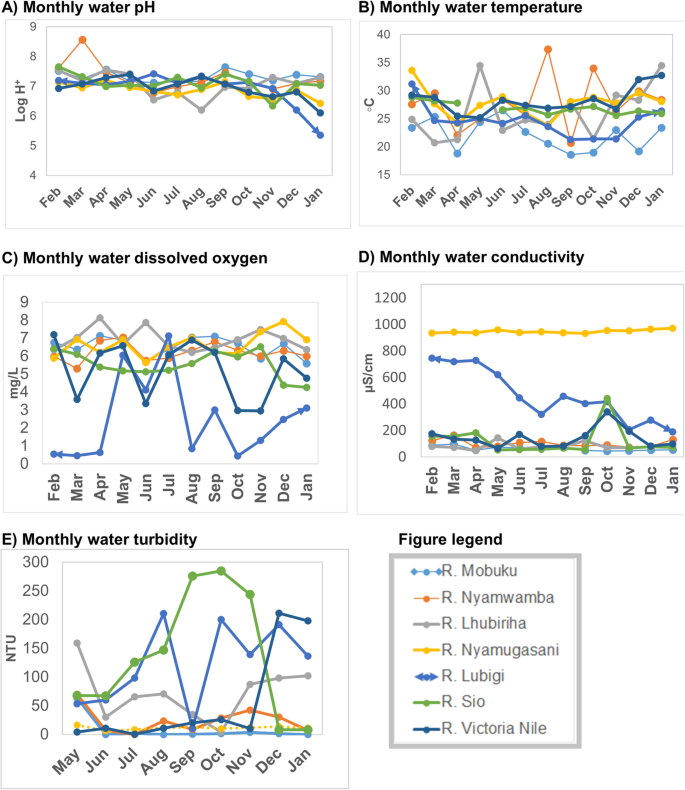
Monthly variations of the physicochemical characteristics of river water, February 2015 – January 2016: Part a ) water pH variations; Part b ) water temperature variations; Part c ) water dissolved oxygen variations; Part d ) water conductivity variations; Part e ) water turbidity variations
There were variations in the water physicochemical parameters between rivers and within the same river overtime. Most rivers showed fluctuations of water pH and temperature. Some rivers such as R. Nyamugasani and R. Lhubiriha both in Kasese district had wide temperature fluctuations. River Mobuku (Kasese district) had the lowest water temperature recorded over the study period. Fluctuations in dissolved oxygen were highest in R. Lubigi (Kampala district), Lake Victoria basin. Dissolved oxygen for R. Lubigi was below the recommended level of more than 5 mg/L for most of the study period. Seasonal variations of water dissolved oxygen were also more noticeable in R. Lubigi than the rest of the river sites. Relatively more dissolved oxygen was found during the rainy seasons (March – July, 2015, first rainy season and September – December, 2015, second rainy season) than in dry season.
There were small variations in the water conductivity in the majority of the rivers. Wide fluctuations in conductivity were observed for water samples of R, Lubigi (Kampala district). River Nyamugasani (Kasese district, Lake Edward basin) had steady but higher conductivity than all the other rivers. There were variations in turbidity within the same river overtime and between the different rivers. River Sio (Busia district) had the highest and the widest turbidity variations during the study period.
Water test results for the springs and ponds
The mean physicochemical characteristics of spring and pond water are shown in Table 3 .
The mean physicochemical characteristics of water from the springs and ponds showed variations between the sites. The majority of site means values were within the WHO accepted pH range. Two sites, Wanseko pond (Buliisa, district, Lake Albert basin) and Katanga spring (Kampala district, Lake Victoria basin) had mean water pH below the recommended WHO drinking water acceptable range at the acidic level of 5.73 and 6.19 respectively. Forty percent (40%, 2/5) of the ponds and 33% (1/3) of the springs had mean dissolved oxygen below the recommended WHO level. The ponds with the low dissolved oxygen were found within Lake Albert basin. Among the springs, Katanga spring (Kampala district, L. victoria basin) had mean dissolved oxygen that was below the WHO recommended level of 5 mg/L. Conductivities of the spring water were 89.81–3276.36 μS/cm and for ponds 55.99–3280.83 μS/cm. For both the springs and the ponds the differences between the lowest and the highest conductivities were wide.
Monthly variations of the springs and ponds water physicochemical characteristics
The monthly variations of spring and pond water physicochemical characteristics are shown in Fig. 4 .
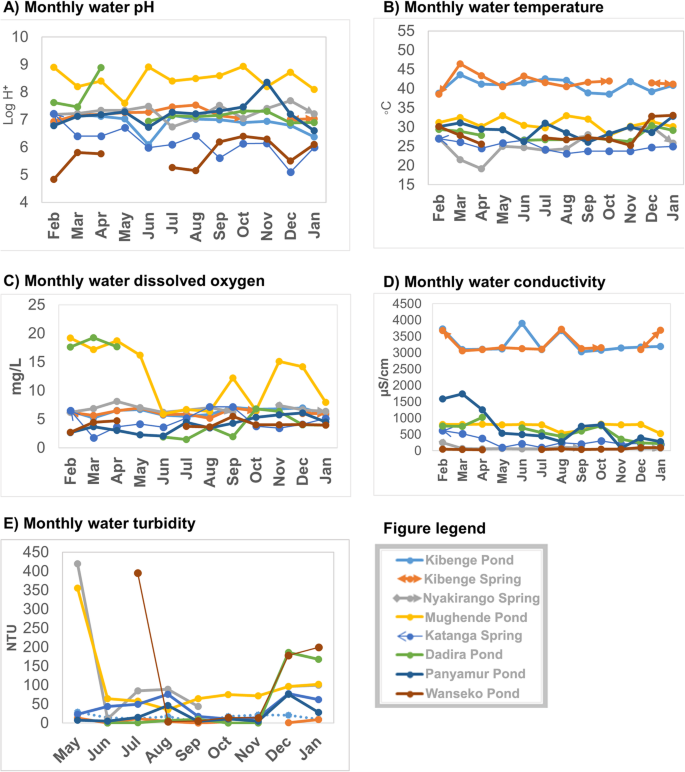
Monthly variations of the physicochemical characteristics of the spring and pond water, February 2015 – January 2016: Part a ) water pH variations; Part b ) water temperature variations; Part c ) dissolved oxygen variations; Part d ) conductivity variations and Part e ) water turbidity variations
There were variations in the water physicochemical characteristics of the spring and the pond water overtime. The variations in water (springs and ponds) were also present between the different sites. The springs had small monthly variations of the water physicochemical parameters while the ponds had wide variations. Mughende pond (Kasese district) had the highest pH for most of the study period. Katanga spring (Kampala district) had the lowest pH compared to other springs during the study period. Kibenge spring (Kasese district) had higher temperature than the rest of the two springs (Katanga spring, Kampala district and Nyakirango spring, Kasese district). Most springs and ponds had slight fluctuations in dissolved oxygen except for Mughende pond (Kasese district). Most springs and ponds except for Panyimur pond (Nebbi district) had small monthly fluctuations in water conductivity. Kibenge spring and pond (both located in Kasese district) had higher conductivity compared to the rest of the springs or ponds. Mughende spring and pond were outliers with higher conductivity than the rest of the water sites. There were variations in water turbidity with months for both the springs and the ponds. Apart from Mughende pond (Kasese district), the rest of the springs and ponds showed variations that had two peaks, the first peak (May – August, 2015) and the second peak (November – January, 2016).
Test results of the other surface water sources: Mobuku irrigation canal water
Mobuku irrigation canal water, water diverted from Mobuku River for irrigation purposes by the Mobuku irrigation scheme was tested because the local communities were using this water for domestic purposes including drinking. Apart from water turbidity which was above the WHO recommended standard of 5NTU, the rest of the water physicochemical parameters (pH, temperature, dissolved oxygen and conductivity) were in the WHO acceptable range as follow: pH of 7.93 ± Standard Error (SE) of 0.23, temperature of 26.57 °C ± SE of 1.25 °C, dissolved oxygen of 6.38 mg/L ± SE of 0.18 mg/L, conductivity of 69.06 ± SE of 2.57) and turbidity of 28.68 ± SE of 9.06NTU.
Monthly variations of physicochemical characteristics of Mobuku irrigation canal water
There were monthly variations in water physicochemical characteristics of Mobuku irrigation canal. The water pH and dissolved oxygen showed two peaks each. The first peak was in March – May, 2015 and the second peak, August – November, 2015. The variations of the Mobuku irrigation canal monthly water physicochemical parameters over the study period is shown in Fig. 5 .
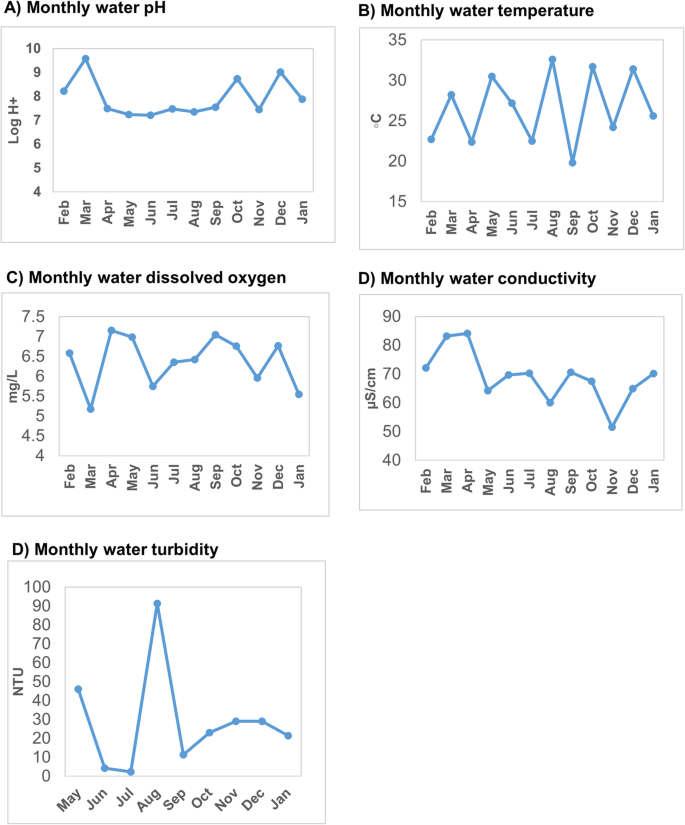
Monthly variations of the physicochemical characteristics of Mobuku irrigation canal water, February 2015 – January 2016: Part a ) water pH variations; Part b ) water temperature variations; Part c ) dissolved oxygen variations; Part d ) conductivity variations and Part e ) water turbidity variations
Results of statistical tests for the differences within sites overtime and between sites
One-Way ANOVA test.
There were no statistically significant differences within most of the study sites except for sites on the lakes and the rivers where the pH and temperature differences were statistically significantly within sites overtime. Statistically significant differences in the water physicochemical characteristics were observed between sites (all p -value < 0.05) as indicated in the additional file 2 .
Turkey’s post hoc test
There were statistically significant differences for all water physicochemical parameters for both the lake and river sites. For instance, Lake Edward had both the highest temperature (34 °C, May, 2015) which was registered at Katwe FLS (Kasese district) and the lowest temperature (18.9 °C, April, 2015) which was recorded at Kayanzi FLS (Kasese district). The results of the comparison of the physicochemical parameters of the various lake and river sites are shown in Table 4 .
Similarly, comparison of the springs or pond water showed statistically significant differences for most (80% of the total comparison) of the water parameters (pH, temperature, dissolved oxygen and conductivity) apart from the water turbidity. Turkey’s post Hoc test results for the comparison of springs and pond water physicochemical parameters are shown in Table 5 .
This study showed that water for drinking and domestic purposes from the surface water sources and springs in cholera affected communities/districts of Uganda were not safe for human use in natural form. The water samples from the water sources in the study area did not meet the WHO drinking water quality standards in terms of the important physicochemical parameters. In addition, all the surface water sources and the springs tested had turbidity above the WHO recommended level of 5NTU yet the same water were used for domestic purposes including drinking in the natural form by the households. The study also found variations in the other physicochemical parameters (pH, temperature, dissolved oxygen and conductivity) between study sites on the same lake and between the different water sources.
While the majority of the water sources had mean water physicochemical characteristics (excluding turbidity) in acceptable range, few water sources, mainly the sites on Lake George, including the springs and ponds had pH and dissolved oxygen outside the recommended WHO ranges. These water sources that did not meet the WHO drinking water standards could expose the users to harmful effects of unsafe drinking water including waterborne diseases such as cholera. The present study findings of high water turbidity if due to algae bloom could encourage pathogen persistence and infection spread, including V. cholerae bacteria [ 40 , 41 ] resulting in ill-health and cholera epidemics. In addition, the high water turbidity complicates water disinfection as it gives rise to significant chlorine demand [ 53 ]. The increased chlorine demand can be costly and difficult to ensure constant availability for disinfection of water since Uganda and several other developing countries need and receive supplementary donor support [ 69 ].
In regard to temperature, dissolved oxygen and conductivity, the majority of the surface water sources and springs tested met the recommended WHO drinking water standards. However, a few water sources such as River Lubigi in Kampala district had mean dissolved oxygen below the recommended WHO drinking water standards. Therefore, in order to ensure universal access to safe drinking water, the water sources that had vital physicochemical parameters outside the WHO drinking water range could be targeted for further studies.
There were statistically significant differences in the water physicochemical characteristics between the different sites and sources (lakes, rivers, springs and ponds). Despite these differences, the required approaches to ensure safe water access to the communities may not differ across sites. First and foremost, all sites and water types will need measures that reduce the high water turbidity to WHO acceptable levels. Secondly, in few instances, such as the water sources with pH in acidic range (Katanga spring in Kampala district, Lake Victoria Basin and Wanseko pond in Buliisa district, lake Albert basin) in addition to requiring further studies to identify the causes of the low pH (acidity), such water sources may also require the use of water treatment methods that neutralize the excess acidity [ 54 ]. Furthermore, since acidity is usually associated with increased solubility of toxic heavy metals (lead, arsenic and others) [ 34 ], testing such water for metallic contamination may be required. Heavy metal contamination of water causes ill-health due to chronic exposure which is cumulative and manifest late for correction to be done [ 70 ].
The findings of this study also highlight the differences in water quality between the urban surface water sources and springs (Kampala district) and the rural surface sources and springs (other study districts – Kasese, Kayunga, Busia, Nebbi and Buliisa) The water sources that met the WHO recommended drinking water quality standards [ 53 ] were mostly the rural springs and the rivers. However, these differences between the rural and the urban water sources do not alter the required approaches to ensure access to safe water which is by promoting measures that reduce the high water turbidity in combination with water disinfection to remove the pathogens. The relatively good quality of rural water sources compared to the urban ones could have been due to availability of plenty of vegetation in rural setting that filtered the water along the way downstream and possibly low level of pollution from industrial inputs in rural areas than in urban areas [ 71 , 72 ].
In relation to cholera outbreaks in the study communities, naturally, the physicochemical conditions for survival of V. cholerae O1 occur in an estuarine environment and other brackish waters [ 73 , 74 ]. In such circumstances, the favourable physicochemical conditions for V. cholerae isolation are the high water turbidity [ 49 ] and temperature of above 17 °C [ 43 ]. Interestingly, all the surface water sources and the springs tested had favourable physicochemical characteristics for the survival of V. cholerae in terms of these two parameters (high water turbidity and temperature of above 17 °C). Furthermore, two lakes sites (Kahendero FLS and Hamukungu FLS, Lake George, Kasese district) had also favourable mean pH for the survival of V. cholerae of 9.03 ± 0.17 and 9.13 ± 0.23 respectively. Favourable pH for V. cholerae survival in waters of Lake George was previously documented in the same area [ 23 ]. Hence, the frequent cholera outbreaks [ 19 , 20 , 21 , 24 ] in the study area could be attributed to both the favourable physicochemical water characteristics and use of unsafe water.
There were wide variations in conductivity between water sources and within the same source overtime. High water conductivities were recorded in the months of January to March 2015 (dry season), possibly due to high evaporation which increased the concentration of electrolytes present in water. Likewise, two rivers namely. River Lubigi (Kampala district) and Nyamugasani (Kasese district) had higher mean conductivities of 460.51 ± 57.83 μS/cm and 946.08 ± 3.63 μS/cm respectively than for typically unpolluted river of 350 μS/cm [ 75 ]. Consequently, given that the two rivers flow through areas of heavy metal mining (copper and cobalt mines in Kasese district by Kilembe Mines Limited and Kasese Cobalt Company Limited) and industrial activities (Kampala City), it is possible for the high water conductivity to be due to the heavy metal contamination as previously documented in drinking water in South-western Uganda [ 62 ] and Kampala City [ 61 ]. Thus, specific studies are required on water from the two rivers to determine the true cause of the high conductivity and to guide mitigation measures.
Hence, more efforts are required to promote safe water access in Uganda to attain the WHO cholera elimination target [ 25 ] and SDG 6 by 2030 since 26% (36/135) of mean physicochemical water tests did not meet WHO drinking water quality standards [ 53 ]. These findings together with those of the previous studies which demonstrated the presence of pathogenic V. cholerae in the same water sources [ 22 , 23 , 76 ] should guide stakeholders to improve access to safe water in the Great Lakes basins of Uganda holistically. Thus, measures such as promotion of use of safe water (using water disinfection), health education, sanitation improvement and hygiene promotion that address both the water bacteriological contents and physicochemical parameters should be considered in both the short and medium terms. However, long term plan to increase access to safe water by construction of permanent safe water treatment plants and distribution systems (pipes) should remain a top priority.
In the short and intermediate period, focusing on the measures that reduce water turbidity and disinfection of water (to kill microorganisms) should be prioritized so as to facilitate progress towards attainment of SDGs and cholera elimination in the study area. The basis for such prioritization lies in the fact that high water turbidity raises water temperature and prevents the disinfection effects of chlorine on water. These in return promote survival of the microorganisms and consequently cholera and other waterborne disease outbreaks. Furthermore, though boiling of water is feasible and recommended through technical guidelines [ 26 ] since it addresses both turbidity and kills the micro-organisms, it has issues of poor compliance due to lack of firewood which is the main cooking energy source in these communities [ 70 ]. Therefore, alternative safe water provision targeting reduction of high water turbidity and removal of microorganism by special filters such as decanting and sand filters and flocculation agents which do not need heat energy should be promoted [ 77 , 78 ]. Also, there is a need to explore the use of solar energy (solar water purifiers) [ 79 ] in these communities given their location in the tropics where sunshine is plenty. In the minority of situations, in addition to use of above methods to make water safe, there may be a need to employ different approaches of water purification depending on the water source. For example the water sources with lower or higher than recommended pH [ 53 ] (Wanseko pond, Hamukungu and Kahendero FLS on L. George), use of water treatment reagents that are affected by pH such as chlorine tablets should be reevaluated.
In additional to disinfection and turbidity corrective measures for all the water that were studied, each of the springs in the study area (Katanga in Kampala district and Nyakirango and Kibenge springs in Kasese district) will also need a sanitary survey (a comprehensive inspection of the entire water delivery system from the source to the mouth so as to identify potential problems and changes in the quality of drinking water) [ 80 ]. The findings of the sanitary survey should then guide the medium and long term interventions for water quality improvement in areas served by targeted springs. The following are some of the interventions that could be carried out after a sanitary survey: provision of a screen to prevent the entrance of animals, erecting a warning signs, digging of a diversion ditch located at the uphill end to keep rainwater from flowing over the spring area, establishment of an impervious barrier (a clay or a plastic liner) to prevent potential contaminants from entering into the water or and others measures described in the handbook for spring protection [ 81 ].
Furthermore, as a stopgap measure while access to safe water is scaled up, the communities in the study area should be protected from cholera using Oral Cholera Vaccines [ 82 ]. Protection of these communities is necessary since this study shows that favorable conditions for cholera propagation/transmission are present in the water in the study area. The favorable conditions that were documented in this study included the high water turbidity which makes it difficult to disinfect water [ 53 ] and the water temperature of above 17 °C which speeds up the multiplication of pathogens [ 43 ].
In addition, there were some other important study findings that were not fully understood. For example, some water sources (Kibenge spring and pond (located in Kasese district, western Uganda) had extreme vital physicochemical values for both conductivity and water temperature relative to the rest of above 40 °C and 3000 μS/cm respectively. It is possible that the extreme values were due to geochemical effects documented in water sources around Mount Rwenzori [ 83 ]. However, since there was copper and cobalt mining in Kasese district, high water conductivity could have been due to chemical contamination. Similarly, River Lubigi, Kampala district (central Uganda) had very low dissolved oxygen of less than 1 mg/L during some months (for example in January 2015, dissolved oxygen of 0.45 mg/L) which could have been due to organic pollutants from the communities in Kampala City [ 84 ] that used up the oxygen in the water. Also, Wanseko pond (Lake Albert basin, Buliisa district) had low pH of 4.84 in February 2015. Such water with low pH have the potential to increase the solubility of heavy metals some of which make water harmful when consumed [ 85 ]. Therefore, further studies will be required to better understand such extreme values.
Strength and limitations of this study
This study had several strengths. First, the longitudinal study design that employed repeated measurements of water physicochemical characteristics from the same site and source. This design reduced the likelihood of errors that could arise from one-off measurements seen in cross-sectional study designs resulting in increased validity of the study findings. Second, the inclusion of a variety of the water sources from which drinking and domestic water were collected namely, lakes, rivers, ponds, springs and a canal from different regions of Uganda made the findings representative of the water sources in study districts. Third, use of robust equipment, Hach meters, HQ40d [ 68 ] which automatically compensated for the weather changes (corrected for possible confounders and biases) for the parameters that had effect on each other such as raising water temperature impacting on the water conductivity and dissolved oxygen. Forth, purposive selection of the districts with frequent cholera outbreaks, an important waterborne disease that is targeted for elimination locally within Uganda and globally by WHO [ 25 ]. This meant that the findings had higher potential for used by stakeholders targeting to improve access to safe water and those for cholera prevention.
There were also some study limitations. First, though the study identified the favourable conditions (higher than recommended mean water turbidity and temperature of above 17 °C) for cholera in the study area, we could not report on causal-effect relationship between V. cholerae and the parameters studied. Vibrio cholera e pathogens were detected by use of multiplex Polymerase Chain Reaction (PCR). The results for PCR test were interpreted as positive or negative for V. cholerae O1, O139, non O1, and non O139 [ 22 ]. These data were not appropriate for establishment of causal-effect relationship Therefore, further studies using appropriate methods are recommended to establish such relationships.
Second, during some months of the study, water samples could not be obtained from some sources especially the ponds that had dried up during the dry season. The drying up reduced the number of samples collected from these points. However, since the months without water were few compared to the entire study period, the impact of the missing data could have been minimal.
Third, water samples were only tested for the five key physicochemical water characteristics, Vital Signs [ 32 ] however, there are many other parameters that effect survival and health of living things namely, nitrates, copper, lead, fluoride, phosphates, arsenic and others. Studies are therefore required to provide more information on these other parameters not addressed by the current study.
The study showed that surface and spring water for drinking and other domestic purposes in cholera prone communities in Great Lakes basins of Uganda were unsafe in terms of vital physicochemical water characteristics. These water sources had favourable physicochemical characteristics for transmission/propagation of waterborne diseases, including cholera. All test sites (100%, 27/27) had temperature above 17 °C that is suitable for V. cholerae survival and transmission and higher than the WHO recommended mean water turbidity of 5NTU. In addition, more than a quarter (27%) of lake sites and 40% of the ponds had pH and dissolved oxygen outside the WHO recommended range of 6.5–8.5 and less than 5 mg/L respectively. These findings complement bacteriological findings that were previously reported in the study area which found that use of this water increased their vulnerability to cholera outbreaks [ 22 ]. Therefore, in order for Uganda to attain the WHO cholera elimination and the United Nations SDG 6 target by 2030, stakeholders (the Ministry of Water and Environment, the local governments, Ministry of Health development partners and others) should embrace interventions that holistically improve water quality through addressing both physicochemical and biological characteristics. Furthermore, studies should be conducted to generate more information on the other physicochemical parameters not included in this study such as detection of the heavy metal contamination.
Availability of data and materials
The datasets generated and/or analysed during the current study are available in the Mendeley Data repository, https://doi.org/10.17632/57sw2w23tw.1 . The cholera incidence data used to identify the study area were from Uganda Ministry of Health and the district (Kasese, Busia, Nebbi, Buliisa and Kayunga) weekly epidemiological reports.
Abbreviations
Analysis of Variance
Conductivity
Central Public Health Laboratories
Dissolved Oxygen
Delivery of Oral Vaccines Effectively
Fish landing site
Institutional Review Board
Ministry of Health
Nephrometric units
Polymerase Chain Reaction
Sustainable Development Goal
Standard Operating Procedures
United States of America
World Health Organization
Walther J. Earth’s natural resources. Jones & Bartlett Learning; 2014. https://www.researchgate.net/publication/267327567_EARTH’S_NATURAL_RESOURCES/link/544e7df80cf2bca5ce90b65b/download .
UNDP. Sustainable Development GOALS 2030. 2015.
Nikanorov AM, Brazhnikova LV. Water chemical composition of Rivers, lakes and wetlands. Encycl Life Support Syst. 2009;2:42–80 https://www.eolss.net/Sample-Chapters/C07/E2-03-04-02.pdf .
Google Scholar
United Nations. International Decade for Action “Water for Life” 2005–2015. Focus Areas: The human right to water and sanitation1. UN. International Decade for Action “Water for Life” 2005–2015. Focus Areas: The human right to water and sanitation [Internet]. United Nati. United Nations. 2014. http://www.un.org/waterforlifedecade/human_right_to_water.shtml . Accessed 2 Dec 2019.
UNDP. Goal 6: Clean water and sanitation | UNDP. UNDP. 2015. https://www.undp.org/content/undp/en/home/sustainable-development-goals/goal-6-clean-water-and-sanitation.html . Accessed 29 Nov 2019.
Programme UNE. Africa water atlas. Nairobi, Kenya: United Nations Environment Programme; 2010. https://www.zaragoza.es/contenidos/medioambiente/onu/340-eng.pdf .
Dos Santos S, Adams EA, Neville G, Wada Y, de Sherbinin A, Mullin Bernhardt E, et al. Urban growth and water access in sub-Saharan Africa: progress, challenges, and emerging research directions. Sci Total Environ. 2017;607–8:497–508. https://doi.org/10.1016/j.scitotenv.2017.06.157 .
Walker C. Lack of safe water, sanitation spurs growing dissatisfaction with government performance. 2016. http://afrobarometer.org/countries/results-round . Accessed 9 July 2020.
WHO/UNICEF JMP. Progress on household drinking water , sanitation and hygiene I 2000-2017. Special Focus on Inequalities 2017. https://washdata.org . Accessed 3 Dec 2019.
Uganda Bureu of Statistics. Uganda Bureau of Statistics Education Sector Gender Statistics Profile November 2012. 2012; November:1–43.
University of Califonia Museum of Paleontology (UCMP). The Aquatic Biome. 2014;:1–4. http://www.ucmp.berkeley.edu/glossary/gloss5/biome/aquatic.html . Accessed 3 Aug 2017.
World Health Organization. Protecting Surface Water for Health. 2016. http://apps.who.int/iris/bitstream/10665/246196/1/9789241510554-eng.pdf?ua=1 . Accessed 3 Aug 2017.
Hunter PR. Climate change and waterborne and vector-borne disease. J Appl Microbiol Symp Suppl. 2003;94. https://doi.org/10.1046/j.1365-2672.94.s1.5.x .
Vitousek PM, Mooney HA, Lubchenco J, Melillo JM. Human domination of Earth’s ecosystems. In: Urban Ecology: An International Perspective on the Interaction Between Humans and Nature 2008.
World Health Organization. WHO | Water-related Diseases. https://www.who.int/water_sanitation_health/diseases-risks/diseases/diarrhoea/en/ . Accessed 9 July 2020.
World Health Organization. Weekly epidemiological record Relevé épidémiologique hebdomadaire. 2013;:321–36.
World Health Organization. Weekly epidemiological record Relevé épidémiologique hebdomadaire. 2019;94:561–80. https://extranet.who.int/iris/restricted/bitstream/handle/10665/330003/WER9448-eng-fre.pdf?ua=1 .
Bwire G, Malimbo M, Maskery B, Kim YE, Mogasale V, Levin A. The burden of cholera in Uganda. PLoS Negl Trop Dis. 2013;7:e2545. https://doi.org/10.1371/journal.pntd.0002545 .
Article PubMed PubMed Central Google Scholar
Bwire G, Munier A, Ouedraogo I, Heyerdahl L, Komakech H, Kagirita A, et al. Epidemiology of cholera outbreaks and socio-economic characteristics of the communities in the fishing villages of Uganda: 2011-2015. PLoS Negl Trop Dis. 2017;11.
Kwesiga B, Pande G, Ario AR, Tumwesigye NM, Matovu JKB, Zhu BP. A prolonged, community-wide cholera outbreak associated with drinking water contaminated by sewage in Kasese District, western Uganda. BMC Public Health. 2017;18. https://doi.org/10.1186/s12889-017-4589-9 .
Pande G, Kwesiga B, Bwire G, Kalyebi P, Riolexus AA, Matovu JKB, et al. Cholera outbreak caused by drinking contaminated water from a lakeshore water-collection site, Kasese District, South-Western Uganda, June-July 2015. PLoS One. 2018;13:e0198431. https://doi.org/10.1371/journal.pone.0198431 .
Article CAS PubMed PubMed Central Google Scholar
Bwire G, Debes AK, Orach CG, Kagirita A, Ram M, Komakech H, et al. Environmental surveillance of Vibrio cholerae O1/O139 in the five African Great Lakes and other major surface water sources in Uganda. Front Microbiol. 2018;9:1560. https://doi.org/10.3389/FMICB.2018.01560 .
Kaddumukasa M, Nsubuga D, Muyodi FJ. Occurence of Culturable Vibrio cholerae from Lake Victoria, and Rift Valley lakes Albert and George, Uganda. Lakes Reserv Res Manag. 2012;17:291–9.
Article CAS Google Scholar
Oguttu DW, Okullo A, Bwire G, Nsubuga P, Ario AR. Cholera outbreak caused by drinking lake water contaminated with human faeces in Kaiso Village, Hoima District, Western Uganda, October 2015. Infect Dis Poverty. 2017;66:146. https://doi.org/10.1186/s40249-017-0359-2 .
World Health Organization. Ending cholera: A global roadmap to 2030. 2017. http://www.who.int/cholera/publications/global-roadmap.pdf?ua=1 . Accessed 9 July 2020.
Ministry of Health Uganda. Prevention and Control of Cholera Operational Guidelines for the National and District Health Workers and Planners. 2017. http://www.health.go.ug/sites/default/files/Final CHOLERA GUIDELINES 2017_0.pdf. Accessed 13 Feb 2019.
Snow J. Mode of Communication of Cholera (John Snow, 1855). 1855;:1–38. http://www.ph.ucla.edu/epi/snow/snowbook.html .
Bingham P, Verlander NQ, Cheal MJ. John snow, William Farr and the 1849 outbreak of cholera that affected London: a reworking of the data highlights the importance of the water supply. Public Health. 2004;118:387–94.
United Nations Environment Programme and the World Health Organization. United Nations Environment Programme and the World Health Organization. 1996.
Daud MK, Nafees M, Ali S, Rizwan M, Bajwa RA, Shakoor MB, et al. Drinking Water Quality Status and Contamination in Pakistan 2017;2017.
Ford L, Bharadwaj L, Mcleod L, Waldner C. Human health risk assessment applied to rural populations dependent on unregulated drinking water sources : a scoping review. 2017.
Team CW. Vital Signs : The Five Basic Water Quality Parameters. 2010;:3–4.
Fondriest Environmental, Inc. “pH of Water” Fundamentals of Environmental Measurements. 2013. Web. https://www.fondriest.com/environmentalmeasurements/parameters/water-quality/ph/ . Accessed 7 July 2020.
Vancouver Water Resources Education Center. Water Quality: Temperature, pH and Dissolved Oxygen. 2019. www.middleschoolchemistry.com/lessonplans/ . Accessed 5 Dec 2019.
Fondriest Environmental, Inc. “Water Quality” Fundamentals of Environmental Measurements. 2013. Web. https://www.fondriest.com/environmentalmeasurements/parameters/water-quality/ . Accessed 7 July 2020.
Spellman FR. The Drinking Water Handbook 2017. doi: https://doi.org/10.1201/9781315159126 .
World Health Organization. Protecting Surface Water for health: Identifying, assessing and managing drinking-water quality risks in surface-water catchments. 2016. http://www.who.int . Accessed 4 July 2019.
Bliem R, Reischer G, Linke R, Farnleitner A, Kirschner A. Spatiotemporal dynamics of Vibrio cholerae in turbid alkaline lakes as determined by quantitative PCR. Appl Environ Microbiol. 2018;84:317–35. https://doi.org/10.1128/AEM.00317-18 .
U.S. Environmental Protection Agency. Drinking Water Treatability Database: Vinclozolin. 2009. https://iaspub.epa.gov/tdb/pages/contaminant/contaminantOverview.do%3FcontaminantId%3D10540 . Accessed 5 Dec 2019.
Sagir Ahmed M, Raknuzzaman M, Akther H, Ahmed S. The role of cyanobacteria blooms in cholera epidemic in Bangladesh. J Appl Sci. 2007;7:1785–9.
Article Google Scholar
Epstein PR. Algal blooms in the spread and persistence of cholera. BioSystems. 1993;31:209–21. https://doi.org/10.1016/0303-2647(93)90050-M .
Article CAS PubMed Google Scholar
Gil AI, Louis VR, Rivera ING, Lipp E, Huq A, Lanata CF, et al. Occurrence and distribution of Vibrio cholerae in the coastal environment of Peru. Environ Microbiol. 2004;6:699–706.
Nair G. B, Havelaar A. H, Bartram J, Jacob J, Hueb J et al. Vibrio cholerae 1. 1997;:119–42. http://www.who.int/water_sanitation_health/dwq/admicrob6.pdf .
Roberts MB V. Biology: a functional approach. 1986. https://books.google.co.ug/books?id=ASADBUVAiDUC&pg=PA232&lpg=PA232&dq=body+temperatures+of+microorganisms+the+same+as+surroundings+poikilothermic&source=bl&ots=hGQDzoXNw2&sig=ACfU3U3H3-n-YybOibjEg_Q_dOmSdu1L5Q&hl=en&sa=X&ved=2ahUKEwjAy5zerJ_mAhUICsAKHU5b . Accessed 5 Dec 2019.
Fondriest Environmental I. Conductivity, Salinity & Total Dissolved Solids - Environmental Measurement Systems. Fundamentals of Environmental Measurements, Fondriest Environmental, Inc. 2014;:Web. https://www.fondriest.com/environmental-measurements/parameters/water-quality/conductivity-salinity-tds/ . Accessed 19 Dec 2019.
Queensland G. Environmental protection (water) policy 2009 - monitoring and sampling manual physical and chemical assessment. 2018. https://environment.des.qld.gov.au/water/monitoring/sampling-manual/pdf/physical-and-chemical-assesssment-swab-sampling.pdf . Accessed 29 Nov 2019.
Fondriest Environmental, Inc. “Turbidity-total-suspended-solids-water-clarity” Fundamentals of Environmental Measurements. 2013. Web. https://www.fondriest.com/environmental-measurements/parameters/water-quality/turbiditytotal-suspended-solids-water-clarity/ . Accessed 7 July 2020.
Otieno OS. Physico-chemical and bacteriological quality of water from five rural catchment areas of Lake Victoria basin in Kenya 2015. doi: https://doi.org/10.1017/CBO9781107415324.004 .
Kelley CD, Krolick A, Brunner L, Burklund A, Kahn D, Ball WP, et al. An affordable open-source turbidimeter. Sensors (Switzerland). 2014;14:7142–55. https://doi.org/10.3390/s140407142 .
Sagir Ahmed M, Raknuzzaman M, Akther H, Ahmed S. The role of cyanobacteria blooms in cholera epidemic in Bangladesh. J Appl Sci. 2007;7:1785–9. https://doi.org/10.3923/jas.2007.1785.1789 .
Gorchev HG, Ozolins G. WHO guidelines for drinking-water quality 2011. doi: https://doi.org/10.1016/S1462-0758(00)00006-6 .
World Health Organization. Essential environmental health standards in health care. Non serial. Geneva: World Health Organization Press; 2008. https://books.google.co.ug/books?hl=en&lr=&id=QwVj87Mmz4sC&oi=fnd&pg=PA3&dq=Essential+environmental+health+standards+in+health+care.+World+Health++Organization&ots=qBUjaQCxQV&sig=CyG-4NLSRKIEZG-L0vDxuK5Ga-o&redir_esc=y#v=onepage&q=Essential%20environmental . Accessed 6 July 2020.
World Health Organization. Guidelines for Drinking Water Quality. Fourth Edi. 2017. https://apps.who.int/iris/bitstream/handle/10665/254636/9789241550017-eng.pdf;jsessionid=B0351A975B001E76D924D2B1183DE673?sequence=1 .
Sharma S, Bhattacharya A. Drinking water contamination and treatment techniques. Appl Water Sci. 2017;7:1043–67. https://doi.org/10.1007/s13201-016-0455-7 .
Centers for Disease Control and Prevention (CDC); Cholera prevention and control. Atlanta, USA. https://www.cdc.gov/cholera/pdf/five-basic-cholera-prevention-messages.pdf . Accessed 10 July 2020.
Luff R. Oxfam guidelines for water treatment in emergencies. 2001. http://ec.europa.eu/echo/files/evaluation/watsan2005/annex_files/OXFAM/OXF5 - Oxfam guidelines for water treatment in emergencies. PDF.
Iceland Kasozi K, Namubiru S, Kamugisha R, Daniel Eze E, Stuart Tayebwa D, Ssempijja F, et al. Safety of Drinking Water from Primary Water Sources and Implications for the General Public in Uganda 2019. doi: https://doi.org/10.1155/2019/7813962 .
Alajo S. Comparison of two communities affected by cholera in Kasese district in Uganda. Am J Trop Med Hyg. 2013;89:205 http://www.embase.com/search/results?subaction=viewrecord&from=export&id=L71312507%5Cnhttp://www.ajtmh.org/content/89/5_Suppl_1/153.full.pdf+html%5Cnhttp://vb3lk7eb4t.search.serialssolutions.com?sid=EMBASE&issn=00029637&id=doi:&atitle=Comparison+of+two+co.
Jutla A, Whitcombe E, Hasan N, Haley B, Akanda A, Huq A, et al. Environmental factors influencing epidemic cholera. Am J Trop Med Hyg. 2013;89:597–607. https://doi.org/10.4269/ajtmh.12-0721 .
Ojok W, Wasswa J, Ntambi E. Assessment of seasonal variation in water quality in river Rwizi using multivariate statistical techniques, Mbarara municipality, Uganda. J Water Resour Prot. 2017;09:83–97.
Semuyaba AS, Segawa I, Wamala A. Potential risk of Lead toxicity from bottled water in Uganda. MPJ. 2014;12:e13–20 https://chs.mak.ac.ug/content/potential-risk-lead-toxicity-bottled-water-uganda . Accessed 18 Dec 2019.
Kasozi KI, Namubiru S, Kamugisha R, Eze ED, Tayebwa DS, Ssempijja F, et al. Safety of drinking water from primary water sources and implications for the general public in Uganda. J Environ Public Health. 2019;2019. https://doi.org/10.1155/2019/7813962 .
Okello PE, Bulage L, Riolexus AA, Kadobera D, Kwesiga B, Kajumbula H, et al. A cholera outbreak caused by drinking contaminated river water, Bulambuli District, eastern Uganda, march 2016. BMC Infect Dis. 2019;19. https://doi.org/10.1186/s12879-019-4036-x .
Agensi A, Tibyangye J, Tamale A, Agwu E, Amongi C. Contamination potentials of household water handling and storage practices in Kirundo subcounty, Kisoro District, Uganda. J Environ Public Health. 2019;2019. https://doi.org/10.1155/2019/7932193 .
Bwire G, Ali M, Sack DA, Nakinsige A, Naigaga M, Debes AK, et al. Identifying cholera “hotspots” in Uganda: an analysis of cholera surveillance data from 2011 to 2016. PLoS Negl Trop Dis. 2017;11:e0006118. https://doi.org/10.1371/journal.pntd.0006118 .
Bompangue Nkoko D, Giraudoux P, Plisnier P-D, Tinda AM, Piarroux M, Sudre B, et al. Dynamics of cholera outbreaks in Great Lakes region of Africa, 1978-2008. Emerg Infect Dis. 2011;17:2026–34.
PubMed Google Scholar
Debes AK, Ateudjieu J, Guenou E, Lopez AL, Bugayong MP, Retiban PJ, et al. Evaluation in Cameroon of a novel, simplified methodology to assist molecular microbiological analysis of v. cholerae in resource-limited settings. PLoS Negl Trop Dis. 2016;10:4307. https://doi.org/10.1371/journal.pntd.0004307 .
Hach campany. HQ Series Portable Meters. 2006. www.hach.com . Accessed 4 Jan 2019.
Langan M. Budget support and Africa–European Union relations: free market reform and neo-colonialism? Eur J Int relations. 2015.
UNICEF. UNICEF Handbook On Water Quality. 2008. http://www.unicef.org/wes . Accessed 14 Dec 2019.
Ouma S, Ngeranwa JN, Juma KK, Mburu DN. Journal of environmental analytical seasonal variation of the physicochemical and bacteriological quality of water from five rural catchment areas of Lake Victoria Basin in Kenya. J Environ Anal Chem. 2016;3:1–7. https://doi.org/10.4172/2380-2391.1000170 .
Mapira J. River pollution in the City of Mutare (Zimbabwe) and its implications for sustainable development. J Sustain Dev Africa. 2011;13:181–94. https://pdfs.semanticscholar.org/a581/6beb20e79ea0590a7717de4a095c02dc14a5.pdf . Accessed 4 July 2019.
Colwell RR, Huq A. Chapter 9: Vibrios in the environment: viable but nonculturable Vibrio cholerae. In: Wachsmuth, Kaye I, Blake P, Olsvik Ø, editors. Vibrio cholerae and Cholera. Washington, DC: American Society of Microbiology; 1994. p. 117–33. https://www.asmscience.org/content/book/10.1128/9781555818364.chap9 . Accessed 9 July 2020.
Colwell RR, Spira WM. The ecology of Vibrio cholerae. In: Cholera. Boston, MA: Springer US; 1992. p. 107–27. https://doi.org/10.1007/978-1-4757-9688-9_6 .
Chapter Google Scholar
Koning N, Roos JC. The continued influence of organic pollution on the water quality of the turbid Modder River. Water SA. 1999;25:285–92.
CAS Google Scholar
Bagalwa M, Yalire M, Balole E, Karume K. A preliminary assessment of Physico-chemical and bacteriological characteristics of Lake Eduard and Majors tributaries Rivers, Democratic Republic of Congo. Sch Acad J Biosci. 2014;2:236–45 www.saspublisher.com . Accessed 29 July 2017.
Collin C. Biosand filtration of high turbidity water: modified filter design and safe filtrate storage. Massachusetts Institute of Technology; 2009. http://web.mit.edu/watsan/Docs/Student%20Theses/Ghana/2009/Thesis%20-%20Final,%20Clair%20Collin,%205-15-09.pdf . Accessed 9 July 2020.
Collin C. Biosand filtration of high turbidity water: modified filter design and safe filtrate storage. Massachusetts Institute of Technology; 2009. https://dspace.mit.edu/handle/1721.1/50623 . Accessed 7 July 2020.
Graf J, Togouet SZ, Kemka N, Niyitegeka D, Meierhofer R, Pieboji JG. Health gains from solar water disinfection (SODIS): Evaluation of a water quality intervention in Yaoundé, Cameroon. J Water Health. 2010;8:779–96. https://doi.org/10.2166/wh.2010.003 .
Open University. Hygiene and environmental health module: 16. Sanitary Survey of Drinking Water 2019. https://www.open.edu/openlearncreate/mod/oucontent/view.php?id=203&printable=1 . Accessed 6 Jun 2020.
Herrmann H, Bucksch H. spring protection. In: Dictionary Geotechnical Engineering/Wörterbuch GeoTechnik. 2014. p. 1290–1290.
World Health Organization. Oral Cholera Vaccine stockpile for cholera emergency response. 2013. http://www.who.int/cholera/vaccines/Briefing_OCV_stockpile.pdf .
Kato V, Kraml M. Geochemistry of Rwenzori hot spring waters. 2010; November:22–5. https://www.geothermal-energy.org/pdf/IGAstandard/ARGeo/2008/V.Kato_Rwenzori.pdf . Accessed 4 Jan 2019.
Kayima JK, Mayo AW, Nobert J. Ecological Characteristics and Morphological Features of the Lubigi Wetland in Uganda. Environ Ecol Res. 2018;6:218–28. https://doi.org/10.13189/eer.2018.060402 ..
Ozoko DC. Heavy metal geochemistry of acid mine drainage in Onyeama coal mine, Enugu, Southeastern Nigeria. J Environ Earth Sci. 2015.
Download references
Acknowledgements
The authors are grateful to the following: the district teams and the communities in Kasese, Kampala, Nebbi, Buliisa, Kayunga and Busia districts for the cooperation and support; the Ministry of Health, Makerere University School of Public Health, Dr. Asuman Lukwago, Dr. Jane Ruth Aceng and Prof. AK. Mbonye for technical guidance. The authors are grateful to Dunkin Nate from John Hopkins University for training of the field teams on water sampling and testing. The authors also thank Ambrose Buyinza Wabwire and to Damari Atusasiire for the support in creating the map and statistical guidance respectively. Special thanks to the laboratory teams in the district hospitals; CPHL (Kampala) and John Hopkins University (Maryland, USA) for carrying out the water tests.
This study was funded by the Bill and Melinda Gates Foundation, USA, through John Hopkins University under the Delivering Oral Vaccine Effectively (DOVE) project. (OPP1053556). The funders had no role in the implementation of the study and in the decision to publish the study findings.
Author information
Authors and affiliations.
Department of Community and Behavioral Sciences, Makerere University College of Health Sciences, School of Public Health, Kampala, Uganda
Godfrey Bwire, Henry Komakech & Christopher Garimoi Orach
Department of International Health, Johns Hopkins Bloomberg School of Public Health, Dove Project, Baltimore, MD, USA
David A. Sack, Amanda K. Debes, Malathi Ram & Christine Marie George
Uganda National Health Laboratory Services (UNHS/CPHL), Ministry of Health, Kampala, Uganda
Atek Kagirita
Department of Quality Control, Uganda National Drug Authority, Kampala, Uganda
Tonny Obala
You can also search for this author in PubMed Google Scholar
Contributions
GB, DAS, AKD and CGO conceived the idea. GB, CGO, AKD, MR, HK, AK, TO and CMG conducted the investigation. MR, HK and TO carried out data curation. MR, HK, GB, DAS, CMG, AKD and AK analysed data. GB, DAK, AKD, CGO, MR, AK, TO and CMG wrote the first draft. All authors read and approved the final manuscript.
Corresponding author
Correspondence to Godfrey Bwire .
Ethics declarations
Ethics approval and consent to participate.
This study was approved by the Makerere University School of Public Health Institution Review Board (IRB 00011353) and the Uganda National Council of Science and Technology. Cholera data used in selection of the water bodies and study communities were aggregated disease surveillance data from the Ministry of Health with no personal identifiers. The laboratory reports on the water sources found contaminated during the study period were shared immediately with the district team to ensure that preventive measures were instituted to protect the communities. In addition, the communities served by such water sources were educated on water treatment/purification (filtration, boiling, chlorination, use of Waterguard ).
Consent for publication
Not applicable.
Competing interests
The authors report no competing interests.
Additional information
Publisher’s note.
Springer Nature remains neutral with regard to jurisdictional claims in published maps and institutional affiliations.
Supplementary information
Additional file 1..
The number and the type of water sources in each of the lake basins in cholera prone communities of Uganda that were enrolled in the study, February 2015 – January 2016.
Additional file 2.
One Way ANOVA test results for the differences within the study sites overtime (February 2015 – January 2016) and between sites.
Rights and permissions
Open Access This article is licensed under a Creative Commons Attribution 4.0 International License, which permits use, sharing, adaptation, distribution and reproduction in any medium or format, as long as you give appropriate credit to the original author(s) and the source, provide a link to the Creative Commons licence, and indicate if changes were made. The images or other third party material in this article are included in the article's Creative Commons licence, unless indicated otherwise in a credit line to the material. If material is not included in the article's Creative Commons licence and your intended use is not permitted by statutory regulation or exceeds the permitted use, you will need to obtain permission directly from the copyright holder. To view a copy of this licence, visit http://creativecommons.org/licenses/by/4.0/ . The Creative Commons Public Domain Dedication waiver ( http://creativecommons.org/publicdomain/zero/1.0/ ) applies to the data made available in this article, unless otherwise stated in a credit line to the data.
Reprints and permissions
About this article
Cite this article.
Bwire, G., Sack, D.A., Kagirita, A. et al. The quality of drinking and domestic water from the surface water sources (lakes, rivers, irrigation canals and ponds) and springs in cholera prone communities of Uganda: an analysis of vital physicochemical parameters. BMC Public Health 20 , 1128 (2020). https://doi.org/10.1186/s12889-020-09186-3
Download citation
Received : 02 April 2019
Accepted : 01 July 2020
Published : 17 July 2020
DOI : https://doi.org/10.1186/s12889-020-09186-3
Share this article
Anyone you share the following link with will be able to read this content:
Sorry, a shareable link is not currently available for this article.
Provided by the Springer Nature SharedIt content-sharing initiative
- Drinking water
- Water quality
- Physicochemical parameter
- Water source
- Surface water
BMC Public Health
ISSN: 1471-2458
- General enquiries: [email protected]

An official website of the United States government
The .gov means it’s official. Federal government websites often end in .gov or .mil. Before sharing sensitive information, make sure you’re on a federal government site.
The site is secure. The https:// ensures that you are connecting to the official website and that any information you provide is encrypted and transmitted securely.
- Publications
- Account settings
Preview improvements coming to the PMC website in October 2024. Learn More or Try it out now .
- Advanced Search
- Journal List
- HHS Author Manuscripts

Challenges to Sustainable Safe Drinking Water: A Case Study of Water Quality and Use across Seasons in Rural Communities in Limpopo Province, South Africa
Joshua n. edokpayi.
1 Department of Hydrology and Water Resources, University of Venda, Thohoyandou 0950, South Africa; [email protected]
2 Department of Civil and Environmental Engineering, University of Virginia, Charlottesville, VA 22904, USA; ude.qud@drelhak (D.M.K.); moc.liamg@320hlc (C.L.H.); ude.ainigriv@sm4rfc (C.R.); ude.ainigriv@e9saj (J.A.S.)
Elizabeth T. Rogawski
3 Department of Public Health Sciences, University of Virginia, Charlottesville, VA 22908, USA; ude.ainigriv@m5rte
4 Division of Infectious Diseases & International Health, University of Virginia, Charlottesville, VA 22908, USA; ude.ainigriv.ccm.liamcsh@v8dr
David M. Kahler
5 Center for Environmental Research and Education, Duquesne University, Pittsburgh, PA 15282, USA
Courtney L. Hill
Catherine reynolds.
6 School of Civil and Environmental Engineering, Georgia Institute of Technology, Atlanta, GA 30332, USA
Emanuel Nyathi
7 Department of Animal Science, University of Venda, Thohoyandou 0950, South Africa; [email protected]
James A. Smith
John o. odiyo, amidou samie.
8 Department of Microbiology, University of Venda, Thohoyandou 0950, South Africa; [email protected] (A.S.); [email protected] (P.B.)
Pascal Bessong
Rebecca dillingham.
Author Contributions: Conceived and designed the experiments: J.N.E., E.T.R., D.M.K., C.L.H. Performed the experiments: J.N.E., E.T.R., D.M.K., C.L.H., C.R., E.N. Contributed reagents/materials/analysis tools: P.B., E.N., A.S., R.D., J.A.S., J.O.O. Analyzed the data: J.N.E., E.T.R., D.M.K., C.L.H. Wrote the paper: J.N.E., E.T.R., D.M.K., C.L.H. Participated in the editing of the manuscript: J.N.E., E.T.R., D.M.K., C.L.H., P.B., A.S., R.D., J.A.S., J.O.O., E.N., C.R.
Associated Data
Table S2: Membrane-filtration results for E. Coli and total coliforms of water sources,
Table S3: Anion concentrations (mg/L) of water sources,
Table S4: Major metal concentrations (mg/L) of water sources,
Table S5: Trace metal concentrations μg/L) of water sources.
Consumption of microbial-contaminated water can result in diarrheal illnesses and enteropathy with the heaviest impact upon children below the age of five. We aimed to provide a comprehensive analysis of water quality in a low-resource setting in Limpopo province, South Africa. Surveys were conducted in 405 households in rural communities of Limpopo province to determine their water-use practices, perceptions of water quality, and household water-treatment methods. Drinking water samples were tested from households for microbiological contamination. Water from potential natural sources were tested for physicochemical and microbiological quality in the dry and wet seasons. Most households had their primary water source piped into their yard or used an intermittent public tap. Approximately one third of caregivers perceived that they could get sick from drinking water. All natural water sources tested positive for fecal contamination at some point during each season. The treated municipal supply never tested positive for fecal contamination; however, the treated system does not reach all residents in the valley; furthermore, frequent shutdowns of the treatment systems and intermittent distribution make the treated water unreliable. The increased water quantity in the wet season correlates with increased treated water from municipal taps and a decrease in the average contaminant levels in household water. This research suggests that wet season increases in water quantity result in more treated water in the region and that is reflected in residents’ water-use practices.
1. Introduction
Clean and safe drinking water is vital for human health and can reduce the burden of common illnesses, such as diarrheal disease, especially in young children. Unfortunately, in 2010, it was estimated that 1.8 billion people globally drank water that was not safe [ 1 ]. This scenario is most common in developing countries, and the problem is exacerbated in rural areas [ 1 ]. Significant amounts of time are spent by adults and school children upon water abstraction from various sources [ 2 , 3 ]. It is estimated that, in developing countries, women (64%) and girls (8%) spend billions of hours a year collecting water [ 1 ]. The erratic supply of safe drinking and domestic water often affects good hygiene practices. In most developing countries of the world, inadequate supplies of drinking water can contribute to the underage death of children in the region [ 4 – 10 ].
Storage of collected water from rivers, springs, community stand-pipes, and boreholes is a common practice in communities that lack potable water supplies piped into their homes. Even when water is piped into the home, it is often not available on a continuous basis, and water storage is still necessary. Water is stored in various containers which include jerry cans, buckets, drums, basins and local pots [ 11 – 13 ]. It has been reported that when collection of water from sources of high quality is possible, contamination during transport, handling and storage and poor hygienic practices often results and can cause poor health outcomes [ 11 , 13 – 15 ].
South Africa is a semi-arid country that has limited water resources, and the provision of adequate water-supply systems remains a great challenge. In some of the major cities, access to clean and safe drinking water is comparable to what is found in other developed cities, but this is not the case in some cities, towns and most villages where there is constant erratic supply of potable water, and in some cases, there is no water supply system [ 16 ]. Although access to clean and safe drinking water is stipulated as a constitutional right for all South Africans in the country’s constitution [ 17 , 18 ], sustainable access to a potable water supply by millions of South Africans is lacking.
Residents of communities with inadequate water supply are left with no alternative other than to find local sources of drinking water for themselves. Rural areas are the most affected, and residents resort to the collection of water from wells, ponds, springs, lakes, rivers and rainwater harvesting to meet their domestic water needs [ 19 – 24 ]. Water from such sources is often consumed without any form of treatment [ 12 , 19 , 21 ]. However, these alternative sources of drinking water are often vulnerable to point and non-point sources of pollution and are contaminated frequently by fecal matter [ 5 , 19 , 25 ]. A report by the South African Council for Scientific and Industrial Research clearly showed that almost 2.11 million people in South Africa lack access to any safe water infrastructure. The consumption of water from such unimproved sources without treatment constitutes a major public health risk [ 26 ].
Consumption of contaminated drinking water is a cause of diarrheal disease, a leading cause of child mortality in developing countries with about 700,000 deaths of children under the age of 5 reported in 2011 [ 10 , 27 ]. In South Africa, diarrhea is one of the leading causes of death among young children, and this problem is worst in children infected with HIV (Human Immunodeficiency Virus).
The health risks associated with the consumption of unsafe drinking water are not only related to infectious diseases but also to other environmental components such as fluoride, arsenic, lead, cadmium, nitrates and mercury. Excessive consumption of these substances from contaminated drinking water can lead to cancer, dental and skeletal fluorosis, acute nausea, memory lapses, renal failure, anemia, stunted growth, fetal abnormalities and skin rashes [ 16 , 28 ]. Groundwater contamination with high arsenic concentrations have been reported in Bangladesh, and high fluoride concentrations have been reported in the drinking water from various provinces in South Africa [ 28 – 34 ].
Temporary seasonal variations have been reported to influence the levels of contaminants in various water sources differently. The key environmental drivers across the wet and dry seasons include: volume of water, flow, frequency of rainfall events, storm run-off, evaporation and point sources of pollution [ 35 , 36 ]. An increase in storm-water run-off within a river catchment may increase the level of contaminants due to land-use activities. Increased water volume could lead to a decrease in the concentration of contaminants due to the dilution effect. A low incidence of rainfall and high evaporation can cause a contaminant to concentrate in water. Very few water-quality parameters such as turbidity are expected to be higher in the wet season. Other parameters can vary depending on the key environmental drivers. There is paucity of data on the effect of change across seasons on water-use practices among household in rural areas of developing countries.
The geographic area for this study is located 35 km north of Thohoyandou, in Limpopo Province, South Africa. The area is primarily agricultural, such that water contamination by nitrates is a potential concern. In addition, mining operations in the area may contaminate water sources with heavy metals.
The significance of this study lies in the broad characterization of water-quality parameters that could affect human health, which is not restricted to microbiological analysis. In a rural community, the primary concern of drinking water is the microbiological quality of the water and chemical constituents are often considered not as problematic. This study was designed to evaluate a broad spectrum of water-quality constituents of natural water sources and household drinking water used by residents of rural communities in Limpopo Province. We also aimed to determine how water sources and collection practices change between dry and wet seasons within a one-year sampling period.
2. Materials and Methods
2.1. study design.
A baseline census of 10 villages in the Thulamela Municipality of Limpopo Province was completed to identify all households in which there was at least one healthy child under 3 years of age in the household, the child’s caregiver was at least 16 years of age, and the household did not have a permanent, engineered water-treatment system. 415 households that met these eligibility criteria were enrolled for the purposes of a water-treatment intervention trial. The baseline assessment of water-quality and use practices is reported here. Caregivers of the child under 3 years of age were given a questionnaire concerning demographics, socioeconomic status, water-use practices, sanitation and hygiene practices, and perceptions of water quality and health. In addition, a sample of drinking water was taken from a random selection of 25% of the total enrolled households in the dry (June–August 2016) and wet seasons (January–February 2017). The participant population was sorted by community, as a surrogate for water supply, and one-third from each community was randomly selected by a random number generated within Microsoft Excel (Seattle, WA, USA), which was sampled. The protocol used was approved by the Research Ethics Committee at the University of Venda (SMNS/15/MBY/27/0502) and the Institutional Review Board for Health Sciences Research at the University of Virginia (IRB-HSR #18662). Written informed consent was obtained from all participants and consent documentation was made available in English and Tshivenda. The majority of the baseline surveys were conducted in the dry season (approximately April to October). Six-months later, follow-on surveys were conducted at the height of the wet season (approximately November to March; however, the height of the season in 2016–17 was January to March).
2.2. Regional Description of the Study Area
The communities are located in a valley in the Vhembe District of Limpopo Province, South Africa ( Figure 1 ). The valley surrounds the Mutale River in the Soutpansberg Mountains and is located around 22°47′34′′ S and 30°27′01′′ E, in a tropical environment that exhibits a unimodal dry/wet seasonality ( Figure 2 ). In recent years, the area has received annual precipitation between 400 mm and 1100 mm; more importantly, the timing of the precipitation is highly variable ( Figure 2 ). Specifically, in 2010, the annual precipitation was about 750 mm; however, the majority of the precipitation came in March while, traditionally, the wet season begins earlier, in September or October. The year 2011 had the highest precipitation in the six-year period and had the majority of the rainfall in November. The years 2012 and 2015 began with a typical precipitation pattern; however, the rainfall did not continue as it did in 2013 and 2014. Annual temperature of the area also varies, with the highest temperature always recorded in the wet season ( Figure 3 ). There has been much variability of temperature in past years; however, this is beyond the scope of this study. The abbreviations used in Figure 1 and other figures, including the supplementary data and the type of the various water sources used in this study, are shown in Table 1 .

Map of the study area. The communities are all located within the Mutale River watershed. The rivers are indicated in blue, villages outlined in purple, environmental samples in blue squares, tributaries in green circles (which have intermittent flow), watershed boundary in orange. This heavily agricultural area has cultivated areas along both sides of thee Mutale River for the vast majority of the region; the area is shown with green outlines. There are two identified brick-processing areas shown in brown rectangles. Unfortunately, some sites are so close that the markers overlap (as with CR and IR). The location of the community supplies (CA, CB, and CC) are not shown to protect the privacy of those villages. See supplemental information for Google Earth files.

Precipitation trends in the study area. ( a ) Annual precipitation by hydrologic year. Data quality are presented on a scale of zero to unity where the quantity shown represents the proportion of missing or unreliable data in a year; ( b ) Cumulative precipitation for the last five complete years; ( c ) Average monthly precipitation calculated for years with greater than 90% reliable data (bottom right). All data are presented by the standard Southern hemisphere hydrologic year from July to June numbered with the ending year. Data are from the Nwanedzi Natural Reserve at the Luphephe Dam (17 km from the study area) and fire available through the Republic of South Africa, Department of Water and Sanitation, Hydrologic Services ( http://www.dwa.gov.za/Hydrology/ ).

The mean monthly temperature in the region recorded at Punda Milia. ( a ) Mean monthly temperature based on the means from 1962–1984; ( b ) Mean monthly temperature record. Data are available from the National Oceanic and Aviation Administration (U.S.), National Climatic Data Center, Climate Data Online service ( https://www.ncdc.noaa.gov/cdo-web/ ).
Abbreviations, water sources and type.
Source Name | Abbreviation | Type |
---|---|---|
Municipal Tap (Branch 2) | MT | treated |
Mutale River-downstream | MD | surface |
Mutale River-upstream | MU | surface |
Tshiombedi River | TB | surface |
Madade River | MA | surface |
Pfaleni River | PF | surface |
Tshala River | TH | surface |
Irrigation Canal (Tshala River diversion) | IR | diversion (from surface) |
Community A System | CA | diversion (from surface) |
Community B System | CB | diversion (from surface) |
Community C System | CC | diversion (from surface) |
Tshibvumo/Mbahela Spring | TS | shallow groundwater |
Mbulugeni’s Orchard Spring | OS | shallow groundwater |
Lutsingeni Spring | LS | shallow groundwater |
Clinic A Borehole | CR | groundwater |
Clinic B Borehole | CT | groundwater |
Madadani Borehole | MB | groundwater |
Pile Spring | PS | shallow groundwater |
Agriculture occupies tine greatest land cover in the valley. Mogt households are engaged in some level of farming. Crops cultivated include maize and vegetables, and tree fruits include mangos and citrus fruits. Livestock is prevalent in the area with chickens, goats, and cattle. Smaller animals typically remain closer to households and larger animals graze throughout the region without boundaries. There are several brick-making facilities in the valley that include excavation, brick-forming and drying.
2.3. Water Sources
Drinking water in the study communities is available from a number of municipal and natural sources. The primary source of drinking water for seven of the villages is treated, municipal water. Two of the villages have community-level boreholes, storage tanks, and distribution tanks. An additional village has a borehole as well; however, residents report that, since its installation, the system has never supplied water.
The water for the treatment facility is drawn from behind a weir in the Mutale River and pumped to a retention basin. The water then undergoes standard treatment that includes pH adjustment, flocculation, settling, filtration, and chlorine disinfection. Water is then pumped to two elevated tanks that supply several adjacent regions, including the study area. Specifically, Branch 1 supplies Tshandama, Pile, Mutodani, Tshapasha and Tshibvumo; Branch 2 supplies an intermediary tank that in turn serves Matshavhawe, Muledane and Thongwe. Households can pay for a metered yard connection for the water used; these yard connections can be connected to household plumbing at the household’s discretion. The treated municipal water service is intermittent. Service in Tshandama and Pile was observed to be constant during the wet season and for only about two to three days per week during the dry season. Service in the remaining communities is two to four days per week during the wet season and about two days per week during the dry season. Furthermore, for the past two years, major repairs in the dry season caused the treated municipal water to cease completely. Households typically stored water for the periods when the treated municipal water was off; however, when the municipal water was unavailable for longer periods or not on the anticipated schedule, households obtained water from natural sources. The community-level boreholes provided water almost constantly but were subject to failure and delays in repairs.
Aside from the municipal sources, many residents of three villages have access to a community installed and operated distribution system that delivers water from the adjacent ephemeral rivers throughout the community (CA, CB, and CC). These systems are constructed with 50 mm to 70 mm (5 to 7 × 10 −2 m) high-density polyethylene pipes. Even these community-level schemes provide water on a schedule and sometimes require repair. Another common source of water for the community is springs. These shallow groundwater sources are common in the valley; however, there are communities that do not have a nearby spring. Some springs have had a pipe placed at the outlet to keep the spring open and facilitate filling containers. Researchers did not observe any constructions around the springs to properly isolate them from further contamination, and they are, therefore, not improved water sources. Pit latrines are common in every household throughout the region. Source (TS) is located near these communities while other springs (OS, LS) are located in agricultural areas. Boreholes provide deep groundwater supplies but require a pump. Such systems provide water as long as there is power for the pump and the well is deep enough to withstand seasonal variations. The two clinics in the study area surveyed each relied on a borehole for their water supply. Some residents also collected water directly from the river. The Mutale River is a perennial river; however, the ephemeral rivers, the Tshiombedi, Madade, Pfaleni, and Tshala Rivers, do not flow in the dry season all the way to the floor of the valley. The Tshala River has a diversion to a lined irrigation canal that always carries water, but there is very little flow that remains in the natural channel.
2.4. Water Sampling
The team of community health workers (CHW) that had previously conducted the MAL-ED (Malnutrition and Enteric Diseases) study in the same region [ 37 ] were recruited to assist with the data collection for this study; specifically, the regional description and water sources. These CHWs have an intimate knowledge of the communities as they are residents and have conducted health research in the area. The CHWs provided information on the location and condition of the various water sources in the study communities.
Water sources were tested during two intensive study periods: one in the dry season (June–August, 2016) and the other in the wet season (January–February, 2017). Water sources for investigation were selected based on identification from resident community health workers. Single samples were taken from all 28 identified drinking water sources in the 10 villages and three days of repeated samples were taken from six sources, which represented a range of sources (e.g., surface, borehole, shallow ground, pond, and municipal treated) in the dry season. Single samples of 17 of the original sources and three days of repeated samples were taken from five sources in the wet season, six months later. Some sources were not resampled because the routes to the sources were flooded, and these sources were likely infrequently used during the wet season due to blocked pathways. The wet and dry season measurements gave two different scenarios for water-use behaviors and allowed the researchers to measure representative water-quality parameters.
2.5. Measurement of Physicochemical Parameters
Physicochemical parameters of source water samples were measured in the field by a YSI Professional Plus meter (YSI Inc., Yellow Springs, OH, USA) for pH, dissolved oxygen and conductivity. The probes and meter was calibrated according to the manufacturer’s instructions. Turbidity was measured in the field with an Orbeco-Hellige portable turbidimeter (Orbeco Hellige, Sarasota, FL, USA) (U.S. Environmental Protection Agency method 180.1) [ 38 ]. The turbidimeter was calibrated according to the manufacturer’s instructions. Measured levels were compared to the South African water-quality standards in the regulations [ 39 ], pursuant to the Water Services Act of 1997.
2.6. Microbiological Water-Quality Analysis
Escherichia coli ( E. coli ) and total coliform bacteria were measured in both source and household water samples by membrane filtration according to U.S. Environmental Protection Agency method 10,029 [ 40 ]. Sample cups of the manifold were immersed in a hot-water bath at 100 °C for 15 min. Reverse osmosis water was flushed through the apparatus to cool the sample cups. Paper filter disks of 47 mm (4.7 × 10 −2 m) diameter and 0.45 μm (4.5 × 10 −7 m) pore size (EMD Millipore, Billerica, MA, USA) were removed from their sterile, individual packages and transferred to the surface of the manifold with forceps with an aseptic technique. Blank tests were run with reverse osmosis dilution water. Two dilutions were tested: full-strength (100 mL sample) and 10 −2 (1 mL sample with 99 mL of sterile dilution water) were passed through the filters; this provides a range of zero to 30,000 CFU/100 mL (colony forming units) for both E. coli and total coliforms. The filter paper was placed in a sterile petri dish with absorbent pad with 2 mL (2 × 10 −6 m 3 ) of selective growth media solution (m-ColiBlue24, EMD Millipore, Billerica, MA, USA). The samples were incubated at 35 °C (308.15 K) for 23–25 h. Colonies were counted on the full-strength sample. If colonies exceeded 300 (the maximum valid count), the dilution count was used. In all tests, the dilution value was expected to be within 10 −2 of the full-strength value and the sample was discarded otherwise.
The distribution of the household bacteria levels was evaluated by the (chi square) χ 2 goodness-of-fit test for various subsets of the data. Subsets of the data were then compared by an unpaired Student’s t-test for statistical significance; specifically, wet versus dry season levels as well as any other subsets that could demonstrate differences within the data.
2.7. Major Metals Analysis
A Thermo ICap 6200 Inductively Coupled Plasma Atomic Emission Spectrometer (ICP-AES, Chemetix Pty Ltd., Johannesburg, South Africa) was used to analyze the major metals in the various samples. The National Institute of Standards and Technology traceable standards (NIST, Gaithersburg, MD, USA) purchased from Inorganic Ventures (INORGANIC VENTURES 300 Technology Drive Christiansburg, Christiansburg, VA, USA) were used to calibrate the instrument for the quantification of selected metals. A NIST-traceable quality control standard from De Bruyn Spectroscopic Solutions, Bryanston, South Africa, were analyzed to verify the accuracy of the calibration before sample analysis, as well as throughout the analysis to monitor drift.
2.8. Trace Metals Analysis
Trace elements were analyzed in source water samples using an Agilent 7900 Quadrupole inductively coupled plasma mass spectrometer (ICP-MS) (Chemetix Pty Ltd., Johannesburg, South Africa). Samples were introduced via a 0.4 mL/min (7 × 10 −9 m 3 s −1 ) micro-mist nebulizer into a Peltier-cooled spray chamber at a temperature of 2 °C (275.15 K), with a carrier gas flow of 1.05 L/min (1.75 × 10 −5 m 3 s −1 ). The elements V, Cr, Mn, Fe, Co, Ni, Cu, Zn, As, Se were analyzed under He-collision mode to remove polyatomic interferences. NIST-traceable standards was used to calibrate the instrument. A NIST-traceable quality control standard of a separate supplier to the main calibration standards was analyzed to verify the accuracy of the calibration before sample analysis.
2.9. Anion Analysis
The anions were analyzed in source-water samples as stated in Edokpayi et al. [ 41 ]. Briefly, an Ion Chromatograph (Metrohm, Johannesburg, South Africa) was used to analyze the concentrations of fluoride, bromide, nitrates, chloride and sulfate. Calibration standards in the range of 1–20 mg/L were prepared from 100 mg/L stock solution containing all the test elements. Prior to analysis, the samples were filtered with a 0.45 μm (4.5 × 10 −7 m) syringe filter. Eluent for the sample run was prepared from sodium bicarbonate and sodium carbonate. A 50 mmol/L sulphuric acid with a flow rate of 0.5 mL/min (8 × 10 −9 m 3 s −1 ) was used as suppressant.
3.1. Socio-Demographic Characteristics of Enrolled Households
We included 405 enrolled households who completed the baseline questionnaire. The majority of caregivers were the mothers (n = 342, 84.4%, median age = 27 years) or grandmothers (n = 51, 12.6%, median age = 50 years) of a young child in the household. Almost all the caregivers had completed at least secondary school education (n = 371, 91.6%). Median monthly income for the entire household was USD$106 (interquartile range (IQR): 71–156). Access to improved sanitation was high. 373 (n = 92.1%) households used an improved pit latrine, and only 19 (n = 4.7%) reported open defecation. However, few households (n = 35,8.6%) reported having a designated place to wash hands near their toilet, and only 29% (n = 119) reported always using soap when washing hands.
Most households had their primary water source ( Table 2 ) piped into their or their neighbor’s yard (dry: n = 226, 62.3%; wet: n = 241, 67.5%) or used a public tap (dry: n = 69, 19.0%; wet: n = 74, 20.7%). A minority (dry: n = 40, 11.0%; wet: n = 19, 5.3%) collected their water directly from rivers, lined canals, or springs. Water was collected by adult women in most households, and it was reported to take a median of 10 min (IQR, both seasons: 5–30) to go to their water source, collect water, and come back in one trip. Three quarters (n = 270, 74.4%) reported that their water source was not continually available in the dry season and two-thirds (n = 234, 65.5%) in the wet season. Almost half (48.9%) reported interruptions in availability that lasted at least 7 days in the dry season and 32.8% in the wet season. Households stored water during interruptions and/or collected water from alternative sources (dry: n = 133, 36.6%; wet: n = 115, 32.2%), which were surface water or shallow groundwater sources (e.g., rivers, lined canals, or springs).
Primary drinking-water sources reported among 363 and 357 households in the study area in the dry and wet seasons, respectively.
Main Drinking-Water Source | Number (%) | |
---|---|---|
Dry Season | Wet Season | |
n = 363 | n = 357 | |
Piped into house | 13 (3.6) | 15 (4.2) |
Piped into yard | 189 (52.1) | 218 (61.1) |
Neighbor’s pipe | 37 (10.2) | 23 (6.4) |
Public tap | 69 (19.0) | 74 (20.7) |
Natural source | 40 (11.0) | 19 (5.3) |
Tanker truck | 0 (0.0) | 0 (0.0) |
Other | 15 (4.1) | 8 (2.2) |
Household water was most frequently stored in jerry cans or plastic buckets (n = 363, 89.7%), while 25 households stored water in large drums or plastic tanks (6.2%). Most households reported that their drinking water containers were covered (n = 329, 81.2%), but most used a cup with a handle (n = 281, 69.4%) or their hands (n = 93, 23.0%) for water collection ( Table 3 ). Only 13.3% (n = 54) households reported treating their water, mainly by boiling (n = 22), chlorine (n = 15), or letting the water stand and settle (n = 11).
Mode of water collection from storage containers.
Mode of Water Collection | n (%) |
---|---|
Pour directly | 21 (5.2) |
Use cup with handle | 281 (69.4) |
Use cup with hands | 93 (23.0) |
Spigot | 4 (1.0) |
Other | 6 (1.5) |
Approximately one-third of caregivers (n = 114, 28.2%) perceived that one can get sick from drinking water (n = 114, 28.2%), and cited diarrhea, schistosomiasis, cholera, fever, vomiting, ear infections, malnutrition, rash, flu and malaria as specific illnesses associated with water. Despite these perceptions, the majority were satisfied with their current water source (n = 297, 73.3%). Those who were unsatisfied cited reasons of insufficient quantity (n = 75), shared water supply (n = 65), uncleanliness (n = 73), cloudiness (n = 47), and bad odor or taste (n = 38).
3.2. Physicochemical and Microbiological Characteristics of the Water Sources
pH and conductivity values ranged between 5.5–7.3 and 24–405 μS/cm in the wet season and 5.8–8.7 and 8–402 μS/cm in the dry season ( Table S1 ). Both pH and conductivity levels were within the recommended limits of the World Health Organization (WHO) for drinking water. The microbiological results and turbidity of the sources tested are presented in Figures Figures4 4 and and5, 5 , and Table S2 , respectively. Microbiological data show contamination with E. coli , a fecal coliform that is potentially pathogenic, and other coliform bacteria.

Membrane filtration results for ( a ) E. coli and ( b ) other coliforms. Data are presented for wet and dry seasons. The four ephemeral rivers (*) have no dry season data because they had no flow; all other sources have the results reported, some of which are zero or near-zero. South African National Standard (SANS 241:1-2015) set the limit of 0 CFU/100 mL for E. coli and 10 CFU/100 mL for total coliforms (CFU/10 −4 m 3 ). Ephemeral rivers that do not flow all the way into the valley are indicated (*) in the dry season.

Turbidity of the water sources in the study area. Two to three measurements were taken during an intensive study period from 13 January 2017 to 4 February 2017 in the wet season and three to four measurements from 5 June 2016 to 15 July 2016 in the dry season. The median measurement of the values is reported here. Ephemeral rivers that do not flow all the way into the valley are indicated (*) in the dry season.
Municipal treated water never showed any detectable colony-forming units (CFU) in a 100 mL sample for E. coli , which is within the Soufh African regulation [ 39 ]. In the wet season, other coliform bacteriaweae detected in the treated wtter (a median valueof 10 CFU/100 mL wac recorded).
Household sample of stored water ( Figure 6 ) show that bacterial contamination levels ranged from no detectable colonies lo the maximum detection level of our protocol of 30,000 CFU/100 mL. There is a trend that total colitorm levels ere lower (during the wet season than the dry season. In the wet season, some communities within the sturdy area had access to constant municipal treated water as monitored by researcher verification of public tap-watcr availebJlity. Othet communities had intermittent access to municipal treated water. Of these honseholds, those that had constant access to treated water at or near their household did have less total coliform in their stored water than those with intermittent services ( Figure 7 ). This neglects the communities that are outside of the municipal treated-water servic e area.

Box-and-whisker plot of total coliform measurements of stored, untreated water in study households in the wet (n = 95) and dry (n = 103) seasons. The box-and-whisker plot indicates the mean (diamond), first, second, and third quartiles (box), and minimum and maximum (whiskers).

Box-and-whisker plot of total coliform measurements of stored water in the wet season in study households in communities that had verified continuous access to municipal treated water versus verified intermittent access.
The total coliform from households in communities with verified continuous treated water had a log-normal distribution (verified by 99%, α = 1 significance level, χ 2 goodness-of-fit test) and were statistically significantly lower (α =1 significance level) than those from households in communities with verified intermittent treated water. Unfortunately, due to the low number of samples from intermittent households, a χ 2 goodness-of-fit test was not meaningful.
3.3. Anion Concentrations
Major anions investigated in the various water sources fell within the recommended guideline values from the WHO [ 42 ]. Fluoride concentrations ranged from below the detection limit (bdl) to 0.82 mg/L in the dry season and to 1.48 mg/L ( Table S3 ) in the wet season. Fluoride levels fell below the threshold limit for fluoride in drinking water from the WHO (1.5 mg/L). Nitrates were also observed within the limit of drinking water, between bdl–17.48 mg/L and bdl–9.72 mg/L in the dry and wet seasons, respectively. Chloride, sulfate and phosphate levels were also present in moderate levels in the various water sources; however, a relatively high concentration of chloride of 462.9 mg/L was determined in the Mutale River in the wet season.
3.4. Trace and Major Elements Composition
Major metals in the various water sources in both seasons complied with the recommended limits of SANS and WHO in drinking water [ 39 , 42 ]. Sodium concentrations in the range of 3.14–41.03 mg/L and 3.02–15.34 mg/L were measured in the wet and the dry seasons, respectively ( Table S4 ). Low values of potassium were measured. Calcium levels ranged between 0.66–33.91 mg/L and 0.53–27.39 mg/L, in the wet and dry seasons, respectively. Low levels of magnesium were also found. Most of the water sources can be classified as soft water owing to the low levels of calcium and magnesium. Aluminium (Al) concentration ranged between 39.18–438 μg/L ( Figure 8 ). Two of the water sources which are community-based water supply systems recorded high levels of Al which exceeded the aesthetic permissible levels of drinking water; others fell within this limit. Similarly, the levels of iron (Fe) varied between 37.30–1354 mg/L and 35.21–1262 mg/L in the wet and the dry seasons, respectively ( Figure 9 ). Some of the sources showed high Fe concentration which exceeded the aesthetic permissible limit of WHO in drinking water [ 42 ]. Two community-based water systems had higher levels of Fe in the wet season as well as the major river in the region (Mutale River) for which high Fe levels were observed in both seasons. One of the clinic boreholes also recorded high levels of Fe above the permissible aesthetic value of (300 mg/L) in both seasons. Temporary seasonal variation was significant only in the levels of Fe and Al. In the wet season, their levels were generally higher than in the dry season. Some other trace metals of concern like Pb, Hg, As, Cd, Cr, Ni, Cu, Mn, Sr were all present at low levels that were below their recommended limits in drinking water for both seasons ( Table S5 ).

Aluminum, measured by an inductively coupled plasma mass spectrometer (ICP-MS), concentration for natural sources in the study area in the wet and dry seasons. The SANS 241 standard is shown (an operational standard is intended for treated water). Sources marked with * are intermittent sources and had no dry-season sample. Other sources have measured concentrations; although they may be too low to plot.

Iron, measured by an ICP-MS, concentration for natural sources in the study area in the wet and dry seasons. The SANS 241 standard is shown. Sources marked with * are intermittent sources and had no dry-season sample. Other sources have measured concentrations; although they may be too low to plot.
4. Discussion
This study provides a comprehensive description of water quality and drinking-water use across seasons in a low-resource community in rural South Africa, including a variety of water sources, ranging from the municipal tap to natural sources and a combination of both when the municipal tap was intermittently available.
Water sources in the study area, aside from the municipal tap, were highly contaminated with E. coli in both the wet and dry seasons; that is, E. coli was above the South African standard (acute health) of 0 CFU/100 mL. It is particularly important to note that E. coli was detected in the boreholes used for water at the local clinics, implying inadequate access to potable water for potentially immunocompromised patients. While the municipal treated water met the E. coli detection limit, the municipal tap did not always fall within the standards of turbidity (≤1 NTU operational and ≤5 NTU aesthetic) and total coliform (≤10 CFU/100 mL) [ 39 ]. These are not direct health risks; however, both measurements can be used to judge the efficacy of the treatment process and suggest that treatment may not have removed other pathogens that were not directly tested, such as protozoan parasites.
While the microbiological contamination of the drinking-water sources was not acceptable, the chemical constituents fell within the South African guidelines [ 39 ]. Calcium, sodium, magnesium and potassium were present in low levels and their concentrations complied with regulatory standards of SANS [ 39 ] and WHO [ 42 ]. Some metals (cadmium, mercury, arsenic and lead) known to be carcinogenic, mutagenic and teratogenic, causing various acute and chronic diseases to humans even at trace levels in drinking water, were investigated and found to be present in very low concentrations that could be of no health risk to the consumers of the various water resources in the region. However, some other metals, such as Al and Fe, were higher in some of the water sources; yet these were still well below the health guidelines for the respective constituent (recommended health levels from SANS and WHO are given as Al < 0.9 mg/L, Fe < 2 mg/L). At these levels, they do not present a health risk but could impart color and significant taste to the water thereby affecting its aesthetic value. Water sources from the community water-supply systems and one of the clinic boreholes recorded higher levels of Al and Fe. The other metals evaluated (copper, zinc, nickel, chromium, Se and Mn) were present in low levels that complied with their recommended limits in drinking water [ 39 , 42 ].
Fluoridation of drinking water is a common practice for oral health in many countries [ 43 ]. The required level of fluoride to reduce incidences of dental caries is in the range of 0.6–0.8 mg/L; however, levels above 1.5 mg/L are associated with dental and skeletal fluorosis [ 43 – 45 ]. The likelihood of fluorosis as a result of high concentration of fluoride is low in these communities, but there could be a high incidence of dental caries since fluoride levels below 0.6 mg/L were measured and some of the water sources did not have fluoride concentrations detectable by the instrument. The National Children’s Health Survey conducted in South Africa showed that 60.3% of children in the age group of 6 years have dental caries. Approximately a third (31.3%) of children aged 4–5 years in Limpopo province have reported cases of dental caries [ 44 , 45 ].
Chloride levels in the water sources do not cause any significant risk to the users except imparting taste to the water for some of the sources that recorded chloride levels above 300 mg/L. Although the study area is characterized by farming activities, the nitrate concentrations measured do not present any health risks. Therefore, the occurrence of methemoglobinemia or blue-baby syndrome as a result of high nitrate levels is unlikely. Other anions were present in moderate levels that would also not constitute any health risks. The levels of all the anions determined in the various sources were lower than the recommended guidelines of WHO [ 42 ].
The microbiological analysis of environmental water sources revealed several trends. Without exception in these samples, bacterial levels in the wet season were higher than in the dry season. This may be caused by greater runoff or infiltration, which carries bacteria from contaminated sources to these water bodies. The upward trend in bacteria in the municipal treated water is not explained by an increase in runoff, but may be due to higher turbidity of the intake for the municipal treated water in the wet season. The treatment facility workers reported to the researchers that they were unable to monitor the quality of the treated water due to instrument failure during the wet season surveillance period.
Water stored in the household showed that the mean total coliform in the wet season was lower than that in the dry season. This trend is opposite to what was observed in the source, or environmental samples. This difference may be explained by the greater availability of treated water in the wet season versus the dry season for approximately 40% of the sampled households ( Figure 7 ). In addition, it is possible that families try to save water during the dry season and do not reject residual water, while the rainy season allows easier washing of the container and for it to be filled with fresh water more regularly.
In the wet season, two communities had consistently treated water available from household connections (usually a tap somewhere in a fence-in yard) or public taps. While the municipal treated water was of lower quality in the wet season than the dry season, the quality was significantly better than most environmental sources.
Another potential explanation is that residents stored their water within their households for a shorter time, which is supported by the use data that showed interruptions in supply were more common and for longer duration in the dry season. The quality of the water stored in households with continuous supply versus intermittent supply also suggests that water availability may play a role in household water quality. This is consistent with research that demonstrates that intermittent water supply introduces contamination into the distribution system in comparison with continuous supply [ 46 ]. Intermittent supply of water may also result in greater quantity and duration of storage at household level, which could increase the likelihood of contamination.
While it has been shown that the quality of water used for drinking in these villages does not meet South African standards, this problem is confounded by evidence from surveys indicating that residents believe they have high-quality water and, therefore, do not use any form of treatment. In the rare case that they do, it is by letting the water stand and settle or by boiling. In addition, even if treated water is collected, there is a risk of recontamination during storage and again when using a cup held by a hand to retrieve water from storage devices, which was common in surveyed homes. In addition, there was little to no detectable residual chlorine in the municipal tap water to prevent recontamination. A previous study performed in an adjacent community showed higher household treatment levels; however, this may have been due to intervention studies in that community (the community in question was excluded from this study because of previous interventions) [ 47 ]. The study also concurred that boiling was the most common method employed.
Given that most of the water from the various sources in this community is contaminated and not treated, there is a high risk of enteric disease in the community. Lack of access to adequate water and sanitation cause exposure to pathogens through water, excreta, toxins, and water-collection and storage pathways, resulting in immense health impacts on communities [ 48 ]. A large burden of death and disability due to lack of access to clean water and sanitation is specifically associated with diarrheal diseases, intestinal helminths, schistosomiasis and trachoma [ 49 ]. While it was found in this study that the study area has a high prevalence of improved sanitation, the likelihood of poor water quality due to intermittent supply and lack of treatment poses a risk of the adverse health effects described. In a previous longitudinal cohort study of children in these villages, most children were exclusively breastfed for only a month or less, and 50% of children had at least one enteropathogen detected in a non-diarrheal stool by three months of age [ 50 ]. Furthermore, the burden of diarrhea was 0.66 episodes per child-year in the first 2 years of life, and stunting prevalence (length-for-age z-score less than −2) in the cohort increased from 12.4% at birth to 35.7% at 24 months [ 50 ]. It is likely that contaminated water contributed to the observed pathogen burden and stunting prevalence in these communities. In summary, microbiological contamination of the drinking water is high in the study area, and risk from other chemical constituents is low. Therefore, engineered solutions should focus more on improving the microbiological quality of the drinking water.
The intermittent supply in municipal tap water, inadequate water quality from alternative sources, and the risk of recontamination during storage suggest a need for a low-cost, point-of-use water-treatment solution to be used at the household level in these communities. Access to clean drinking water will contribute to improving the health of young children who are at highest risk of the morbidity and mortality associated with waterborne diseases. Such an intervention may go beyond the prevention of diarrhea by impacting long-term outcomes such as environmental enteropathy, poor growth and cognitive impairment, which have been associated with long-term exposure to enteropathogens [ 51 ]. This is supported by a recent finding that access to improved water and sanitation was associated with improvements on a receptive vocabulary test at 1, 5 and 8 years of age among Peruvian, Ethiopian, Vietnamese and Indian children [ 52 ]. The implementation of point-of-use water treatment devices would ensure that water is safe to drink before consumption in the homes of these villages, improving child health and development.
5. Conclusions
This study was comprehensive in the assessment of all aspects of water quality and corresponding water-use practices in rural areas of Limpopo Province. The results obtained indicate that microbiological water quality is more likely to have adverse effect on the consumers of natural water without adequate treatment, as E. coli was determined in all the natural water sources. Local needs assessments are critical to understanding local variability in water quality and developing appropriate interventions. Interventions to ensure clean and safe drinking water in rural areas of Limpopo province should, first and foremost, consider microbiological contamination as a priority. Risk-assessment studies of the impact of water quality on human health is, therefore, recommended.
Supplementary Material
Tables s1 through s5.
Table S1: Physical characteristics of water sources. Two to three measurements were taken during an intensive study period from 13 January 2017 to 4 February 2017 in the wet season, and three to four measurements from 5 June 2016 to 15 July 2016 in the dry season. The median measurement of the values is reported here. Sites with missing samples, such as ephemeral rivers that do not flow all the way into the valley in the dry season, are indicated (*). Sites with missing data due to instrument failure are indicated (#). Values that were below the detection limit are indicated (bdl). South African regulation (SANS 241:1-2015) and the World Health Organization Recommended Guidelines for Drinking Water Quality (Fourth Edition) are listed; parameters not listed are indicated (nl),
Acknowledgments:
This project was funded by the Fogarty International Center (FIC) of the National Institutes of Health (NIH) (Award Number D43 TW009359), National Science Foundation (NSF) (Award Number CBET-1438619), the Center for Global Health at the University of Virginia (CGH), and the University of Virginia’s Jefferson Public Fellows (JPC) program. The content is solely the responsibility of the authors and does not represent the official views of the funders. The authors also acknowledge the tireless work of the community field workers who undertook interventions and collected all of the survey data. The authors also acknowledge A. Gaylord, N. Khuliso, S. Mammburu, K. McCain and E. Stinger, who performed much of the water-quality analysis and T. Singh, who supported the laboratory analysis for inorganic materials.
Supplementary Materials: The following are available online at www.mdpi.com/s1 ,
Conflicts of Interest: The authors declare no conflict of interest.

Maintenance work is planned from 22:00 BST on Monday 16th September 2024 to 22:00 BST on Tuesday 17th September 2024.
During this time the performance of our website may be affected - searches may run slowly, some pages may be temporarily unavailable, and you may be unable to access content. If this happens, please try refreshing your web browser or try waiting two to three minutes before trying again.
We apologise for any inconvenience this might cause and thank you for your patience.
Environmental Science: Water Research & Technology
Water quality in drinking water distribution systems: research trends through the 21st century.

* Corresponding authors
a ÉSAD, Université Laval, Quebec City, Canada E-mail: [email protected]
b WaterShed Monitoring, Quebec City, Canada
This paper provides new insight into the global landscape of water quality research in drinking water distribution systems and how it has evolved over the first twenty years of the 21st century. An up-to-date bibliometric analysis of relevant literature published between 2000 and 2020 revealed how the research landscape has expanded in terms of number of publications made, variety of topics, and geographic diversity that offers an increasingly inclusive global conversation. Results showed technological, microbial and chemical needs are currently the major research streams that are concentrated on popular topics of simulations, chlorine, biofilms, intrusion and monitoring. However, there is a vast diversity of sub-disciplines related to maintaining water quality, which are highly interconnected. These changing priorities and perspectives offer opportunities for sharing of best practice, identification of research gaps, and interdisciplinary thinking as we all strive to provide consumers with high quality drinking water now and into the future.
Article information
Download citation, permissions.

S. L. Weston, A. Scheili, S. Behmel and M. J. Rodriguez, Environ. Sci.: Water Res. Technol. , 2022, 8 , 3054 DOI: 10.1039/D2EW00491G
To request permission to reproduce material from this article, please go to the Copyright Clearance Center request page .
If you are an author contributing to an RSC publication, you do not need to request permission provided correct acknowledgement is given.
If you are the author of this article, you do not need to request permission to reproduce figures and diagrams provided correct acknowledgement is given. If you want to reproduce the whole article in a third-party publication (excluding your thesis/dissertation for which permission is not required) please go to the Copyright Clearance Center request page .
Read more about how to correctly acknowledge RSC content .
Social activity
Search articles by author, advertisements.
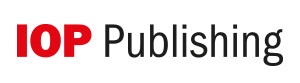
We apologize for the inconvenience...
To ensure we keep this website safe, please can you confirm you are a human by ticking the box below.
If you are unable to complete the above request please contact us using the below link, providing a screenshot of your experience.
https://ioppublishing.org/contacts/
Information
- Author Services
Initiatives
You are accessing a machine-readable page. In order to be human-readable, please install an RSS reader.
All articles published by MDPI are made immediately available worldwide under an open access license. No special permission is required to reuse all or part of the article published by MDPI, including figures and tables. For articles published under an open access Creative Common CC BY license, any part of the article may be reused without permission provided that the original article is clearly cited. For more information, please refer to https://www.mdpi.com/openaccess .
Feature papers represent the most advanced research with significant potential for high impact in the field. A Feature Paper should be a substantial original Article that involves several techniques or approaches, provides an outlook for future research directions and describes possible research applications.
Feature papers are submitted upon individual invitation or recommendation by the scientific editors and must receive positive feedback from the reviewers.
Editor’s Choice articles are based on recommendations by the scientific editors of MDPI journals from around the world. Editors select a small number of articles recently published in the journal that they believe will be particularly interesting to readers, or important in the respective research area. The aim is to provide a snapshot of some of the most exciting work published in the various research areas of the journal.
Original Submission Date Received: .
- Active Journals
- Find a Journal
- Proceedings Series
- For Authors
- For Reviewers
- For Editors
- For Librarians
- For Publishers
- For Societies
- For Conference Organizers
- Open Access Policy
- Institutional Open Access Program
- Special Issues Guidelines
- Editorial Process
- Research and Publication Ethics
- Article Processing Charges
- Testimonials
- Preprints.org
- SciProfiles
- Encyclopedia
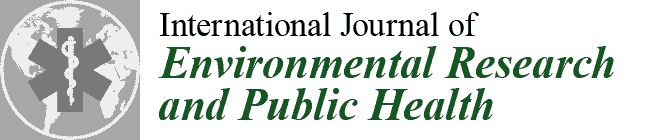
Journal Menu
- IJERPH Home
- Aims & Scope
- Editorial Board
- Reviewer Board
- Topical Advisory Panel
- Instructions for Authors
- Special Issues
- Sections & Collections
- Article Processing Charge
- Indexing & Archiving
- Editor’s Choice Articles
- Most Cited & Viewed
- Journal Statistics
- Journal History
- Journal Awards
- Society Collaborations
- Conferences
- Editorial Office
- 20th Anniversary
Journal Browser
- arrow_forward_ios Forthcoming issue arrow_forward_ios Current issue
- Vol. 21 (2024)
- Vol. 20 (2023)
- Vol. 19 (2022)
- Vol. 18 (2021)
- Vol. 17 (2020)
- Vol. 16 (2019)
- Vol. 15 (2018)
- Vol. 14 (2017)
- Vol. 13 (2016)
- Vol. 12 (2015)
- Vol. 11 (2014)
- Vol. 10 (2013)
- Vol. 9 (2012)
- Vol. 8 (2011)
- Vol. 7 (2010)
- Vol. 6 (2009)
- Vol. 5 (2008)
- Vol. 4 (2007)
- Vol. 3 (2006)
- Vol. 2 (2005)
- Vol. 1 (2004)
Find support for a specific problem in the support section of our website.
Please let us know what you think of our products and services.
Visit our dedicated information section to learn more about MDPI.
Drinking Water Quality and Human Health
- Print Special Issue Flyer
Special Issue Editors
Special issue information, benefits of publishing in a special issue.
- Published Papers
A special issue of International Journal of Environmental Research and Public Health (ISSN 1660-4601). This special issue belongs to the section " Environmental Health ".
Deadline for manuscript submissions: closed (30 June 2018) | Viewed by 172069
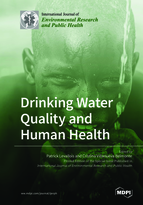
Share This Special Issue
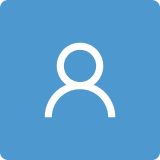
Dear Colleagues,
Quality of drinking water is paramount for public health. Despite important improvements in the last decades, access to safe drinking water is not universal. The World Health Organization estimates that almost 10% of the population in the world do not have access to improved drinking water sources. Among other diseases, waterborne infections cause diarrhoea, which kills nearly one million people every year, mostly children under 5 years of age. On the other hand, chemical pollution is a concern in high-income countries and an increasing problem in low- and middle-income countries. Exposure to chemicals in drinking water may lead to a range of chronic non-communicable diseases (e.g., cancer, cardiovascular disease), adverse reproductive outcomes and effects on children’s health (e.g., neurodevelopment), among other health effects. Although drinking water quality is regulated and monitored in many countries, increasing knowledge leads to the need for reviewing standards and guidelines on a nearly permanent basis, both for regulated and newly identified contaminants. Drinking water standards are mostly based on animal toxicity data, and more robust epidemiologic studies with accurate exposure assessment are needed. The current risk assessment paradigm dealing mostly with one-by-one chemicals dismisses potential synergisms or interactions from exposures to mixtures of contaminants, particularly at the low-exposure range. Thus, evidence is needed on exposure and health effects of mixtures of contaminants in drinking water. In addition, drinking water components and even quantity of water ingested may have some health benefits, which are currently under evaluated. Finally, water stress and water quality problems are expected to increase in the coming years due to climate change and increasing water demand by population growth, and new evidence is needed to design appropriate adaptation policies.
This special issue of International Journal of Environmental Research and Public Health (IJERPH) focuses on the current state of knowledge on the links between drinking water quality and human health. New research papers, reviews, case reports and conference papers are welcome to this issue. Papers dealing with new approaches to derive drinking water standards or risk assessment and management are also welcome. Other manuscript types accepted include methodological papers, position papers, brief reports, and commentaries.
We will accept manuscripts from different disciplines including exposure assessment science, epidemiology, intervention studies, risk and health impact assessment, risk management including drinking water standards. Here are some examples of topics that could be addressed in this Special Issue:
- Microorganisms and infectious diseases: virus, bacteria, parasites, etc.
- Natural contaminants: arsenic, barium, manganese, fluoride, radiation, etc.
- Anthropogenic chemicals: nitrate, pesticides, pharmaceutical and veterinary residues, personal care products, household products, antimicrobial resistance, organic compounds, etc.
- Algal blooms and microcystins.
- Contaminants due to treatment of drinking water: disinfection by-products, fluoride, by-products of flocculants or coagulants, etc.
- Contaminants from drinking water distribution: metals (e.g., lead, copper), and other compounds released by pipes (e.g., vinyl chloride).
- Bottled water quality.
- Mixtures of contaminants.
- Other water parameters, e.g., hardness, minerals.
- Interaction/balance between microorganisms and chemicals.
- Health benefits associated with drinking water.
- Climate change and drinking water quality.
Prof. Patrick Levallois Dr. Cristina Villanueva Belmonte Guest Editors
Manuscripts should be submitted online at www.mdpi.com by registering and logging in to this website . Once you are registered, click here to go to the submission form . Manuscripts can be submitted until the deadline. All submissions that pass pre-check are peer-reviewed. Accepted papers will be published continuously in the journal (as soon as accepted) and will be listed together on the special issue website. Research articles, review articles as well as short communications are invited. For planned papers, a title and short abstract (about 100 words) can be sent to the Editorial Office for announcement on this website.
Submitted manuscripts should not have been published previously, nor be under consideration for publication elsewhere (except conference proceedings papers). All manuscripts are thoroughly refereed through a single-blind peer-review process. A guide for authors and other relevant information for submission of manuscripts is available on the Instructions for Authors page. International Journal of Environmental Research and Public Health is an international peer-reviewed open access monthly journal published by MDPI.
Please visit the Instructions for Authors page before submitting a manuscript. The Article Processing Charge (APC) for publication in this open access journal is 2500 CHF (Swiss Francs). Submitted papers should be well formatted and use good English. Authors may use MDPI's English editing service prior to publication or during author revisions.
- Drinking water
- Microorganisms
- Infectious diseases
- Non-communicable diseases
- Epidemiology
- Health impact
- Drinking water standards
- Emerging contaminants
- Exposure assessment
- Multiroute exposure
- Risk assessment
- Risk management
- Risk communication
- Ease of navigation: Grouping papers by topic helps scholars navigate broad scope journals more efficiently.
- Greater discoverability: Special Issues support the reach and impact of scientific research. Articles in Special Issues are more discoverable and cited more frequently.
- Expansion of research network: Special Issues facilitate connections among authors, fostering scientific collaborations.
- External promotion: Articles in Special Issues are often promoted through the journal's social media, increasing their visibility.
- e-Book format: Special Issues with more than 10 articles can be published as dedicated e-books, ensuring wide and rapid dissemination.
Further information on MDPI's Special Issue polices can be found here .
Published Papers (21 papers)
Jump to: Research , Review
Jump to: Editorial , Review
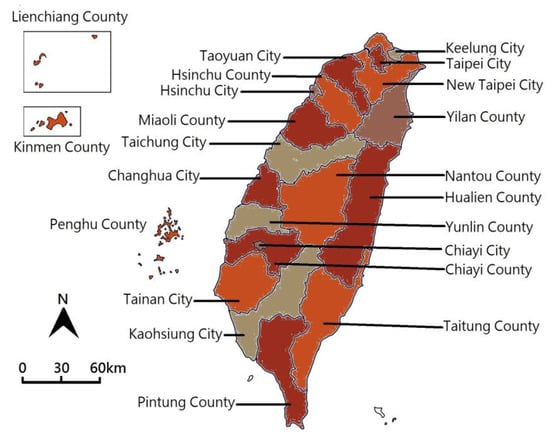
Jump to: Editorial , Research
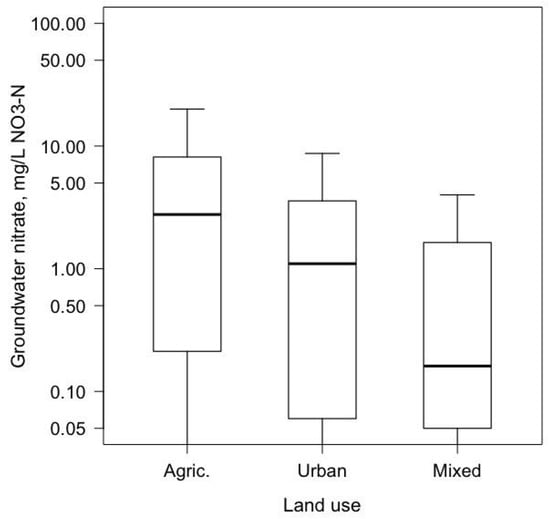
Further Information
Mdpi initiatives, follow mdpi.
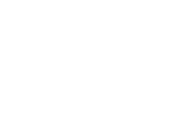
Subscribe to receive issue release notifications and newsletters from MDPI journals
- Open access
- Published: 21 January 2016

Drinking water quality assessment and its effects on residents health in Wondo genet campus, Ethiopia
- Yirdaw Meride 1 &
- Bamlaku Ayenew 1
Environmental Systems Research volume 5 , Article number: 1 ( 2016 ) Cite this article
116k Accesses
97 Citations
Metrics details
Water is a vital resource for human survival. Safe drinking water is a basic need for good health, and it is also a basic right of humans. The aim of this study was to analysis drinking water quality and its effect on communities residents of Wondo Genet.
The mean turbidity value obtained for Wondo Genet Campus is (0.98 NTU), and the average temperature was approximately 28.49 °C. The mean total dissolved solids concentration was found to be 118.19 mg/l, and EC value in Wondo Genet Campus was 192.14 μS/cm. The chloride mean value of this drinking water was 53.7 mg/l, and concentration of sulfate mean value was 0.33 mg/l. In the study areas magnesium ranges from 10.42–17.05 mg/l and the mean value of magnesium in water is 13.67 mg/l. The concentration of calcium ranges from 2.16–7.31 mg/l with an average value of 5.0 mg/l. In study areas, an average value of sodium was 31.23 mg/1and potassium is with an average value of 23.14 mg/1. Water samples collected from Wondo Genet Campus were analyzed for total coliform bacteria and ranged from 1 to 4/100 ml with an average value of 0.78 colony/100 ml.
On the basis of findings, it was concluded that drinking water of the study areas was that all physico–chemical parameters. All the Campus drinking water sampling sites were consistent with World Health Organization standard for drinking water (WHO).
Safe drinking water is a basic need for good health, and it is also a basic right of humans. Fresh water is already a limiting resource in many parts of the world. In the next century, it will become even more limiting due to increased population, urbanization, and climate change (Jackson et al. 2001 ).
Drinking water quality is a relative term that relates the composition of water with effects of natural processes and human activities. Deterioration of drinking water quality arises from introduction of chemical compounds into the water supply system through leaks and cross connection (Napacho and Manyele 2010 ).
Access to safe drinking water and sanitation is a global concern. However, developing countries, like Ethiopia, have suffered from a lack of access to safe drinking water from improved sources and to adequate sanitation services (WHO 2006 ). As a result, people are still dependent on unprotected water sources such as rivers, streams, springs and hand dug wells. Since these sources are open, they are highly susceptible to flood and birds, animals and human contamination (Messeret 2012 ).
The quality of water is affected by an increase in anthropogenic activities and any pollution either physical or chemical causes changes to the quality of the receiving water body (Aremu et al. 2011 ). Chemical contaminants occur in drinking water throughout the world which could possibly threaten human health. In addition, most sources are found near gullies where open field defecation is common and flood-washed wastes affect the quality of water (Messeret 2012 ).
The World Health Organization estimated that up to 80 % of all sicknesses and diseases in the world are caused by inadequate sanitation, polluted water or unavailability of water (WHO 1997 ). A review of 28 studies carried out by the World Bank gives the evidence that incidence of certain water borne, water washed, and water based and water sanitation associated diseases are related to the quality and quantity of water and sanitation available to users (Abebe 1986 ).
In Ethiopia over 60 % of the communicable diseases are due to poor environmental health conditions arising from unsafe and inadequate water supply and poor hygienic and sanitation practices (MOH 2011 ). About 80 % of the rural and 20 % of urban population have no access to safe water. Three-fourth of the health problems of children in the country are communicable diseases arising from the environment, specially water and sanitation. Forty-six percent of less than 5 years mortality is due to diarrhea in which water related diseases occupy a high proportion. The Ministry of Health, Ethiopia estimated 6000 children die each day from diarrhea and dehydration (MOH 2011 ).
There is no study that was conducted to prove the quality water in Wondo Genet Campus. Therefore, this study is conducted at Wondo Genet Campus to check drinking water quality and to suggest appropriate water treated mechanism.
Results and discussions
The turbidity of water depends on the quantity of solid matter present in the suspended state. It is a measure of light emitting properties of water and the test is used to indicate the quality of waste discharge with respect to colloidal matter. The mean turbidity value obtained for Wondo Genet Campus (0.98 NTU) is lower than the WHO recommended value of 5.00 NTU.
Temperature
The average temperature of water samples of the study area was 28.49 °C and in the range of 28–29 °C. Temperature in this study was found within permissible limit of WHO (30 °C). Ezeribe et al. ( 2012 ) reports similar result (29 °C) of well water in Nigeria.
Total dissolved solids (TDS)
Water has the ability to dissolve a wide range of inorganic and some organic minerals or salts such as potassium, calcium, sodium, bicarbonates, chlorides, magnesium, sulfates etc. These minerals produced un-wanted taste and diluted color in appearance of water. This is the important parameter for the use of water. The water with high TDS value indicates that water is highly mineralized. Desirable limit for TDS is 500 mg/l and maximum limit is 1000 mg/l which prescribed for drinking purpose. The concentration of TDS in present study was observed in the range of 114.7 and 121.2 mg/l. The mean total dissolved solids concentration in Wondo Genet campus was found to be 118.19 mg/l, and it is within the limit of WHO standards. Similar value was reported by Soylak et al. ( 2001 ), drinking water of turkey. High values of TDS in ground water are generally not harmful to human beings, but high concentration of these may affect persons who are suffering from kidney and heart diseases. Water containing high solid may cause laxative or constipation effects. According to Sasikaran et al. ( 2012 ).
Electrical conductivity (EC)
Pure water is not a good conductor of electric current rather’s a good insulator. Increase in ions concentration enhances the electrical conductivity of water. Generally, the amount of dissolved solids in water determines the electrical conductivity. Electrical conductivity (EC) actually measures the ionic process of a solution that enables it to transmit current. According to WHO standards, EC value should not exceeded 400 μS/cm. The current investigation indicated that EC value was 179.3–20 μS/cm with an average value of 192.14 μS/cm. Similar value was reported by Soylak et al. ( 2001 ) drinking water of turkey. These results clearly indicate that water in the study area was not considerably ionized and has the lower level of ionic concentration activity due to small dissolve solids (Table 1 ).
PH of water
PH is an important parameter in evaluating the acid–base balance of water. It is also the indicator of acidic or alkaline condition of water status. WHO has recommended maximum permissible limit of pH from 6.5 to 8.5. The current investigation ranges were 6.52–6.83 which are in the range of WHO standards. The overall result indicates that the Wondo Genet College water source is within the desirable and suitable range. Basically, the pH is determined by the amount of dissolved carbon dioxide (CO 2 ), which forms carbonic acid in water. Present investigation was similar with reports made by other researchers’ study (Edimeh et al. 2011 ; Aremu et al. 2011 ).
Chloride (Cl)
Chloride is mainly obtained from the dissolution of salts of hydrochloric acid as table salt (NaCl), NaCO 2 and added through industrial waste, sewage, sea water etc. Surface water bodies often have low concentration of chlorides as compare to ground water. It has key importance for metabolism activity in human body and other main physiological processes. High chloride concentration damages metallic pipes and structure, as well as harms growing plants. According to WHO standards, concentration of chloride should not exceed 250 mg/l. In the study areas, the chloride value ranges from 3–4.4 mg/l in Wondo Genet Campus, and the mean value of this drinking water was 3.7 mg/l. Similar value was reported by Soylak et al. ( 2001 ) drinking water of Turkey.
Sulfate mainly is derived from the dissolution of salts of sulfuric acid and abundantly found in almost all water bodies. High concentration of sulfate may be due to oxidation of pyrite and mine drainage etc. Sulfate concentration in natural water ranges from a few to a several 100 mg/liter, but no major negative impact of sulfate on human health is reported. The WHO has established 250 mg/l as the highest desirable limit of sulfate in drinking water. In study area, concentration of sulfate ranges from 0–3 mg/l in Wondo Genet Campus, and the mean value of SO 4 was 0.33 mg/l. The results exhibit that concentration of sulfate in Wondo Genet campus was lower than the standard limit and it may not be harmful for human health.
Magnesium (Mg)
Magnesium is the 8th most abundant element on earth crust and natural constituent of water. It is an essential for proper functioning of living organisms and found in minerals like dolomite, magnetite etc. Human body contains about 25 g of magnesium (60 % in bones and 40 % in muscles and tissues). According to WHO standards, the permissible range of magnesium in water should be 50 mg/l. In the study areas magnesium was ranges from 10.42 to 17.05 mg/l in Wondo Genet Campus and the mean value of magnesium in water is 13.67 mg/l. Similar value was reported by Soylak et al. ( 2001 ) drinking water of Turkey. The results exhibit that concentration of magnesium in Wondo Genet College was lower than the standard limit of WHO.
Calcium (Ca)
Calcium is 5th most abundant element on the earth crust and is very important for human cell physiology and bones. About 95 % of calcium in human body stored in bones and teeth. The high deficiency of calcium in humans may caused rickets, poor blood clotting, bones fracture etc. and the exceeding limit of calcium produced cardiovascular diseases. According to WHO ( 2011 ) standards, its permissible range in drinking water is 75 mg/l. In the study areas, results show that the concentration of calcium ranges from 2.16 to 7.31 mg/l in Wondo Genet campus with an average value of 5.08 mg/l.
Sodium (Na)
Sodium is a silver white metallic element and found in less quantity in water. Proper quantity of sodium in human body prevents many fatal diseases like kidney damages, hypertension, headache etc. In most of the countries, majority of water supply bears less than 20 mg/l, while in some countries the sodium quantity in water exceeded from 250 mg/l (WHO 1984 ). According to WHO standards, concentration of sodium in drinking water is 200 mg/1. In the study areas, the finding shows that sodium concentration ranges from 28.54 to 34.19 mg/1 at Wondo Genet campus with an average value of 31.23.
Potassium (k)
Potassium is silver white alkali which is highly reactive with water. Potassium is necessary for living organism functioning hence found in all human and animal tissues particularly in plants cells. The total potassium amount in human body lies between 110 and 140 g. It is vital for human body functions like heart protection, regulation of blood pressure, protein dissolution, muscle contraction, nerve stimulus etc. Potassium is deficient in rare but may led to depression, muscle weakness, heart rhythm disorder etc. According to WHO standards the permissible limit of potassium is 12 mg/1. Results show that the concentration of potassium in study areas ranges from 20.83 to 27.51 mg/1. Wondo Genet College with an average value of 23.14 mg/1. Present investigation was similar with reports made by other researchers’ study (Edimeh et al. 2011 ; Aremu et al. 2011 ). These results did not meet the WHO standards and may become diseases associated from potassium extreme surpassed.
Nitrate (NO 3 )
Nitrate one of the most important diseases causing parameters of water quality particularly blue baby syndrome in infants. The sources of nitrate are nitrogen cycle, industrial waste, nitrogenous fertilizers etc. The WHO allows maximum permissible limit of nitrate 5 mg/l in drinking water. In study areas, results more clear that the concentration of nitrate ranges from 1.42 to 4.97 mg/l in Wondo Genet campus with an average value of 2.67 mg/l. These results indicate that the quantity of nitrate in the study site is acceptable in Wondo Genet campus (Table 2 ).
Bacterial contamination
The total coliform group has been selected as the primary indicator bacteria for the presence of disease causing organisms in drinking water. It is a primary indicator of suitability of water for consumption. If large numbers of coliforms are found in water, there is a high probability that other pathogenic bacteria or organisms exist. The WHO and Ethiopian drinking water guidelines require the absence of total coliform in public drinking water supplies.
In this study, all sampling sites were not detected of faecal coliform bacteria. Figure 1 shows the mean values of total coliform bacteria in drinking water collected from the study area. All drinking water samples collected from Wondo Genet Campus were analyzed for total coliform bacteria and ranged from 1 to 4/100 ml with an average value of 0.78 colony/100 ml. In Wondo Genet College, the starting point of drinking water sources (Dam1), the second (Dam2) and Dam3 samples showed the presence of total coliform bacteria (Fig. 1 ). According to WHO ( 2011 ) risk associated in Wondo Genet campus drinking water is low risk (1–10 count/100 ml).
The mean values of total coliform bacteria in drinking water
According to the study all water sampling sites in Wondo Genet campus were meet world health organization standards and Ethiopia drinking water guideline. Figure 2 indicated that mean value of the study sites were under the limit of WHO standards.
Comparison of water quality parameters of drinking water of Wondo Genet campus with WHO and Ethiopia standards
Effect of water quality for residence health’s
Diseases related to contamination of drinking-water constitute a major burden on human health. Interventions to improve the quality of drinking-water provide significant benefits to health. Water is essential to sustain life, and a satisfactory (adequate, safe and accessible) supply must be available to all (Ayenew 2004 ).
Improving access to safe drinking-water can result in tangible benefits to health. Every effort should be made to achieve a drinking-water quality as safe as practicable. The great majority of evident water-related health problems are the result of microbial (bacteriological, viral, protozoan or other biological) contamination (Ayenew 2004 ).
Excessive amount of physical, chemical and biological parameters accumulated in drinking water sources, leads to affect human health. As discussed in the result, all Wondo Genet drinking water sources are under limit of WHO and Ethiopian guideline standards. Therefore, the present study was found the drinking water safe and no residence health impacts.
On the basis of findings, it was concluded that drinking water of the study areas was that all physico–chemical parameters in all the College drinking water sampling sites, and they were consistent with World Health Organization standard for drinking water (WHO). The samples were analyzed for intended water quality parameters following internationally recognized and well established analytical techniques.
It is evident that all the values of sodium (Na), potassium (K), calcium (Ca), magnesium (Mg), chloride (Cl), SO 4 , and NO 3 fall under the permissible limit and there were no toxicity problem. Water samples showed no extreme variations in the concentrations of cations and anions. In addition, bacteriological determination of water from College drinking water sources was carried out to be sure if the water was safe for drinking and other domestic application. The study revealed that all the College water sampling sites were not contained fecal coliforms except the three water sampling sites had total coliforms.
The study was conducted in Wondo Genet College of Forestry and Natural Resources campus, which is located in north eastern direction from the town of Hawassa and about 263 km south of Addis Ababa (Fig. 3 ). It lies between 38°37′ and 38°42′ East longitude and 7°02′ and 7°07′ north latitude. Landscape of the study area varies with an altitude ranging between 1600 and 2580 meters above sea level. Landscape of the study area varies with an altitude ranging between 1600 and 2580 meters above sea level.
Map of study area
The study area is categorized under Dega (cold) agro-ecological zone at the upper part and Woina Dega (temperate) agro-ecological zone at the lower part of the area. The rainfall distribution of the study area is bi-modal, where short rain falls during spring and the major rain comes in summer and stays for the first two months of the autumn season. The annual temperature and rainfall range from 17 to 19 °C and from 700 to 1400 mm, respectively (Wondo Genet office of Agriculture 2011).
Methodology
Water samples were taken at ten locations of Wondo Genet campus drinking water sources. Three water samples were taken at each water caching locations. Ten (10) water samples were collected from different locations of the Wondo Genet campus. Sampling sites for water were selected purposely which represents the entire water bodies.
Instead of this study small dam indicates the starting point of Wondo Genet campus drinking water sources rather than large dams constructed for other purpose. Taps were operated or run for at least 5 min prior to sampling to ensure collection of a representative sample (temperature and electrical conductivity were monitored to verify this). Each sample’s physico–chemical properties of water were measured in the field using portable meters (electrical conductivity, pH and temperature) at the time of sampling. Water samples were placed in clean containers provided by the analytical laboratory (glass and acid-washed polyethylene for heavy metals) and immediately placed on ice. Nitric acid was used to preserve samples for metals analysis.
Analysis of water samples
Determination of ph.
The pH of the water samples was determined using the Hanna microprocessor pH meter. It was standardized with a buffer solution of pH range between 4 and 9.
Measurement of temperature
This was carried out at the site of sample collection using a mobile thermometer. This was done by dipping the thermometer into the sample and recording the stable reading.
Determination of conductivity
This was done using a Jenway conductivity meter. The probe was dipped into the container of the samples until a stable reading will be obtained and recorded.
Determination of total dissolved solids (TDS)
This was measured using Gravimetric Method: A portion of water was filtered out and 10 ml of the filtrate measured into a pre-weighed evaporating dish. Filtrate water samples were dried in an oven at a temperature of 103 to 105 °C for \(2\frac{1}{2}\) h. The dish was transferred into a desiccators and allowed cool to room temperature and were weighed.
In this formula, A stands for the weight of the evaporating dish + filtrate, and B stands for the weight of the evaporating dish on its own Mahmud et al. ( 2014 ).
Chemical analysis
Chloride concentration was determined using titrimetric methods. The chloride content was determined by argentometric method. The samples were titrated with standard silver nitrate using potassium chromate indicator. Calcium ions concentrations were determined using EDTA titrimetric method. Sulphate ions concentration was determined using colorimetric method.
Microorganism analysis
In the membrane filtration method, a 100 ml water sample was vacuumed through a filter using a small hand pump. After filtration, the bacteria remain on the filter paper was placed in a Petri dish with a nutrient solution (also known as culture media, broth or agar). The Petri dishes were placed in an incubator at a specific temperature and time which can vary according the type of indicator bacteria and culture media (e.g. total coliforms were incubated at 35 °C and fecal coliforms were incubated at 44.5 °C with some types of culture media). After incubation, the bacteria colonies were seen with the naked eye or using a magnifying glass. The size and color of the colonies depends on the type of bacteria and culture media were used.
Statically analysis
All data generated was analyzed statistically by calculating the mean and compare the mean value with the acceptable standards. Data collected was statistically analyzed using Statistical Package for Social Sciences (SPSS 20).
Abbreviations
ethylene dinitrilo tetra acetic acid
Minstor of Health
nephelometric turbidity units
total dissolved solid
World Health Organization
Abebe L (1986) Hygienic water quality; its relation to health and the testing aspects in tropical conditions. Department of Civil Engineering, University of Tempere, Finland
Aremu MO et al (2011) Physicochemical characteristics of stream, well and borehole water sources in Eggon, Nasarawa State, Nigeria. J Chem Soc Nigeria 36(1):131–136
Google Scholar
Ayenew T (2004) Environmental implications of changes in the levels of lakes in the Ethiopian Rift since 1970. Reg Environ Chang 4:192–204
Article Google Scholar
Edimeh et al (2011) Physico-chemical parameters and some Heavy metals content of rivers Inachalo and Niger in Idah, Kogi State. J Chem Soc Nigeria 36(1):95–101
Ezeribe AL et al (2012) Physico-chemical properties of well water samples from some villages in Nigeria with cases of stained and mottle teeth. Sci World J 7(1):1–13
Jackson et al (2001) Water in changing world, Issues in Ecology. Ecol Soc Am, Washington, pp 1–16
Mahmud et al (2014) Surface water quality of Chittagong University campus, Bangladesh. J Environ Sci 8:2319-2399
Messeret B (2012) Assessment of drinking water quality and determinants of household potable water consumption in Simada district, ethiopia
MOH (2011) Knowledge, attitude and practice of water supply, environmental sanitation and hygiene practice in selected worked as of Ethiopia
Napacho A, Manyele V (2010) Quality assessment of drinking water in Temeke district (Part II): characterization of chemical parameters. Af J Environ Sci Technol 4(11):775–789
Sasikaran S et al (2012) Physical, chemical and microbial analysis of bottled drinking water. J Ceylon Medical 57(3):111–116
Soylak et al (2002) Chemical analysis of drinking water samples from Yozgat, Turkey. Polish J Environ Stud 11(2):151–156
WHO (1984) Guideline for drinking water quality. Health Criteria Support Inf 2:63–315
World Health Organization (1997) Basic Environmental Health, Geneva
World Health Organization (2004) Guidelines for drinking-water quality. World Health Organization, Geneva
World Health Organization (2006) In water, sanitation and health world health organization
WHO (2011) Guidelines for drinking-water quality, 4th edn. Geneva, Switzerland
Download references
Authors’ contributions
YM: participated in designing the research idea, field data collection, data analysis, interpretation and report writing; BA: participated in field data collection, interpretation and report writing. Both authors read and approved the final manuscript.
Authors’ information
Yirdaw Meride: Lecturer at Hawassa University, Wondo Genet College of Forestry and Natural Resources. He teaches and undertakes research on solid waste, carbon sequestration and water quality. He has published three articles mainly in international journals. Bamlaku Ayenew: Lecturer at Hawassa University, Wondo Genet College of Forestry and Natural Resources. He teaches and undertakes research on Natural Resource Economics. He has published three article with previous author and other colleagues.
Acknowledgements
Hawassa University, Wondo Genet College of Forestry and Natural Resources provided financial support for field data collection and water laboratory analysis. The authors thank anonymous reviewers for constructive comments.
Competing interests
The authors declare that they have no competing interests.
Author information
Authors and affiliations.
School of Natural Resource and Environmental Study, Wondo Genet College of Forestry and Natural Resources, Hawassa University, P.O. Box 128, Shashemene, Ethiopia
Yirdaw Meride & Bamlaku Ayenew
You can also search for this author in PubMed Google Scholar
Corresponding author
Correspondence to Yirdaw Meride .
Rights and permissions
Open Access This article is distributed under the terms of the Creative Commons Attribution 4.0 International License ( http://creativecommons.org/licenses/by/4.0/ ), which permits unrestricted use, distribution, and reproduction in any medium, provided you give appropriate credit to the original author(s) and the source, provide a link to the Creative Commons license, and indicate if changes were made.
Reprints and permissions
About this article
Cite this article.
Meride, Y., Ayenew, B. Drinking water quality assessment and its effects on residents health in Wondo genet campus, Ethiopia. Environ Syst Res 5 , 1 (2016). https://doi.org/10.1186/s40068-016-0053-6
Download citation
Received : 01 September 2015
Accepted : 06 January 2016
Published : 21 January 2016
DOI : https://doi.org/10.1186/s40068-016-0053-6
Share this article
Anyone you share the following link with will be able to read this content:
Sorry, a shareable link is not currently available for this article.
Provided by the Springer Nature SharedIt content-sharing initiative
- Drinking water
- Bacteriological
- Physico–chemical
Thank you for visiting nature.com. You are using a browser version with limited support for CSS. To obtain the best experience, we recommend you use a more up to date browser (or turn off compatibility mode in Internet Explorer). In the meantime, to ensure continued support, we are displaying the site without styles and JavaScript.
- View all journals
- Explore content
- About the journal
- Publish with us
- Sign up for alerts
- Perspective
- Open access
- Published: 18 August 2020
A framework for monitoring the safety of water services: from measurements to security
- Katrina J. Charles ORCID: orcid.org/0000-0002-2236-7589 1 ,
- Saskia Nowicki ORCID: orcid.org/0000-0001-9011-6901 1 &
- Jamie K. Bartram ORCID: orcid.org/0000-0002-6542-6315 2 , 3
npj Clean Water volume 3 , Article number: 36 ( 2020 ) Cite this article
5417 Accesses
32 Citations
14 Altmetric
Metrics details
- Environmental sciences
- Water resources
The sustainable developments goals (SDGs) introduced monitoring of drinking water quality to the international development agenda. At present, Escherichia coli are the primary measure by which we evaluate the safety of drinking water from an infectious disease perspective. Here, we propose and apply a framework to reflect on the purposes of and approaches to monitoring drinking water safety. To deliver SDG 6.1, universal access to safe drinking water, a new approach to monitoring is needed. At present, we rely heavily on single measures of E. coli contamination to meet a normative definition of safety. Achieving and sustaining universal access to safe drinking water will require monitoring that can inform decision making on whether services are managed to ensure safety and security of access.
Similar content being viewed by others
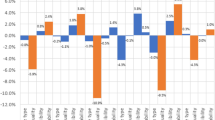
Integrating water-quality analysis in national household surveys: water and sanitation sector learnings of Ecuador
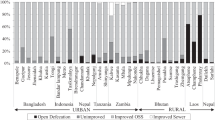
Indicators to complement global monitoring of safely managed on-site sanitation to understand health risks
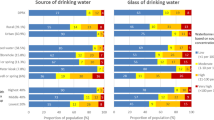
Safely managed drinking water services in the Democratic People’s Republic of Korea: findings from the 2017 Multiple Indicator Cluster Survey
Introduction.
Access to affordable, safe drinking water is critical for securing health gains from development. Significant gains were made in water access during the millennium development goal (MDG) period (1990–2015); however, the approach to drinking water safety relied on a binary improved/unimproved categorisation of water source types, approximating a crude sanitary inspection, which inadequately addresses water safety 1 . Building on the achievements of the MDG period, the sustainable development goals (SDGs) include a target for safe drinking water. The associated indicator for this target is based on water quality analysis for a one-off cross-sectional survey of a nationally representative sample of households and the primary water source that they use.
The term ‘safe’ was used in the MDGs (target 7c—‘halve the proportion of people without sustainable access to safe drinking water and basic sanitation’) and again in the SDGs (target 6.1—‘achieve universal and equitable access to safe and affordable drinking water for all’) to emphasise the importance that drinking water should not propagate disease; however, measurement of ‘safety’ has been an ongoing challenge. The intent and operationalisation of the SDGs—through the wording of targets and indicators—was developed through international participatory processes and built on the successes of the MDGs. The negotiations concerning targets and indicators demanded that these be supported by meaningful baseline data. These restrictions constrained the monitoring approach options, in this case to safety as defined by the Joint Monitoring Programme as Escherichia coli and a few selected ‘priority chemical contaminants’. In the coming decade, as we continue to achieve gains in access to water, we need to ensure our monitoring approaches move beyond quality to monitor the safety, and ongoing security, of drinking water services.
In this Special Collection on Monitoring drinking water quality for the Sustainable Development Goals , we reflect on the purposes of monitoring, considering the tools used and their limitations in guiding achievement of that purpose and of progress towards universal use of safe drinking water. Our reflections are framed around the prevention of transmission of infectious disease through drinking water, in both endemic and outbreak forms, and we focus on E. coli as the most common indicator used in drinking water safety monitoring. In developing a framework for monitoring the safety of drinking water safety, we recognise limitations in approaches to delivering safe drinking water, consider the historical pathways that have led us here, and explore opportunities to reimagine monitoring for safe drinking water.
A framework for monitoring drinking water safety
With reference to SDG target 6.1, the aim of drinking water monitoring is to track and advance progress towards universal access to safe drinking water. This indicates two important components of purpose—one concerns the intention to determine levels of coverage and compare them to the goal of universality; the second is the reference to safety as distinct from quality, which requires that the water be judged as to its fitness for human consumption.
The word ‘monitoring’ is defined by the notion of keeping track of something. Scientific dictionaries normally refine this to include two concepts: the ongoing nature of the activity, and the taking of periodic and programmed observations or measurements. Whether implicit or explicit, the definitions convey an understanding that monitoring ought to be designed with reference to a declared purpose, with the resulting data fit for the intended use. Monitoring for different purposes, to inform different decisions, will require different approaches and measures. For example, compliance monitoring tracks performance against a regulated standard such as a chemical or microbiological parameter, whereas operational monitoring tracks performance against process indicator limits such as for turbidity or residual chlorine 2 , 3 .
In Fig. 1 , we present a framework for monitoring drinking water safety. Monitoring (as it relates to prevention of infectious disease transmission through drinking water) is framed in sequential domains of concern, moving from taking single measurements of indicators or contaminants, to interpreting health hazard, tracking safety of services, and finally monitoring the prospective security of safe services. For the purposes of our discussion here, we use the term ‘safe’ to imply potable water, i.e. that which is fit for human consumption. The framework enables us to interrogate the role of indicators in monitoring drinking water safety. Further, we use it to illustrate the constraints, benefits, and interrelationships of different outlooks—in terms of both conceptualising drinking water safety and interpreting the findings of associated monitoring activities.
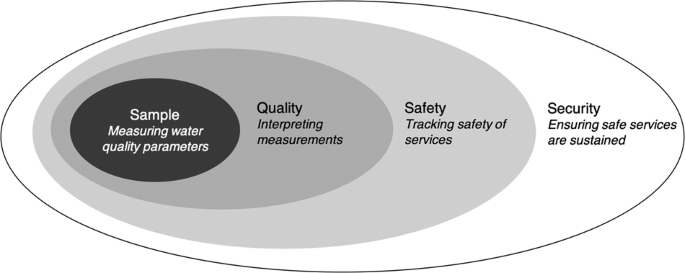
A framework for monitoring drinking water safety.
Sample: measuring water quality parameters
Water quality is measured to assess potential contamination. The most common measure used to determine microbial drinking water quality is E. coli . Used as an index of certain pathogens or as an indicator of faecal contamination, the presence of E. coli informs on the likelihood that pathogens are present 4 . E. coli are one of a methodologically defined group of indicators, referred to as total coliforms, that includes members of the genera Escherichia , Klebsiella , Enterobacter , Citrobacter and Serratia . Colony count approaches for coliform bacteria have been formally used to manage water quality in the UK since Report 71 5 was published 4 . E. coli were identified in the 1880s 4 , and were suggested as an indicator of water quality in 1892 6 . As ideas on what was required of an indicator organism advanced in the 1960s and 1970s 7 , 8 , E. coli became the preferred indicator of faecal contamination 9 . E. coli were recognised as ‘the more precise indicator of faecal pollution’ 10 because at the time they were thought to originate exclusively from human and warm-blooded animal faeces, in which they are always present in high quantities. Whereas, other coliform organisms were already known to originate from non-faecal sources as well as from faeces, making them less likely to be reliably associated with the presence of human pathogens 9 . Recognition of this, alongside advancements in methods, resulted in inclusion of E. coli as a preferred indicator of faecal contamination in the second edition of WHO’s Guidelines for Drinking Water Quality (GDWQ) in 1993 11 , and in the EU Drinking Water Directive in 1998 12 . In addition to E. coli , a less specific methodologically defined faecal indicator organism group, thermotolerant coliforms, is also recognised as useful in the GDWQ. Thermotolerant coliforms are a sub-group of coliforms, inclusive of E. coli , that grow at 44.5 °C. Use of this elevated temperature is intended to inhibit the growth of non- Escherichia coliforms, but mostly Citrobacter and Enterobacter are reduced, and even then, not all strains of those genera 13 . While fixed ratios of E. coli to thermotolerant coliforms are sometimes reported, this relationship varies widely 14 —including by climate 15 and water type 16 , and by the enumeration method 14 . Thermotolerant coliforms are sometimes referred to as ‘faecal coliforms’, a misnomer as they do not all originate from faeces 17 . An analytical result of zero (or more correctly <1) thermotolerant coliforms in a water sample would imply zero E. coli , but might be more difficult to achieve, whereas a result positive for thermotolerant coliforms does not confirm the presence of E. coli .
Measurement in our framework is the test that can help to assess if a ‘glass’ of water is contaminated, either by direct measurement of contaminants or using indicators. It is worth noting that the volume used in analyses is typically 100 mL, a volume equated with a ‘glass of water’ 18 . The first GDWQ recommended 100 mL sample sizes while also recognising that it would be ‘statistically more meaningful to examine larger samples, possibly 200, 500, or 1000 ml’ 19 .
Quality: interpreting measurements
Measurement results are often interpreted in terms of the hazard they represent. This is too often used to define the quality of water as ‘safe’ or not. For example, water is considered safe with respect to measured parameters if it does not exceed relevant guidelines or standards. This positivist, normative definition assumes that all potential hazards are known, are measurable, and have been considered. Its limitations are exemplified by widespread occurrence of arsenic in groundwater that had been previously declared ‘safe’ in Bangladesh in the 1990s 20 , 21 .
Drinking water may contain numerous potential health threats for which guidelines have not been established due to insufficient or inconsistent evidence, the low-priority given to threats deemed to be ‘only’ locally significant in few settings, and as yet unrecognised hazards. Guidelines are revisited as new evidence emerges. But guidelines (and therefore our understanding of safe water by normative definitions) are constrained: firstly, by practical considerations, such as the limits of readily available detection methods (for which arsenic is again an example 22 ) and treatment technologies; and secondly, by political considerations such as trade-offs among competing hazards as is the case of disinfectants versus disinfection by-products.
Consequently, judgments of safety are assisted by objective definitions. The GDWQ 23 defines safe drinking-water as that which “does not represent any significant risk to health over a lifetime of consumption, including different sensitivities that may occur between life stages”, where significant risk is defined in terms of the tolerable burden of disease of 10 −6 disability adjusted life years per person per year. The UK also uses an objective definition in their water regulations: the term ‘wholesome’ is applied to water that ‘does not contain any micro-organism… or parasite or any substance …at a concentration or value which would constitute a potential danger to human health’ whether or not a standard has been set 24 .
When E. coli is detected, it is interpreted as a health hazard—in keeping with the notion that it is a ‘faecal indicator bacteria’ (FIB), a long-established notion in sanitary microbiology. Several authors have proposed necessary and desirable characteristics of the ideal faecal indicator 25 , 26 , 27 , and reviewed the degree of fit of candidate organisms against them. Here, we selectively use the criteria that are included in the GDWQ. It is important to revisit these criteria to reflect on the role that E. coli performs as the most common measure to assess progress against SDG 6.1. E. coli align with several of the GDWQ criteria: 23 they are (generally) not pathogens themselves; they are universally present in faeces of humans and animals in large numbers; they are present in higher numbers than faecal pathogens; and they are ‘readily detected by simple, inexpensive culture methods’ (p148). However: while the majority of detected E. coli are not pathogens, a significant subset are pathogenic; and while methods may be simple and inexpensive compared to tests for specific pathogens, they remain sufficiently expensive in most settings that they do not meet the intent of this criterion articulated by Medema et al. 9 : that indicator tests should be inexpensive ‘thereby permitting numerous samples to be taken’.
While field tests have been developed, for regulatory purposes, most E. coli tests are undertaken in a laboratory. This requires that a cold chain be maintained during sample transport and that samples be processed within 6 h, which can be logistically and financially problematic, limiting the validity of results due to changes in the sample composition during storage. For example, in Colombia an estimated 30% of rural water samples would require storage for more than 6 h en route to the laboratory 28 .
Three of the WHO FIB criteria are not met by E. coli
Firstly, some E. coli multiply in natural waters. Such growth has been demonstrated in soils 29 , 30 , sediments 31 , and water columns 32 including in drinking water reservoirs 33 . It has also been shown in the biofilms of distribution systems 34 and in handpumps 35 . Conditions for growth require temperatures over 15 °C, assimilable carbon availability, and absence of disinfectant residuals 36 . While these conditions are uncommon in large utility systems in temperate countries, they are common in many other water systems; for example, shallow groundwaters are over 15 °C in much of the world 37 and contain high loads of organic carbon 38 .
Secondly, E. coli are less robust than many pathogens, so they neither persist in water nor respond to treatment processes in a similar fashion to faecal pathogens. E. coli die-off quicker than many viral or protozoan pathogens in surface water and groundwater 39 , 40 , 41 and during treatment 23 . E. coli are larger and have different surface charge characteristics than viruses and, therefore, are more readily trapped in filters and soil matrices 42 . The different behaviour of pathogens and E. coli illustrates why there is no direct correlation between concentrations of indicators and pathogens 43 . This lack of correlation is a limitation of quantitative microbial risk assessment approaches, since health risk from pathogens is extrapolated from E. coli measurements 44 .
Since the presence of E. coli is interpreted as indicating a health hazard, ‘immediate investigative action’ 23 is recommended when E. coli are detected in drinking water. However, because of the limitations described above, presence of E. coli indicates that, at the time the test was taken, there had either been recent faecal contamination, or a large faecal contamination event less recently, or environmental conditions were appropriate for growth of E. coli . Conversely, the absence of E. coli does not definitively confirm the absence of faecal pathogens. To interpret the results of E. coli tests, it becomes necessary to have more information available. Throughout the water safety literature, it is emphasised that E. coli (or FIB) are most useful as a component of a programme of measurements, not as a single test result 5 , 9 , 45 , 46 . We expand on this in the next section.
A single (or infrequent) test of water for E. coli , and subsequent interpretation of the health hazard, is widely understood as water quality ‘monitoring’, but is not able to advance water safety. E. coli , the most common measure of progress towards universal safe water, has strengths and limitations when we try to use it to infer health hazard. If we use an objective definition of safe drinking water, even within the context of infectious diseases, one test is insufficient to identify and manage threats. For E. coli , when the interpretation of the result is also unclear, a gap emerges between measurement and the stated aim of safety. With reliance on E. coli , and in the absence of other information, results are subject to confirmation bias: If E. coli are not detected in a second test then it is often assumed that the first was wrong and there is no health hazard, rather than considering variability in occurrence and detection. Or if an outbreak occurs, detection of E. coli is assumed to confirm that the water represents a health hazard, however, E. coli may be present for reasons other than recent faecal contamination, and without validation testing it is not possible to ascribe the source of the outbreak.
Safety: tracking safety of services
The preceding domain of our framework, focusing on interpreting measurements, deals simplistically with risk in terms of the likelihood of experiencing a hazard (e.g. of contracting a disease) given a specific exposure. Here we consider safety, which is not simply the inverse of hazard. Effective disease prevention demands consistent hazard-free status. A water supply is not ‘safe’ if it produces one glass of hazard-free water, nor if it delivers pathogen-laden water, briefly, once a year. Empirically, even in highly compliant water supply systems, Setty et al. 47 show that disease (diarrhoea) prevalence increased following changes in water quality due to rainfall. The importance of consistency is further illustrated by the modelling work of Hunter et al. 48 , which suggests an increased risk of over 10% in the probability of annual infection from enteropathogenic E. coli (12.7%), Cryptosporidium (18%) and rotavirus (12%) associated with switching from treated to untreated drinking water for 1 day.
Because sampling and analysis provide a snapshot of quality at the moment of sampling; and because samples of water necessarily represent a negligible fraction of the volume and time of water supplied, assessing on-going safety demands that we move from making and interpreting single measurements to planning sequences of measurements i.e. ‘monitoring’.
There is abundant evidence that E. coli concentrations in water vary rapidly and across orders of magnitude. This is true within natural waters due to non-random distribution 49 , from hazardous events 47 or failures in control measures, which routine sampling regimes do not readily capture as they are limited by logistics. For example, samples are disproportionately taken in mornings and on days earlier in the working week to facilitate transport, analysis and reporting during normal work hours 18 . Here, online measures, such as turbidity or chlorine residual, can improve understanding of temporal variability 47 , 50 and interpretation of other measurements.
Measurements only provide evidence of what the quality was, so management approaches that integrate understanding of system performance into planning are needed to oversee water safety on an ongoing basis. Prospective management of safety requires and builds on the knowledge of historical measurements. It combines evidence that a system has reliably delivered potable water, based on a programmed series of measurements, with knowledge that controls are in place to ensure that perturbations do not compromise quality. At this level of the framework, we have moved from a focus on measurements, which inform on the water system safety yesterday (which is what E. coli tests currently help us understand), to consideration of safety for tomorrow. This prospective safety perspective and its emphasis on ensuring adequate conditions is exemplified by sanitary inspection and water safety plans (WSPs).
Sanitary inspection originated as an adjunct, to water quality measurement. Victorian hygiene literature is replete with examples, and almost a century ago, Prescott and Winslow 51 stated that ‘the first attempt of the expert called in to pronounce upon the character of a potable water should be to make a thorough sanitary inspection’. This illustrates understanding that, even in the absence of contamination at a moment of sampling, a system that is vulnerable to contamination is not safe. As bacteriological methods developed, and their limitations were recognised, these preventative approaches continued to be valued. For example, the first edition of the GDWQ stated that, for non-piped systems, ‘considerable reliance must be placed on sanitary inspection and not exclusively on the results of bacteriological examination’ 19 . Equally, it advised managers of untreated water for piped supplies to include in their assessment of safety both frequent bacteriological results showing the absence of faecal coliforms and information on whether ‘sanitary inspection has shown the catchment area and storage conditions to be satisfactory’ 19 .
In the sense used here, a sanitary inspection is a visual inspection of a piece of water system infrastructure, with the objective of identifying physical factors that could facilitate contamination. It is exhaustive in the sense that all observable faults are considered, but not comprehensive in the sense that not all faults are detectable by visual inspection. Sanitary inspection, reviewed and explored substantively in the paper by Kelly and Bartram 52 , is widely used by those working on rural water systems, where it is frequently applied to community water sources such as boreholes with handpumps.
Sanitary inspection was one of the tools that inspired the development of the concept of WSPs, along with the hazard analysis and critical control points (HACCP) approach, failure mode analysis, quality management, and multi-barrier approaches (the importance of these approaches is highlighted in Kelly and Bartram’s 52 results). Indeed, sanitary inspection is a key component of WSPs, which extend the principles of sanitary inspection to the whole system (‘catchment-to-consumer’).
WSPs and similar systematic risk-based approaches have demonstrated benefits for reducing temporal variation in water quality 47 , as well as reducing the health burden 53 . One of the key attributes of a WSP 54 is that there is evidence that a water supply system can achieve safe water through validation and verification procedures. In validation, evidence is gathered that a water system can effectively meet water quality targets; this may use a variety of tools, including challenging a water system with different conditions and organisms. Verification provides ongoing evidence that a water system is delivering water of the desired quality, for which regular E. coli measurements provides a useful tool. With the system performance characterised through validation and verification, an individual E. coli measurement can be meaningfully interpreted.
Monitoring water safety requires frequent data collection, underpinned by knowledge of system performance and maintenance. Furthermore, to be effective, monitoring data must be available and useful for decision makers, and should support stakeholder cooperation rather than threaten it 55 .
Security: ensuring safe services are sustained
As the SDG target of universal access is progressively achieved, the importance of the future sustainability of safe drinking water supply becomes increasingly apparent, i.e. attention turns towards the risk that those with access might experience a reduction of the level of service, or a loss of service 56 . While for the purpose of this article we focus on safety of drinking water for human consumption, the level of service we aim to secure includes the broader aspects of the human right to water on which the SDG indicators are based 57 . Securing appropriate levels of quantity, reliability, accessibility, and affordability of water that is fit for purpose, are essential to achieving the health-based targets that WSPs are designed to meet 58 .
Terms describing sustainability and sustaining services are interpreted inconsistently. While our preceding reflections concern monitoring for sustained achievement of safety and its implied continuation, here we use the term security in a prospective manner similar to that of ‘sustainable development’ i.e. in a sense that differentiates management of the day to day and familiar (safety) from adequacy for the long term and against the unfamiliar (security). We use three factors to illustrate the potential for attained access to be systematically undermined: demographic change, climate change, and increasing water pollution. Although we focus on these three types of systemic change, we recognise that there are others, such as economic volatility and armed conflict, that are important at this final level of the framework.
Demographic trends warn us of impending risks to availability of sufficient quantities of water because of increasing population, and accelerating demand for water as populations change lifestyles with urbanisation and increasing affluence 59 , 60 . In Kenya, for example, water is scarce (647 m 3 per capita in 2006 61 ) and by 2030, the population is projected to increase by over 80%, with a 50% increase in the proportion of urban dwellers. Increases in water demand will coincide for domestic supply, agriculture and industry (supplying both domestic and international markets 62 ), and good regulation will be necessary to prevent damaging shortages.
Further to demographic pressure, climate change will have substantial consequences for water access. Shortages in Cape Town and São Paulo have highlighted the vulnerability of water supplies to climatic events, and the importance of appropriate management 63 . With climate change, more frequent higher intensity rainfall events will increase the risk of infrastructure damage 64 . Rising temperatures and increasing evapotranspiration rates will reduce available water and increase competing water demand for irrigation 65 . And water quality will deteriorate due to heavier and more erratic rainfall 47 , 66 , increasing release of glacial flows with associated geochemical hazards 67 , and increasing salinity from rising sea levels and expanding irrigation.
In addition to climate change impacts, the problem of water quality deterioration is compounded by pollution. Pollution threatens health directly (through contamination of drinking water that treatment processes do not remove) or indirectly (if chemicals make water unpalatable). Thus, increasing pollution threatens to reduce access to safe drinking water; for example, substantial investments are needed in Dhaka, Bangladesh, to ensure continued access where industrial growth has resulted in the need to pump water from over 30 kms away to avoid local pollution 68 . Environmental water pollution is addressed through SDG 6.3: to ‘improve water quality by reducing pollution…’. Realisation of this goal will require combatting pollution from domestic, agricultural and industrial sources.
To ensure we are working towards sustainable access to safe water, we need tools that can measure and track this progress. Without appropriate indicators, we will continue to focus on access rather than sustainability, potentially misdirecting resources.
New monitoring tools are needed to assess security of safe water supplies
The MDGs, and now SDGs, are based on the notion of provision: increasing the proportion of people with reliable access to affordable, safe drinking water. There remain challenges to achieving the SDG target of universal access, especially for difficult-to-reach populations. There are also practical limits of measuring universality. As we approach the target of universality, however, we need to consider how to shift to a security perspective that encompasses sustainability of high levels of service. Thanks to the achievements of the MDGs and SDGs, 71% of the global population have access to safely managed water services 69 . But because of this success, it is now possible and necessary to adopt a security perspective and to target prospective, inter-generational access to safe water in our changing world.
The participatory, political nature of the processes that define global targets and indicators creates a conservative environment that hinders innovation and diffusion-adoption of improved approaches. We argue that now is the time to start planning for 2030 and beyond, to support a change in focus from access to security of sustainable, safe water supply services and to ensure we can provide the level of evidence needed for adoption of better indicators by the United Nations committees and processes. We recall that the initial Rapid Assessment of Drinking Water Quality (RADWQ) research, done in 2004/2005 15 , created a platform for change that advanced a recognition of drinking water quality in the MDGs, and then monitoring of quality in the SDGs. In 2020, 5 years into the SDGs, where are the new tools we will need for 2030 and beyond?
Through our framework for monitoring drinking water safety we have deconstructed ‘drinking water quality monitoring’, reflecting on its purpose and component parts. Measurement of drinking water quality alone will not deliver the changes needed for safe water. We have become highly reliant on E. coli as a means to assess drinking water quality. It is critical that we remember, however, that the goal of safe water supply is fitness for human consumption, not absence of E. coli . There are a range of measurement tools available that can facilitate regular checks to track direct or indirect changes in water quality or system performance. These tools are important, but cheaper, quicker methods to measure water quality parameters are not enough. The usefulness of measures, such as E. coli , in communicating the problem of water quality has to be tempered by the risk that we lose sight of their purpose and neglect the range of tools needed to achieve safe water and to sustain improvements.
To assess and manage the safety and security of drinking water services, we need monitoring that includes more than direct water quality measures. Through our framework we advocate for this next step. For water safety, there has been consistent emphasis in the GDWQ on the importance of frequent water testing being complemented by knowledge of risks from sanitary inspections and accompanied by systematic management approaches like WSPs. Increased regulation of water services can be anticipated to increase data availability 57 and create an opportunity to focus on management indicators for monitoring the application, oversight and audits of WSPs and sanitary inspections.
These practices, supported by routine monitoring, are essential for safety, and they contribute to ensuring security of drinking water services in the face of threats from demographic and climate change, and pollution. To fully progress from ‘safety’ to ‘security’, however, will require innovations in monitoring that go beyond current practices. There is an opportunity to act smartly, invest strategically, and accelerate progress by incorporating a security perspective. This perspective should enable us to account for prospective long-term drivers that threaten the ongoing sustainability of access to safe water.
Data availability
No datasets were generated or analysed during the current study.
Code availability
No code was generated or used during the current study.
Bain, R. et al. Fecal contamination of drinking-water in low- and middle-income countries: a systematic review and meta-analysis. PLoS Med. 11 , e1001644 (2014).
Article Google Scholar
Charles, K. & Pond, K. Drinking water quality regulations. Routledge Handb. Water Health https://doi.org/10.4324/9781315693606 (2015).
Rahman, Z., Crocker, J., Chang, K., Khush, R. & Bartram, J. A comparative assessment of institutional frameworks for managing drinking water quality. J. Water Sanit. Hyg. Dev. 1 , 242–258 (2011).
Ashbolt, N. J., Grabow, W. O. K. & Snozzi, M. Indicators of microbial water quality. in Water Quality: Guidelines, Standards and Health: Assessment of Risk and Risk Management for Water-Related Infectious Disease (eds Fewtrell, L. & Bartram, J. K.) (World Health Organization, London, UK, 2001).
HMSO. The Bacteriological Examination of Water Supplies. Reports on Public Health and Medical Subjects, 1st edn, No. 71 (1934).
Leclerc, H., Mossel, D. A. A., Edberg, S. C. & Struijk, C. B. Advances in the Bacteriology of the Coliform Group: Their Suitability as Markers of Microbial Water Safety . (2001).
Bonde, G. J. Bacteriological methods for estimation of water pollution. Health Lab. Sci. 3 , 124–128 (1966).
CAS Google Scholar
Gordon, D. M. The ecology of Escherichia coli . in Escherichia coli: Pathotypes and Principles of Pathogenesis (ed Donnenberg, M.S.) 3–20 (Academic Press, 2013).
Medema, G. J. et al. Safe drinking water: an ongoing challenge. in Assessing Microbial Safety of Drinking Water: Improving Approaches and Methods (eds O. E. C. D. & WHO) (2003).
WHO. Guidelines for Drinking-water Quality: Third edition, incorporating the first and second Addenda, Volume 1 Recommendations. (World Health Organisation, 2008).
WHO. Guidelines for Drinking Water Quality 2nd Edition. Volume 1. Recommendations. (World Health Organisation, 1993).
Anon. Council Directive 98/83/EC of 3 November 1998 on the quality of water intended for human consumption. Off. J. Eur. Commun. 5.12.98 , L330/32–L330/53 (1998).
Gray, N. & Gleeson, C. The Coliform Index and Waterborne Disease. https://doi.org/10.4324/9780203476888 (1996).
Hamilton, W. P., Kim, M. & Thackston, E. L. Comparison of commercially available Escherichia coli enumeration tests: implications for attaining water quality standards. Water Res. 39 , 4869–4878 (2005).
Article CAS Google Scholar
WHO & UNICEF. Rapid Assessment of Drinking-Water Quality: A Handbook for Implementation . (2012).
Mahmud, Z. H. et al. Occurrence of Escherichia coli and faecal coliforms in drinking water at source and household point-of-use in Rohingya camps, Bangladesh. Gut Pathog. 11 , 1–11 (2019).
Allen, M. A. The Public Health Significance of Bacterial Indicators in Drinking Water. in Coliforms and E. coli, Problem or Solution ? (eds Kay, D. & Fricker, C.) 176–181 (Royal Society of Chemistry, 1997).
Geldreich, E. E. Reinventing microbial regulations for safer water supplies. in Coliforms and E. coli, Problem or Solution ? (eds Kay, D. & Fricker, C.) 218–234 (Royal Society of Chemistry, 1997).
WHO. Guidelines for Drinking Water Quality. First Edition. Vol 1 Recommendations. (World Health Organisation, 1984).
Pepper, D. Bangladeshis poisoned by arsenic sue British organisation. Lancet 367 , 199–200 (2006).
George, C. M. The arsenic crisis in Bangladesh. in Routledge Handbook of Water and Health (ed Bartram, J.) 685–692 https://doi.org/10.4324/9781315693606-82 (Routledge, 2015).
WHO. Arsenic in Drinking-water Background Document for Development of WHO Guidelines for Drinking-Water Quality . (World Health Organisation, 2011).
WHO. Guidelines for Drinking-water Quality 4th ed., WHO, Geneva, p. 340. World Health Organization https://doi.org/10.1016/S1462-0758(00)00006-6 (2011).
Anon. Water, England and Wales The Water Supply (Water Quality) Regulations SI 2016/614. Water, England and Wales 52 (2016).
Waite, W. M. Drinking water quality regulation—a European perspective. in Coliforms and E. coli, Problem or Solution ? (eds Kay, D. & Fricker, C.) 208–217 (Royal Chemistry Society, 1997).
Geldreich, E. E. Coliforms: a new beginning to an old problem. in Coliforms and E. coli, Problem or Solution ? (eds Kay, D. & Fricker, C.) 3–11 (Royal Society of Chemistry, 1997).
Mossel, D. A. A. Index and indicator organisms: a current assessment of their usefulness and significance. Food Technol. Aust. 30 , 212–219 (1978).
Google Scholar
Wright, J. et al. Water quality laboratories in Colombia: a GIS-based study of urban and rural accessibility. Sci. Total Environ. 485–486 , 643–652 (2014).
Brennan, F. P., O’Flaherty, V., Kramers, G., Grant, J. & Richards, K. G. Long-term persistence and leaching of Escherichia coli in temperate maritime soils. Appl. Environ. Microbiol. 76 , 1449–1455 (2010).
Brennan, F. P., Abram, F., Chinalia, F. A., Richards, K. G. & O’Flaherty, V. Characterization of environmentally persistent Escherichia coli isolates leached from an Irish soil. Appl. Environ. Microbiol. 76 , 2175–2180 (2010).
Haller, L., Poté, J., Loizeau, J. L. & Wildi, W. Distribution and survival of faecal indicator bacteria in the sediments of the Bay of Vidy, Lake Geneva, Switzerland. Ecol. Indic. https://doi.org/10.1016/j.ecolind.2008.08.001 (2009).
Pote, J. et al. Persistence and growth of faecal culturable bacterial indicators in water column and sediments of Vidy Bay, Lake Geneva, Switzerland. J. Environ. Sci. 21 , 62–69 (2009).
Ashbolt, N. J., Dorsch, M. R., Cox, P. T. & Banens, B. Blooming E. Coli, what do they mean? in Coliforms and E. coli, Problem or Solution ? (eds Kay, D. & Fricker, C.) (The Royal Society of Chemistry, Cambridge, England, 1997).
Block, J. C., Muteaux, L., Gatel, D. & Reasoner, D. J. Survival and growth of E. coli in drinking water distribution systems. in Coliforms and E. coli, Problem or Solution ? (eds Kay, D. & Fricker, C.) 157–167 (Royal Society of Chemistry, 1997).
Ferguson, A. S. et al. Hand-pumps as reservoirs for microbial contamination of well water. J. Water Health 9 , 708–717 (2011).
LeChavallier, M. W. Conditions favouring coliform and HPC bacterial growth in drinking-water and on water contact surfaces. in Heterotrophic Plate Counts and Drinking-water Safety: The Significance of HPCs for Water Quality and Human Health (eds Bartram, J., Cotruvo, J., Exner, M., Fricker, C. & Glasmacher, A.) 177–198 (WHO, 2003).
Benz, S. A., Bayer, P. & Blum, P. Global patterns of shallow groundwater temperatures. Environ. Res. Lett . 12 , 34005 (2017).
McDonough, L. K. et al. Changes in global groundwater organic carbon driven by climate change and urbanization. Nat. Commun. 11 , 1–10 (2020).
Leclerc, H., Mossel, D. A. A., Edberg, S. C. & Struijk, C. B. Advances in the bacteriology of the coliform group: their suitability as markers of microbial water safety. Annu. Rev. Microbiol. 55 , 201–234 (2001).
Osborn, M. J. et al. Indicators for Waterborne Pathogens . (The National Academies Press, 2004).
Murphy, H. Persistence of Pathogens in Sewage and Other Water Types. in Global Water Pathogen Project (eds Rose, J. & Jiménez-Cisneros, B.) https://doi.org/10.14321/waterpathogens.51 (2017).
Taylor, R., Cronin, A., Pedley, S., Barker, J. & Atkinson, T. The implications of groundwater velocity variations on microbial transport and wellhead protection—review of field evidence. FEMS Microbiol. Ecol. 49 , 17–26 (2004).
Payment, P. & Locas, A. Pathogens in water: value and limits of correlation with microbial indicators. Ground Water 49 , 4–11 (2011).
Bivins, A. W. et al. Estimating infection risks and the global burden of diarrheal disease attributable to intermittent water supply using QMRA. Environ. Sci. Technol. 51 , 7542–7551 (2017).
WHO. Surveillance of Drinking Water Quality . (WHO, 1976).
Taylor, E. W., Beale, J. F., Suckling, E. V. & Thresh, J. C. The Examination of Waters and Water Supplies . (Churchill, 1958).
Setty, K. E. et al. Time series study of weather, water quality, and acute gastroenteritis at Water Safety Plan implementation sites in France and Spain. Int. J. Hyg. Environ. Health 221 , 714–726 (2018).
Hunter, P. R., Zmirou-Navier, D. & Hartemann, P. Estimating the impact on health of poor reliability of drinking water interventions in developing countries. Sci. Total Env. 407 , 2621–2624 (2009).
Kirchman, D., Sigda, J., Kapuscinski, R. & Mitchell’, R. Statistical analysis of the direct count method for enumerating bacteria. Appl. Environ. Microbiol . 44 (1982).
Setty, K. et al. Assessing operational performance benefits of a Water Safety Plan implemented in Southwestern France. Perspect. Public Health 138 , 270–278 (2018).
Prescott, S. C. & Winslow, C.-E. A. Elements of Water Bacteriology, with Special Reference to Sanitary Analysis . (Wiley, 1931).
Kelly, E. R., Cronk, R., Kumpel, E., Howard, G. & Bartram, J. How we assess water safety: a critical review of sanitary inspection and water quality analysis. Sci. Total Environ. 718 , 137237 (2020).
Gunnarsdottir, M. J. Benefits of Water Safety Plans: Microbiology, Compliance, and Public Health . https://doi.org/10.1021/es300372h (2012).
WHO. Water Safety Plan Manual: Step-by-step risk management for drinking-water suppliers. WHO Library . https://doi.org/10.1111/j.1752-1688.1970.tb00528.x (2009).
Nowicki, S., Koehler, J. & Charles, K. J. Including water quality monitoring in rural water service provision: why safe water requires challenging the quantity versus quality dichotomy. npj Clean Water 3 , 1–9 (2020).
Bradley, D. J. & Bartram, J. K. Domestic water and sanitation as water security: monitoring, concepts and strategy. Philos. Trans. R. Soc. A Math. Phys. Eng. Sci . 371 , 20120420 (2013).
Anon. Metadata on 6.1.1. (2017). Available at: https://unstats.un.org/sdgs/metadata/files/Metadata-06-01-01.pdf . Accessed 1 May 2020.
WHO. Guidelines for Drinking-Water Quality: Fourth Edition Incorporating the First Addendum . (WHO Press, 2017).
Damania, R. et al. Uncharted Waters. The New Economics of Water Scarcity and Variability . (2017).
Bartram, J., Elliott, M. & Chuang, P. Getting wet, clean, and healthy: why households matter. Lancet 380 , 85–86 (2012).
UN-WATER. Kenya National Water Development Report: Case Study. A WWAP Case Study Prepared for the 2nd UN World Water Development Report: Water, a Shared Responsibility . (2006).
Mendes, D. M. & Paglietti, L. Kenya Irrigation Market Brief . (FAO and IFC, 2015).
Muller, M. Cape Town’s drought: don’t blame climate change. Nature 559 , 174–176 (2018).
Howard, G. et al. Securing 2020 vision for 2030: climate change and ensuring resilience in water and sanitation services. J. Water Clim. Chang. https://doi.org/10.2166/wcc.2010.205 (2010).
Taye, M. T., Dyer, E., Hirpa, F. A. & Charles, K. Climate change impact on water resources in the Awash basin, Ethiopia. Water 10 , 1–16 (2018).
Auld, H., MacIver, D. & Klaassen, J. Heavy rainfall and waterborne disease outbreaks: the walkerton example. J. Toxicol. Environ. Heal. Part A 67 , 1879–1887 (2004).
Brown, G. H., Tranter, M. & Sharp, M. J. Experimental investigations of the weathering of suspended sediment by alpine glacial meltwater. Hydrol. Process. 10 , 579–597 (1996).
Chowdhury, F. J., Ahmad, Z. U. & Aalderink, H. Protecting the Meghna river. A Sustainable Water Resource for Dhaka . (Asian Development Bank, 2019).
UNICEF & WHO. Progress on household drinking water, sanitation and hygiene 2000–2017. Special focus on inequalities. Progress on Drinking Water, Sanitation and Hygiene 2000–2017 (2019).
Download references
Acknowledgements
K.C. and S.N. are supported by the REACH programme funded by UK Aid from the UK Department for International Development (DFID) for the benefit of developing countries (Aries Code 201880). However, the views expressed and information contained in it are not necessarily those of or endorsed by DFID, which can accept no responsibility for such views or information or for any reliance placed on them. The authors thank the health-related water community for all the discussions that have informed this work.
Author information
Authors and affiliations.
School of Geography and the Environment, University of Oxford, South Parks Road, Oxford, OX1 3QY, UK
Katrina J. Charles & Saskia Nowicki
The Water Institute at UNC, Gillings School of Global Public Health, University of North Carolina at Chapel Hill, Chapel Hill, NC, USA
Jamie K. Bartram
School of Civil Engineering, University of Leeds, Leeds, UK
You can also search for this author in PubMed Google Scholar
Contributions
K.C. and J.B. conceived of and drafted the paper. S.N. reviewed and commented throughout the process.
Corresponding author
Correspondence to Katrina J. Charles .
Ethics declarations
Competing interests.
The authors declare no competing interests.
Additional information
Publisher’s note Springer Nature remains neutral with regard to jurisdictional claims in published maps and institutional affiliations.
Rights and permissions
Open Access This article is licensed under a Creative Commons Attribution 4.0 International License, which permits use, sharing, adaptation, distribution and reproduction in any medium or format, as long as you give appropriate credit to the original author(s) and the source, provide a link to the Creative Commons license, and indicate if changes were made. The images or other third party material in this article are included in the article’s Creative Commons license, unless indicated otherwise in a credit line to the material. If material is not included in the article’s Creative Commons license and your intended use is not permitted by statutory regulation or exceeds the permitted use, you will need to obtain permission directly from the copyright holder. To view a copy of this license, visit http://creativecommons.org/licenses/by/4.0/ .
Reprints and permissions
About this article
Cite this article.
Charles, K.J., Nowicki, S. & Bartram, J.K. A framework for monitoring the safety of water services: from measurements to security. npj Clean Water 3 , 36 (2020). https://doi.org/10.1038/s41545-020-00083-1
Download citation
Received : 01 December 2019
Accepted : 14 July 2020
Published : 18 August 2020
DOI : https://doi.org/10.1038/s41545-020-00083-1
Share this article
Anyone you share the following link with will be able to read this content:
Sorry, a shareable link is not currently available for this article.
Provided by the Springer Nature SharedIt content-sharing initiative
This article is cited by
- Freya Mills
- Juliet Willetts
npj Clean Water (2024)
Addressing gaps in data on drinking water quality through data integration and machine learning: evidence from Ethiopia
- Alemayehu A. Ambel
- Robert Bain
- Tom Slaymaker
npj Clean Water (2023)
When Water Quality Crises Drive Change: A Comparative Analysis of the Policy Processes Behind Major Water Contamination Events
- Nameerah Khan
- Katrina J. Charles
Exposure and Health (2023)
Occurrence of waterborne pathogens and antibiotic resistance in water supply systems in a small town in Mozambique
- Elisa Taviani
- Harold van den Berg
- Giuliana Ferrero
BMC Microbiology (2022)
Measuring the gaps in drinking water quality and policy across regional and remote Australia
- Paul R. Wyrwoll
- R. Quentin Grafton
npj Clean Water (2022)
Quick links
- Explore articles by subject
- Guide to authors
- Editorial policies
Sign up for the Nature Briefing: Anthropocene newsletter — what matters in anthropocene research, free to your inbox weekly.

Ceramic membranes synthesized using fly ash pulp and paper boiler for COD and BOD removal from river
- Original Paper
- Published: 13 September 2024
Cite this article
- I. Amri ORCID: orcid.org/0000-0003-2548-7153 1 , 3 ,
- K. H. Pratama 1 ,
- S. Arumnika 1 ,
- Z. Meldha 1 &
- R. Rohani 2
Industrial developments and settlements along the Siak River have affected the quality of the river water. Based on laboratory tests, the COD and BOD content of Siak River water still exceeds the standard set under Government Regulation No. 22 of 2021. This research was conducted to analyze the role of variations in the composition and size of materials of clay, fly ash, and sawdust, in synthesizing ceramic membranes for COD and BOD removal of Siak River water. These residual materials from pulp and paper industry were used for ceramic membranes synthesis and further applied in drinking water treatment. In this study, ceramic membranes with a diameter of 11 cm, a thickness of 0.5 cm, and a combustion temperature of 900 °C were obtained and the composition of clay, fly ash, and sawdust of the ceramic membranes was varied in terms of ratio, respectively, at 32.5%:60%:7.5%, 45%:45%:10%, and 70%:25%:5%. Meanwhile, the variations in the size of the materials were as follows: 60, 80, and 100 mesh. The obtained results have shown that the COD and BOD content of Siak River water was reduced during the filtration process using the ceramic membranes, with the greatest percentage reductions in COD and BOD content (48.9% and 64.6%, respectively). The highest removals percentage were recorded upon using the ceramic membrane M8 (with a composition of 60% fly ash, 32.5% clay, 7.5% sawdust and particle size of 100 mesh). This result indicated that the ceramic membranes can be effectively used for the targeted application.
This is a preview of subscription content, log in via an institution to check access.
Access this article
Subscribe and save.
- Get 10 units per month
- Download Article/Chapter or eBook
- 1 Unit = 1 Article or 1 Chapter
- Cancel anytime
Price includes VAT (Russian Federation)
Instant access to the full article PDF.
Rent this article via DeepDyve
Institutional subscriptions
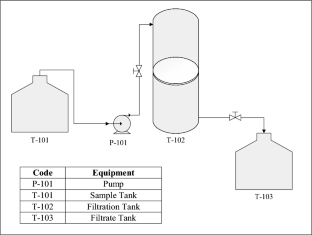
Explore related subjects
- Environmental Chemistry
Agmalini S, Narke NL, Subriyer N (2013) Improving swamp water quality using ceramic membrane made from natural clay and coal fly ash. J Chem Eng 19(2):59–68
Google Scholar
Apriyanti E, Wijayanto W (2018) Coal ash based ceramic membrane application for clean water treatment. J Chem Eng 11(2):40–55
BLH profile. (2015). Monitoring the Water Quality of the Siak River and Siak Tributaries in Pekanbaru City. Pekanbaru: BLH
Chakraborty S, Uppaluri R, Das C (2020) Effect of pore former (saw dust) characteristics on the properties of sub-micron range low-cost ceramic membranes. Int J Ceram Eng Sci 2:243–253
Article CAS Google Scholar
Cheryan M (1998) Ultrafiltration and microfiltration handbook. lancashire technomic. CRC Press, Boca Raton
Book Google Scholar
Choi H, Zhang K, Dionysiou D, Oerther D, Sorial G (2005) Effect of permeate flux and tangential flow on membrane fouling for wastewater treatment. Sep Purif Technol 45:68–78
Furqoni RA, Mahardika P, Sulhadi. (2016) Development of water filters using ceramic materials to improve river water quality. Proc Natl Semin Phys 5(1):12–34
Hlordzi V, Kuebutornye FKA, Afriye G, Abarike EDA, Lu Y, Chi S, Anokyewaa MA (2020) The use of bacillus species in maintenance of water quality in aquaculture: a review. Aquac Rep 18(1):1–12
Huang J, Chen H, Yang J, Zhou T, Zhang H (2023) Effects of particle size on microstructure and mechanical strength of a fly ash based ceramic membrane. Ceram Int 49(10):15655–15664
Kutchko GB, Ann GK, Grim K (2006) Fly ash characterization by SEM–EDS. Fuel 85(17):54–87
Marsono BD, Agustina I, Yuniarto A, Hermana J (2022) Pressure and spacer effect on the performance of immersed microfiltration membrane. IOP Conf Ser Earth Environ Sci 1111:012063
Article Google Scholar
Mulder M (1991) Basic principles of membrane technology. Kluwer Academic Publishers, Netherland
Officer G (2014) Activated charcoal surface area analysis using the BET method (SAA). Diponegoro University, Semarang
Priyatno, D. (2009). SPSS for Correlation, Regression and Multivariate Analysis. Yogyakarta: Media Style
Puspayana D, Aliya D (2013) Tofu liquid waste processing using cross flow silica nanofiltration membrane to reduce nitrate and ammonium content. J Eng Pomits 2(2):6–10
PP RI Number 22 Years. (2021). Concerning the implementation of environmental protection and management. Jakarta: Ministry of Environment
PP RI Number 82 Years. (2001). Concerning water quality management and water pollution control. Jakarta: Ministry of Environment
Saifuddin S, Khairina K, Anwar F. (2018). Pembuatan Membran Keramik Sebagai Media Filter untuk Penyaringan Air PDAM. In: prosiding seminar nasional politeknik negeri lhokseumawe, vol 2 p 1
Sari SF, Sutrisno J, Dwi K (2012) Reduction of total coliform in groundwater using ceramic membrane. J Time Eng 16(1):30–38
Sobsey MD, Stauber CE, Casanova LM, Brown JM, Elliott MA (2008) Point of use household drinking water filtration: A practical, effective solution for providing sustained access to safe drinking water in the developing world. Environ Sci Technol 42(12):4261–4267
Soewignjo A, Nugroho P, Ningrum M (2020) Pemanfaatan geopolimer abu terbang sebagai pozzolanic tanah lempung untuk material tanah dasar perkerasan. Jurnal Fondasi 9(1):77–86
Sudiro and Suroto (2014) Effect of composition and size of briquette powder made from coal and rice straw on combustion characteristics. Indonusa Polytech Sci J 2(2):1–18
Wang YH, Zhang Y, Liu X, Meng GY (2006) Microstructure control of ceramic membrane support from corundum-rutile powder mixture. Powder Technol 168(2):125–133
Download references
Acknowledgements
The engineering faculty at the University of Riau is to be thanked for helping to make use of the Product Technology Laboratory for this project.
Author information
Authors and affiliations.
Chemical Engineering Product Technology Laboratory, Indonesia, Chemical Engineering Study Program, Faculty of Engineering, Riau University, Indonesia, Kampus Bina Widya Jl. HR. Soebrantas KM 12,5 Simpang Baru, Panam, Pekanbaru, 28293, Indonesia
I. Amri, K. H. Pratama, S. Arumnika & Z. Meldha
Department of Chemical & Process Engineering, Faculty of Engineering and Built Environment, Universiti Kebangsaan Malaysia, UKM, 43600, Bangi, Selangor, Malaysia
Department of Chemical Engineering, Faculty of Engineering, University of Riau, Kampus Binawidya, Jl. HR Subrantas KM 12,5 Panam, Pekanbaru, Riau, 28293, Indonesia
You can also search for this author in PubMed Google Scholar
Corresponding author
Correspondence to I. Amri .
Additional information
Editorial responsibility: S.Mirkia.
Rights and permissions
Springer Nature or its licensor (e.g. a society or other partner) holds exclusive rights to this article under a publishing agreement with the author(s) or other rightsholder(s); author self-archiving of the accepted manuscript version of this article is solely governed by the terms of such publishing agreement and applicable law.
Reprints and permissions
About this article
Amri, I., Pratama, K.H., Arumnika, S. et al. Ceramic membranes synthesized using fly ash pulp and paper boiler for COD and BOD removal from river. Int. J. Environ. Sci. Technol. (2024). https://doi.org/10.1007/s13762-024-05999-6
Download citation
Received : 06 July 2023
Revised : 16 July 2024
Accepted : 19 August 2024
Published : 13 September 2024
DOI : https://doi.org/10.1007/s13762-024-05999-6
Share this article
Anyone you share the following link with will be able to read this content:
Sorry, a shareable link is not currently available for this article.
Provided by the Springer Nature SharedIt content-sharing initiative
- Ceramic membrane
- Siak river water
- Find a journal
- Publish with us
- Track your research

IMAGES
VIDEO
COMMENTS
The papers in this issue are interesting and cover many aspects of this research topic, and will be meaningful for the sustainable drinking water quality protection.
Exposure to chemicals in drinking water may lead to a range of chronic diseases (e.g., cancer and cardiovascular disease), adverse reproductive outcomes and effects on children's health (e.g., neurodevelopment), among other health effects [3]. Although drinking water quality is regulated and monitored in many countries, increasing knowledge ...
Water is a vital natural resource for human survival as well as an efficient tool of economic development. Drinking water quality is a global issue, with contaminated unimproved water sources and inadequate sanitation practices causing human diseases (Gorchev & Ozolins, 1984; Prüss-Ustün et al., 2019).Approximately 2 billion people consume water that has been tainted with feces ().
Research and data on US drinking water contamination show that exposure profiles, health risks, and water quality reliability issues vary widely across populations, geographically and by contaminant.
This collection of papers—a collaboration between the WHO/UNICEF JMP and npj Clean Water—reviews emerging water quality data and explores exposure assessments, risk factors for contamination ...
Water quality has been linked to health outcomes across the world. This study evaluated the physico-chemical and bacteriological quality of drinking water supplied by the municipality from source ...
Quality management. Water treatment. The latest Intergovernmental Panel on Climate Change (IPCC) report reveals that climate change is widespread, rapid, and intensifying. (1) When considering the impacts of climate change, the focus is mostly on increasing temperatures, melting glaciers, or rising sea levels and the direct consequences.
A global survey report on "National Regulations and Standards For Drinking Water Quality" was prepared in 2018 which shows the data for 104 countries and territories (Fig. 1) up to the year 2015 [5].At the time of this survey, total population of these 104 countries and territories was approximately 6.5 billion people, representing approximately 89% of the world population.
In a special issue on "Drinking Water Quality and Human Health" IJERPH [5], 20 papers were recently published on different topics related to drinking water. Eight papers were on microbiological contamination, 11 papers on chemical contamination, and one on radioactivity. Five of the eight papers were on microbiology and the one on ...
Nowadays, declining water quality is a significant concern for the world because of rapid population growth, agricultural and industrial activity enhancement, global warming, and climate change influencing hydrological cycles. Assessing water quality becomes necessary by using a suitable method to reduce the risk of geochemical contaminants. Water's physical and chemical properties are ...
As shown in Fig. 1, the paper topics in this special issue dealt with "water, groundwater, health, risk, quality, drinking, spring…", which indicates that groundwater is the most important part of the water for drinking purpose, and health risk is closely related to the drinking water quality that is determined by many indices such as F ...
Background Water is the most abundant resource on earth, however water scarcity affects more than 40% of people worldwide. Access to safe drinking water is a basic human right and is a United Nations Sustainable Development Goal (SDG) 6. Globally, waterborne diseases such as cholera are responsible for over two million deaths annually. Cholera is a major cause of ill-health in Africa and ...
Using these two measures of poor water quality, we find 2.44% of community water systems, a total of 1165, were Safe Drinking Water Act Serious Violators and 3.37% of Clean Water Act permittees in ...
The quality of the water stored in households with continuous supply versus intermittent supply also suggests that water availability may play a role in household water quality. This is consistent with research that demonstrates that intermittent water supply introduces contamination into the distribution system in comparison with continuous ...
Water quality index (WQI) is one of the most used tools to describe water quality. It is based on physical, chemical, and biological factors that are combined into a single value that ranges from 0 to 100 and involves 4 processes: (1) parameter selection, (2) transformation of the raw data into common scale, (3) providing weights and (4) aggregation of sub-index values. The background of WQI ...
This paper provides new insight into the global landscape of water quality research in drinking water distribution systems and how it has evolved over the first twenty years of the 21st century. An up-to-date bibliometric analysis of relevant literature published between 2000 and 2020 revealed how the resear
Paper • The following article is Open access. The effect of drinking water quality on the health and longevity of people-A case study in Mayang, Hunan Province, China . J Lu 1 and F Yuan 1 ... Chen Y et al 1999 Elemental analysis of rocy soil and spring water in a Baizu Longevity area Trace Elements and Health Research 16 61-3. Google Scholar
Feature papers represent the most advanced research with significant potential for high impact in the field. A Feature Paper should be a substantial original Article that involves several techniques or approaches, provides an outlook for future research directions and describes possible research applications. ... Quality of drinking water is ...
In 2019, about 6% of public water utilities in the U.S. had a health-based violation. Due to the high risk of exposure to various contaminants in drinking water, point-of-use (POU) drinking water ...
contaminated water. The aim of this paper is to review water characteristics and its quality with the objectives of outlining the. implications associated with water quality parameters in excess ...
Background Water is a vital resource for human survival. Safe drinking water is a basic need for good health, and it is also a basic right of humans. The aim of this study was to analysis drinking water quality and its effect on communities residents of Wondo Genet. Result The mean turbidity value obtained for Wondo Genet Campus is (0.98 NTU), and the average temperature was approximately 28. ...
Introduction. Globally, 844 million people were still without access to a basic drinking water service in 2015 (WHO and UNICEF, Citation 2017) and more than 2 billion people reside in nations with high water stress (WWAP, Citation 2019).Growing water stress is a sign of intensive water resource usage, which has a negative impact on the sustainability of water supplies.
We recall that the initial Rapid Assessment of Drinking Water Quality (RADWQ) research, done in 2004/2005 15, created a platform for change that advanced a recognition of drinking water quality in ...
Industrial developments and settlements along the Siak River have affected the quality of the river water. Based on laboratory tests, the COD and BOD content of Siak River water still exceeds the standard set under Government Regulation No. 22 of 2021. This research was conducted to analyze the role of variations in the composition and size of materials of clay, fly ash, and sawdust, in ...