- Publications
- Conferences & Events
- Professional Learning
- Science Standards
- Awards & Competitions
- Daily Do Lesson Plans
- Free Resources
- American Rescue Plan
- For Preservice Teachers
- NCCSTS Case Collection
- Partner Jobs in Education
- Interactive eBooks+
- Digital Catalog
- Regional Product Representatives
- e-Newsletters
- Bestselling Books
- Latest Books
- Popular Book Series
- Prospective Authors
- Web Seminars
- Exhibits & Sponsorship
- Conference Reviewers
- National Conference • Denver 24
- Leaders Institute 2024
- National Conference • New Orleans 24
- Submit a Proposal
- Latest Resources
- Professional Learning Units & Courses
- For Districts
- Online Course Providers
- Schools & Districts
- College Professors & Students
- The Standards
- Teachers and Admin
- eCYBERMISSION
- Toshiba/NSTA ExploraVision
- Junior Science & Humanities Symposium
- Teaching Awards
- Climate Change
- Earth & Space Science
- New Science Teachers
- Early Childhood
- Middle School
- High School
- Postsecondary
- Informal Education
- Journal Articles
- Lesson Plans
- e-newsletters
- Science & Children
- Science Scope
- The Science Teacher
- Journal of College Sci. Teaching
- Connected Science Learning
- NSTA Reports
- Next-Gen Navigator
- Science Update
- Teacher Tip Tuesday
- Trans. Sci. Learning

MyNSTA Community
- My Collections
Diabetes and Insulin Signaling
By Kristy J. Wilson
Share Start a Discussion
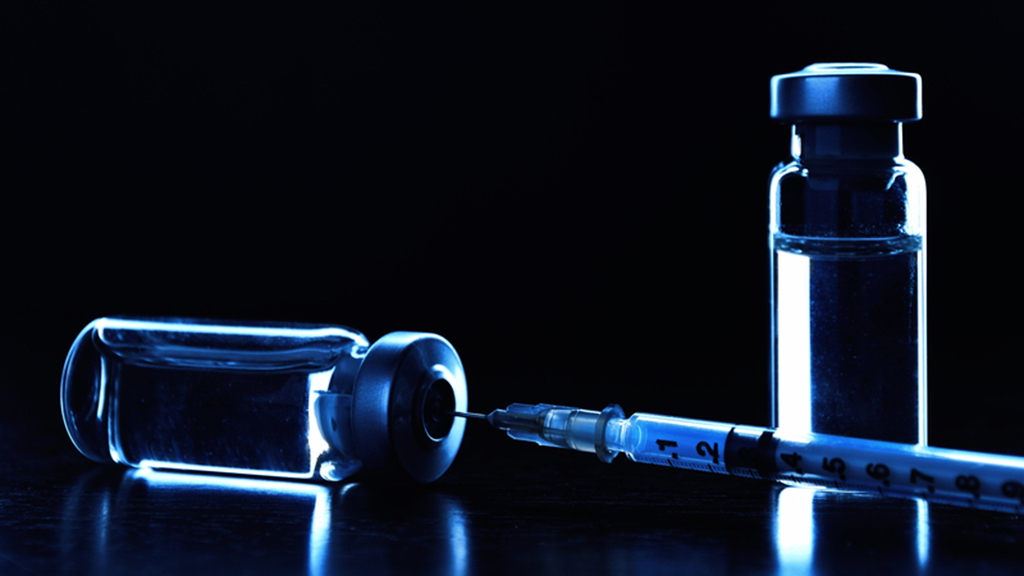
Cellular signaling, otherwise known as signal transduction, is the mechanism by which cellular context and environmental situation are used to regulate or adjust cellular behavior. Multicellular organisms use cellular signaling to coordinate responses to the environment, facilitate development, and maintain homeostasis. The mechanisms by which a cell receives a message and translates it into short-term or long-term effects are as varied as the responses stimulated by cellular signaling. There are, however, some generalized steps that can be applied to many different signaling situations. This case study uses insulin signaling and the pathological case of diabetes as a lens through which students will learn general signaling mechanisms like kinase cascades and second messenger pathways. The case is designed in the interrupted format and has three parts that could be used either as homework or as an in-class activity. It is written for use in a cell biology class for sophomore or junior undergraduates but could also be utilized in biochemistry, physiology, or genetics courses.
Download Case
Date Posted
- Divide a signaling pathway into generalized parts: signal, receptor, signaling effectors (i.e., signaling cascades or second messengers) and short-term/long-term effects.
- Explain the role of phosphorylation in protein/enzyme activity and how it contributes to signal transduction.
- Apply signal transduction mechanisms to diabetes's physiological symptoms.
- Hypothesize a mechanism to explain how signaling in diabetes might be specific for different tissue types.
- Design a line of inquiry or an experiment that could identify a cause of insulin resistance in type-2 diabetes.
Cellular signaling; signal transduction; insulin; insulin signaling; insulin resistance; diabetes; receptor; kinase; transcription factor; second messenger
Subject Headings
EDUCATIONAL LEVEL
Undergraduate lower division, Undergraduate upper division, Graduate
TOPICAL AREAS
TYPE/METHODS
Teaching Notes & Answer Key
Teaching notes.
Case teaching notes are protected and access to them is limited to paid subscribed instructors. To become a paid subscriber, purchase a subscription here .
Teaching notes are intended to help teachers select and adopt a case. They typically include a summary of the case, teaching objectives, information about the intended audience, details about how the case may be taught, and a list of references and resources.
Download Notes
Answer Keys are protected and access to them is limited to paid subscribed instructors. To become a paid subscriber, purchase a subscription here .
Download Answer Key
Materials & Media
Supplemental materials, you may also like.
Web Seminar
Join us on Thursday, June 13, 2024, from 7:00 PM to 8:00 PM ET, to learn about the science and technology of firefighting. Wildfires have become an e...
Join us on Thursday, October 10, 2024, from 7:00 to 8:00 PM ET, for a Science Update web seminar presented by NOAA about climate science and marine sa...

An official website of the United States government
The .gov means it’s official. Federal government websites often end in .gov or .mil. Before sharing sensitive information, make sure you’re on a federal government site.
The site is secure. The https:// ensures that you are connecting to the official website and that any information you provide is encrypted and transmitted securely.
- Publications
- Account settings
Preview improvements coming to the PMC website in October 2024. Learn More or Try it out now .
- Advanced Search
- Journal List
- Cold Spring Harb Perspect Biol
- v.6(1); 2014 Jan
Insulin Receptor Signaling in Normal and Insulin-Resistant States
Jérémie boucher.
1 Section on Integrative Physiology and Metabolism, Joslin Diabetes Center and Department of Medicine, Brigham and Women’s Hospital and Harvard Medical School, Boston, Massachusetts 02115
André Kleinridders
C. ronald kahn.
In the wake of the worldwide increase in type-2 diabetes, a major focus of research is understanding the signaling pathways impacting this disease. Insulin signaling regulates glucose, lipid, and energy homeostasis, predominantly via action on liver, skeletal muscle, and adipose tissue. Precise modulation of this pathway is vital for adaption as the individual moves from the fed to the fasted state. The positive and negative modulators acting on different steps of the signaling pathway, as well as the diversity of protein isoform interaction, ensure a proper and coordinated biological response to insulin in different tissues. Whereas genetic mutations are causes of rare and severe insulin resistance, obesity can lead to insulin resistance through a variety of mechanisms. Understanding these pathways is essential for development of new drugs to treat diabetes, metabolic syndrome, and their complications.
Insulin and IGF-1 act via tyrosine kinase receptors to produce signals that control biological processes. The signaling pathways are precisely regulated, and perturbations in them may lead to insulin resistance.
Insulin and IGF-1 control a wide variety of biological processes by acting on two closely related tyrosine kinase receptors. Receptor activation initiates a cascade of phosphorylation events that leads to the activation of enzymes that control many aspects of metabolism and growth. Insulin/IGF-1 signaling contains many different points of regulation or critical nodes, controlled both positively and negatively, to ensure proper signal duration and intensity (see the schematic in Fig. 1 ). Perturbations in these signaling pathways can lead to insulin resistance. Here we review the insulin-signaling network, its critical nodes, and how these are perturbed in insulin-resistant states.

Insulin- and IGF-1-signaling pathways. Activation of insulin and IGF-1 receptors by their ligands initiates a cascade of phosphorylation events. A conformational change and autophosphorylation of the receptors occur at the time of ligand binding, leading to the recruitment and phosphorylation of receptor substrates such as IRS and Shc proteins. Shc activates the Ras-MAPK pathway, whereas IRS proteins mostly activate the PI3K-Akt pathway by recruiting and activating PI3K, leading to the generation of second messenger PIP 3 . Membrane-bound PIP 3 recruits and activates PDK-1, which phosphorylates and activates Akt and atypical PKCs. Akt mediates most of insulin's metabolic effects, regulating glucose transport, lipid synthesis, gluconeogenesis, and glycogen synthesis. Akt also plays a role in the control of cell cycle and survival. The Shc-Grb2-Sos-Ras-Raf-MAPK pathway controls cellular proliferation and gene transcription.
INSULIN AND IGF-1 RECEPTORS
Insulin and IGF-1 mediate their biological effects via the insulin and IGF-1 receptors (IR and IGF-1R). These highly homologous tyrosine kinase receptors are members of a family that also includes the orphan insulin receptor-related receptor (IRR), which has been suggested to play a role in testis determination ( Nef et al. 2003 ) and act as an extracellular alkali sensor ( Deyev et al. 2011 ). Although insulin and IGF-1 preferentially bind to their own receptors, both ligands can also bind to the alternate receptor with reduced affinity ( Belfiore et al. 2009 ).
The IR, IGF-1R, and IRR are tetrameric proteins that consist of two extracellular α subunits and two transmembrane β subunits that are joined by disulfide bonds. Both subunits are generated from a single large precursor by proteolytic cleavage. The IR messenger RNA (mRNA) undergoes alternative splicing of exon 11 to yield two isoforms that differ by exclusion (isoform A) or inclusion (isoform B) of a 12- amino-acid sequence in the carboxy-terminal part of the α subunit ( Mosthaf et al. 1990 ). IR-A is predominantly expressed in fetal tissues and in the brain, has higher affinity for both insulin and IGF-2, has a higher rate of internalization than the type-B isoform, and tends to be up-regulated in cancer ( Frasca et al. 1999 ), whereas IR-B expression is highest in the liver. Heterotetramers composed of an α/β dimer of IR and an α/β dimer of IGF-1R can form hybrid receptor complexes that bind preferentially IGF-1 and IGF-2 over insulin ( Benyoucef et al. 2007 ). Their formation appears to occur randomly in cells expressing both receptors and depends on the relative expression level of each type of receptor ( Bailyes et al. 1997 ; Pandini et al. 1999 ). Insulin and IGF-1 differential effects in vivo reflect mostly the hormone concentration and relative expression level of receptors in different tissues rather than the capacity of IR and IGF-1R to convey different signaling ( Boucher et al. 2010 ; Siddle 2012 ).
INSULIN RECEPTOR SUBSTRATES
At the time of ligand binding to the α subunits, IR and IGF-1R undergo a conformational change-inducing activation of the kinase activity in the β subunits. This results in transphosphorylation among β subunits, further activating the kinase and allowing the recruitment of receptor substrates. The best characterized substrates are members of the insulin receptor substrate (IRS) family of proteins, simply referred to as IRS-1 through IRS-6, which act as scaffolds to organize and mediate signaling complexes ( Sun et al. 1991 , 1995 ; Lavan et al. 1997a , b ; Cai et al. 2003 ; White 2006 ; Shaw 2011 ). IRS proteins are recruited to the membrane and the activated receptors through both pleckstrin homology (PH) and phosphotyrosine binding (PTB) domains in their amino terminus ( Voliovitch et al. 1995 ). They are subsequently phosphorylated by the activated receptors on multiple tyrosine residues that form binding sites for intracellular molecules that contain Src-homology 2 (SH2) domains ( Sun et al. 1993 ).
Although these substrates have similar tyrosine phosphorylation motifs, they clearly have different functions in vivo. IRS-1 knockout (KO) mice show growth retardation and impaired insulin action, especially in muscle ( Araki et al. 1994 ), but have normal glucose tolerance. IRS-2 KO mice display growth reduction in only selective tissues, such as certain neurons and islet cells, but also have defective insulin signaling in the liver, which when combined with the loss of β cells results in the development of diabetes ( Withers et al. 1998 ). At the cellular level, IRS-1 KO preadipocytes show defects in differentiation, whereas IRS-2 KO preadipocytes differentiate normally, but have impaired insulin-stimulated glucose transport ( Miki et al. 2001 ; Tseng et al. 2005 ). In skeletal muscle cells, IRS-1, but not IRS-2, is required for myoblast differentiation and glucose metabolism, whereas IRS-2 is important for lipid metabolism and ERK activation ( Huang et al. 2005 ; Bouzakri et al. 2006 ).
IRS-3 and IRS-4 show a more restricted tissue distribution pattern. In rodents, IRS-3 is most abundant in adipocytes, liver, and lung ( Sciacchitano and Taylor 1997 ), whereas in humans, the IRS-3 gene is a pseudogene, so no protein is produced at all ( Bjornholm et al. 2002 ). In mice, disruption of the gene for IRS-3 alone does not result in abnormalities, but leads to a severe defect in adipogenesis when combined with deletion of IRS-1 ( Laustsen et al. 2002 ). IRS-4 mRNA is present in skeletal muscle, liver, heart, brain, and kidney ( Fantin et al. 1999 ), and IRS-4 KO mice show only very minimal growth retardation and glucose intolerance ( Fantin et al. 2000 ). IRS-5 (also called DOK4) and IRS-6 (DOK5) have limited tissue expression ( Cai et al. 2003 ) and are relatively poor IR substrates ( Versteyhe et al. 2010 ).
In addition to the IRS proteins, the insulin and IGF-1 receptors can phosphorylate several other substrates ( Siddle 2012 ). Shc proteins are tyrosine phosphorylated by IR and IGF-1R, and participate in the activation of the Ras/ERK pathway. Grb2-associated binder (GAB) proteins are also substrates for a variety of receptors, including IR and IGF-1R. GAB proteins resemble IRS proteins, but lack a protein tyrosine phosphatase (PTP) domain, and could play a role in insulin/IGF-1 signaling in cells expressing low IRS protein levels. APS (SHB2) and Cbl are IR/IGF-1R substrates that recruit other proteins, such as the Cbl-associated protein (CAP), to the insulin-signaling complex. The latter participates in the control of insulin-stimulated glucose uptake ( Baumann et al. 2000 ). SH2B1 directly binds to insulin receptors and IRS proteins and enhances insulin sensitivity by promoting insulin receptor catalytic activity and by inhibiting tyrosine dephosphorylation of IRS proteins.
PHOSPHATIDYLINOSITOL (3,4,5)-TRIPHOSPHATE AND PHOSPHOINOSITIDE 3-KINASE
The critical pathway linking IRS proteins to the metabolic actions of insulin is the PI3-kinase (PI3K) and Akt pathway. The class Ia PI3-kinases are heterodimers consisting of a regulatory and catalytic subunit, each of which occurs in several isoforms ( Vadas et al. 2011 ). Recruitment and activation of the PI3K depends on the binding of the two SH2 domains in the regulatory subunits to tyrosine-phosphorylated IRS proteins ( Myers et al. 1992 ; Shaw 2011 ). This results in activation of the catalytic subunit, which rapidly phosphorylates phosphatidylinositol 4,5-bisphosphate (PIP 2 ) to generate the lipid second messenger phosphatidylinositol (3,4,5)-triphosphate (PIP 3 ). The latter recruits Akt to the plasma membrane, where it is activated by phosphorylation and induces downstream signaling.
The different isoforms of the regulatory subunit of PI3K are encoded by three distinct genes. Pik3r1 encodes 65%–75% of all regulatory subunits, mostly in the form of p85α, but also the splice variants p55α and p50α. Pik3r2 encodes p85β and accounts for ∼20% of the regulatory subunits. Pik3r3 encodes p55γ, which is similar in structure to p55α, but expressed at low levels in most tissues.
The three different catalytic subunits—p110α, β, and δ—are derived from three different genes. Binding of a regulatory to a catalytic subunit increases the catalytic subunit stability and maintains it in an inhibited state. This is relieved by binding of the regulatory subunit to specific phosphotyrosine motifs in IRS proteins, resulting in its activation ( Yu et al. 1998 ; Burke et al. 2011 ; Zhang et al. 2011 ). Liver-specific ablation of p110α, and to a lesser extent p110β, in mice results in glucose intolerance and insulin resistance ( Jia et al. 2008 ; Sopasakis et al. 2010 ). Surprisingly, knockouts of the regulatory subunits of PI3K, including a heterozygous deletion of p85α, p85β KO, or p50α/p55α double KO, all display increased insulin sensitivity ( Terauchi et al. 1999 ; Ueki et al. 2002 ). Different mechanisms by which reducing concentration of regulatory subunits can increase insulin action have been identified. Regulatory subunits typically are in excess concentration to catalytic subunits and thus compete with the enzymatically competent p85/p110 heterodimer for binding to IRS proteins. The p85α monomer has also been linked to regulation of the phosphatase and tensin homolog (PTEN) ( Taniguchi et al. 2010 ). More recently, p85α has been shown to bind to the transcription factor XBP-1 and to modify the unfolded protein response, which contributes to insulin resistance ( Park et al. 2010 ; Winnay et al. 2010 ).
In addition to PI3K, IRS proteins recruit other proteins potentially contributing to insulin and IGF-1 action. Proteomics analysis of the phosphotyrosine interactome of IRS-1 and IRS-2 indicates that most interacting proteins bind to both substrates, such as adaptor proteins Grb2 or Crk, or tyrosine phosphatase SHP2. However, other interaction partners seem to bind exclusively to IRS-1 (Csk) or IRS-2 (Shc, DOCK-6, and DOCK-7) ( Hanke and Mann 2009 ).
ACTIVATION OF DOWNSTREAM KINASES
Most of the physiological effects of PI3K-generated PIP 3 are mediated by a subset of AGC protein kinase family members, which include isoforms of Akt/protein kinase B (PKB), p70 ribosomal S6 kinase (S6K), serum- and glucocorticoid-induced protein kinase (SGK), as well as several isoforms of protein kinase C (PKC), particularly the atypical PKCs. AGC kinase family members share similar structure and mechanisms of activation via phosphorylation of two serine and threonine residues ( Pearce et al. 2010 ). PDK-1 (3-phosphoinositide-dependent protein kinase 1) is the major upstream kinase responsible for the phosphorylation and activation of the AGC kinase members regulated by PI3K ( Bayascas 2010 ). PDK-1 contains a PH domain that binds to membrane-bound PIP 3 , triggering PDK-1 activation. PDK-1 phosphorylates and activates AGC protein kinases at serine/threonine residues, such as Thr-308 for Akt ( Alessi et al. 1997 ). However, Akt phosphorylation at Ser-473 is required for full activation, and this is accomplished by the mammalian target of rapamycin complex 2 (mTORC2) ( Sarbassov et al. 2005 ; Oh and Jacinto 2011 ). DNA-dependent protein kinase (DNA PK) has also been described to phosphorylate and activate Akt in response to DNA damage ( Bozulic et al. 2008 ), and is involved in insulin regulation of metabolic genes such as fatty acid synthase ( Wong et al. 2009 ).
The Akt/PKB family of proteins consists of three different isoforms of serine/threonine protein kinases encoded by different genes ( Schultze et al. 2011 ). All isoforms possess a PH domain, allowing interaction with PIP 3 and recruitment to the plasma membrane. Akt2 is most abundant in insulin-sensitive tissues and seems to play a predominant role in mediating insulin action on metabolism. Thus, Akt2 KO mice are insulin resistant and develop diabetes ( Cho et al. 2001 ), whereas Akt1 and Akt3 KO mice do not.
ACTIONS OF INSULIN DOWNSTREAM FROM AKT
Activation of Akt by PDK-1 and mTORC2 allows the phosphorylation and activation of many downstream targets. Akt phosphorylates tuberous sclerosis complex protein 2 (TSC-2), inducing the degradation of the tumor suppressor complex that consists of TSC-2 and TSC-1, which activates the mTORC1 complex. Akt-induced activation of mTORC1 can also be achieved by phosphorylation of proline-rich Akt substrate 40 KDa (PRAS40), an inhibitor of mTORC1, thereby relieving the inhibition. The mTORC1 complex then phosphorylates and inhibits 4E-binding protein 1 (4E-BP1), activates ribosomal protein S6 kinases S6K1 and S6K2 and SREBP1, and leads to the regulation of a network of genes controlling metabolism, protein synthesis, and cell growth ( Duvel et al. 2010 ).
Transcription factors of the Forkhead box O (Foxo) family control the expression of lipogenic and gluconeogenic genes. Akt phosphorylates Foxos at several sites which provides docking sites for binding proteins of the 14-3-3 family. This interaction leads to the exclusion of Foxo from the nucleus, thus blocking its transcriptional activity ( Tzivion et al. 2011 ). Interestingly, although mice lacking Akt1 and Akt2 show severe hepatic insulin resistance and high levels of hepatic glucose production, these defects are normalized when Foxo1 is concomitantly ablated in the liver. This indicates that an additional pathway exists in the control of hepatic glucose metabolism beyond the Akt/Foxo1 axis, which allows for insulin-mediated regulation of hepatic glucose production ( Lu et al. 2012 ).
There are multiple other substrates of Akt involved in insulin action. The GTPase-activating protein Akt substrate of 160 kDa (AS160), also called TBC1D4, and its homolog TBC1D1, are phosphorylated by Akt and are involved in insulin- and contraction-mediated glucose uptake ( Sano et al. 2003 ; Sakamoto and Holman 2008 ; Taylor et al. 2008 ; An et al. 2010 ). Akt also phosphorylates and inactivates glycogen synthase kinase 3, resulting in glycogen synthase activation and glycogen accumulation in liver ( Kim et al. 2004b ). Akt-dependent phosphorylation of PGC-1α impairs the ability of PGC-1α to promote gluconeogenesis and fatty acid oxidation ( Li et al. 2007 ). Phosphorylation of phosphodiesterase 3B (PDE3B) by Akt results in its activation and in a decrease in cyclic AMP levels ( Kitamura et al. 1999 ), which plays important roles in the effect of insulin to inhibit lipolysis in adipocytes and insulin secretion in β cells ( Degerman et al. 2011 ).
OTHER ACTIONS OF INSULIN DOWNSTREAM FROM PI3K
Akt plays a central role in mediating many other insulin actions by regulating the expression and activity of a wide range of proteins, including enzymes, transcription factors, cell cycle regulating proteins, or apoptosis and survival proteins ( Manning and Cantley 2007 ). Murine double minute 2 (Mdm2) is phosphorylated by Akt, which inhibits p53-mediated apoptosis and contributes to tumorigenesis ( Cheng et al. 2010 ). Akt phosphorylates cell cycle inhibitors p21Cip1/WAF1 and p27Kip1, resulting in cytoplasmic localization, cell growth, and inhibition of apoptosis ( Zhou et al. 2001 ; Motti et al. 2004 ). Akt also phosphorylates and inhibits Bax, Bad, and caspase-9, which promotes cell survival ( Datta et al. 1997 ; Cardone et al. 1998 ; Yamaguchi and Wang 2001 ; Gardai et al. 2004 ). Akt can phosphorylate and activate IkB kinase (IKK), leading to NF-κB activation ( Bai et al. 2009 ). Akt phosphorylates and activates endothelial nitric oxide synthase (eNOS), which catalyzes the production of the vasodilator and anti-inflammatory molecule nitric oxide (NO), providing a potential link between insulin resistance and cardiovascular disease ( Dimmeler et al. 1999 ; Fulton et al. 1999 ; Yu et al. 2011a ). Although less well studied in insulin action, the serum- and glucocorticoid-induced protein family of kinases (SGK) are highly homologous to Akt, are also activated by dual phosphorylation by PDK-1 and mTORC2 in a PI3K dependent manner, and have many downstream substrates in common with Akt ( Bruhn et al. 2010 ).
PROTEIN KINASES C
PKC isoforms are both mediators and modifiers of insulin's metabolic action. Of the three major classes of PKC, the atypical PKCs (aPKCs), PKC-ζ and PKC-λ/ι, are activated via phosphorylation by PDK-1. aPKCs play an important role in insulin-stimulated glucose transport and regulation of lipid synthesis, and their expression and/or activation is decreased in muscle from obese and diabetic humans ( Farese and Sajan 2010 ). Both PKC-λ and PKC-ζ have been shown to function interchangeably in mediating insulin-stimulated glucose transport ( Sajan et al. 2006 ). Muscle-specific deletion of PKC-λ in mice leads to impairment in insulin-induced glucose uptake and insulin resistance ( Farese et al. 2007 ). Mice with liver-specific deletion of PKC-λ display decreased insulin-induced expression of SREBP1c and triglyceride content in the liver, resulting in increased insulin sensitivity in these mice ( Matsumoto et al. 2003 ).
THE GRB2-SOS-RAS-MAPK PATHWAY
A second essential branch of the insulin/IGF-1-signaling pathway is the Grb2-SOS-Ras-MAPK pathway, which is activated independently of PI3K/Akt. Activated receptors and IRS proteins both possess docking sites for adaptor molecules that contain SH2 domains such as Grb2 and Shc. The carboxy-terminal SH3 domain of Grb2 binds to proteins such as Gab-1, whereas the amino-terminal SH3 domain binds to proline-rich regions of proteins such as son-of-sevenless (SOS). SOS is a guanine nucleotide exchange factor (GEF) for Ras, catalyzing the switch of membrane-bound Ras from an inactive, GDP-bound form (Ras-GDP) to an active, GTP-bound form (Ras-GTP). Ras-GTP then interacts with and stimulates downstream effectors, such as the Ser/Thr kinase Raf, which stimulates its downstream target MEK1 and 2 that phosphorylate and activate the MAP kinases ERK1 and 2. Stimulated ERK1/2 play a direct role in cell proliferation or differentiation, regulating gene expression or extra-nuclear events, such as cytoskeletal reorganization, through phosphorylation and activation of targets in the cytosol and nucleus.
NEGATIVE REGULATORS OF INSULIN SIGNALING
Insulin and IGF-1 signaling are tightly controlled because uncontrolled activity of the downstream pathways could lead to severe perturbations in metabolism and tumorigenesis. Intensity and duration of the signal play an important role in determining the specificity of the response to their pleiotropic effects. Therefore, the ability to turn off the insulin signal in a rapid manner at different levels is critical ( Fig. 2 ). On the other hand, some of these inhibitory mechanisms can be altered in pathophysiological conditions and participate in the development of insulin resistance.

Negative modulators of insulin and IGF-1 signaling. Intensity and duration of insulin and IGF-1 signaling play an important role in determining the specificity and the nature of the response to these hormones. Signaling is attenuated by action of several phosphatases, which dephosphorylate the receptors, IRS proteins, PKCs, and ERK or PIP 3 . In addition, stress kinases such as JNK, IKK, and ERK, as well as PKCs or S6K, inhibit insulin/IGF-1 signaling by inducing inhibitory serine/threonine phosphorylation of IR/IGFR and IRS proteins. Trb3 inhibits Akt, and adaptor proteins such as SOCS and Grb bind to the receptors and IRS proteins and inhibit signaling by competition.
Phosphoprotein Phosphatases as Negative Regulators of Insulin Action
Both cytoplasmic protein tyrosine phosphatases, such as PTP1B, and transmembrane phosphatases, such as LAR, have been shown to dephosphorylate the tyrosine residues on activated IR and IGF-1R, as well as IRS proteins, thereby reducing their activity ( Goldstein et al. 1998 ). Although the role of LAR in the control of insulin signaling in vivo remains controversial, PTP1B is an essential component of insulin action. PTP1B KO mice show enhanced insulin sensitivity, increased IR phosphorylation in muscle and liver, and are also resistant to high-fat-diet-induced obesity and associated insulin resistance ( Elchebly et al. 1999 ; Klaman et al. 2000 ).
The serine/threonine phosphatase protein phosphatase 1 (PP1) has been implicated in the regulation of several rate-limiting enzymes in both glucose and lipid metabolism, including glycogen synthase, hormone-sensitive lipase, or acetyl CoA carboxylase ( Brady and Saltiel 2001 ). Protein phosphatase 2A (PP2A), which accounts for ∼80% of serine/threonine phosphatase activity in cells, also regulates the activities of many protein kinases involved in insulin action, including Akt, PKC, S6K, ERK, cyclin-dependent kinases, and IKK ( Millward et al. 1999 ). Several studies indicate that PP2A is hyperactivated in diabetic states ( Kowluru and Matti 2012 ).
Other serine/threonine phosphatases have been implicated in insulin action. Protein phosphatases 2B (PP2B), also known as calcineurin, has been shown to dephosphorylate Akt ( Ni et al. 2007 ). Two novel members of the PP2C family involved in regulation of insulin action are the PH domain leucine-rich repeat protein phosphatases PHLPP-1 and -2, which dephosphorylate both Akt and PKCs ( Brognard and Newton 2008 ). Overexpression of PHLPP1 in cells impairs Akt and glycogen synthase kinase 3 activity, resulting in decreased glycogen synthesis and glucose transport ( Andreozzi et al. 2011 ). Elevated levels of PHLPP1 have been found in adipose tissue and skeletal muscle of obese and/or diabetic patients and correlate with decreased Akt2 phosphorylation ( Cozzone et al. 2008 ; Andreozzi et al. 2011 ).
Lipid Phosphatases as Negative Regulators of Insulin Action
Lipid phosphatases can regulate insulin signaling by modulating PIP 3 levels. PTEN dephosphorylates PIP 3 , thus antagonizing PI3K signaling in cells ( Cantley and Neel 1999 ; Carracedo and Pandolfi 2008 ). Muscle, adipose tissue, or liver-specific deletion of PTEN in mice increases insulin sensitivity ( Stiles et al. 2004 ; Kurlawalla-Martinez et al. 2005 ; Wijesekara et al. 2005 ), and mice with whole-body PTEN haploinsufficiency show improved glucose tolerance and increased insulin sensitivity ( Wong et al. 2007 ). Interestingly, the p85α regulatory subunit of PI3K has recently been shown to bind directly to and enhance PTEN activity, creating a unique interface between the generation and degradation of PIP 3 ( Taniguchi et al. 2006b ; Chagpar et al. 2010 ).
SH2 domain-containing inositol 5-phosphatases (SHIP) 1 and 2 also dephosphorylate PIP 3 . SHIP1 expression is restricted to hematopoietic cells, whereas SHIP2 is ubiquitously expressed and plays a role in insulin signaling ( Suwa et al. 2010 ). SHIP2 deficiency in mice results in hypoglycemia, enhanced insulin-induced Akt activation, and resistance to high-fat-diet-induced obesity, indicating that SHIP2 is a key regulator of glucose and energy homeostasis in vivo ( Clement et al. 2001 ; Sleeman et al. 2005 ). Conversely, SHIP2-overexpressing mice show reduced insulin-induced Akt activation in the liver, fat, and skeletal muscle ( Kagawa et al. 2008 ).
Other Negative Modulators (Grb, SOCS, Trb3, IP7)
Grb10 and Grb14 are cytoplasmic adaptor proteins that decrease IR and to a lesser extent IGF-1R activity, and prevent access of substrates to the activated receptors ( Holt and Siddle 2005 ). Deletion of the Grb10 gene in mice leads to increased growth, enhanced insulin signaling, and increased glucose tolerance ( Smith et al. 2007 ; Wang et al. 2007 ). Grb10 overexpression, on the other hand, results in impaired growth, glucose intolerance, and insulin resistance ( Shiura et al. 2005 ). Grb14 expression is increased in adipose tissue of insulin-resistant animal models and type-2 diabetic patients ( Cariou et al. 2004 ), and Grb14 KO mice display increased glucose tolerance and insulin sensitivity, consistent with an inhibitory role of Grb14 on insulin signaling ( Cooney et al. 2004 ). Grb10 and Grb14 share similar mechanisms as insulin signaling is not further increased in mice with deletion of both proteins ( Holt et al. 2009 ).
Proteins of the suppressor of cytokine signaling (SOCS) family are adaptor proteins that act as negative regulators of cytokine and growth factor signaling. In addition, SOCS proteins, in particular SOCS1 and SOCS3, negatively regulate insulin signaling and thus link cytokine signaling to insulin resistance. Their expression is increased in obesity, and they induce insulin resistance via either inhibition of the tyrosine kinase activity of the IR, competition for binding of the IRS proteins to the receptor, or targeting the IRS proteins to degradation ( Emanuelli et al. 2000 , 2001 ; Rui et al. 2002 ; Ueki et al. 2004a , b ; Sachithanandan et al. 2010 ).
Tribbles homolog 3 (Trb3) is a member of the family of pseudokinases that is thought to function as adaptor proteins. Trb3 expression is induced in liver in fasting and diabetes, and disrupts insulin signaling by binding to Akt and blocking its activation. Trb3 knockdown in mice improves glucose tolerance ( Du et al. 2003 ; Koo et al. 2004 ). In cultured cells, insulin-stimulated S6K activation is decreased when Trb3 is overexpressed, and increased when Trb3 levels are reduced ( Matsushima et al. 2006 ). Trb3 action in adipose tissue seems to be independent of Akt. Thus, whereas insulin promotes lipogenesis, Trb3 stimulates lipolysis by triggering the ubiquitination and degradation of acetyl-CoA carboxylase. Transgenic mice overexpressing Trb3 in adipose tissue are protected from diet-induced obesity because of enhanced fatty acid oxidation and display increased insulin sensitivity ( Qi et al. 2006 ).
A novel negative regulator of insulin signaling is the inositol phosphate IP7. It was recently shown that insulin and IGF-1 increase IP7 levels, which in turn inhibits Akt translocation to the plasma membrane and subsequent activation, creating a potential feedback mechanism that attenuates insulin signaling ( Chakraborty et al. 2010 ). Deletion of the enzyme that catalyzes IP7 formation in mice causes increased insulin responsiveness. Further studies will be needed to elucidate the contribution of this pathway in normal or pathological conditions.
Regulation by Inhibitory Serine and Threonine Phosphorylation
Tyrosine phosphorylation is essential for IR/IGF-1R and IRS activation. On the other hand, serine and threonine phosphorylation of the receptors or IRS proteins is primarily involved in turning the insulin signal down ( Fig. 3 ). Increased inhibitory Ser/Thr phosphorylation of IR and especially IRS-1 and -2 occurs in response to cytokines, fatty acids, hyperglycemia, mitochondrial dysfunction, and ER stress, and insulin itself via activation of multiple kinases, predominantly by c-Jun amino-terminal kinase (JNK), IKK, conventional and novel PKCs, but also mTORC1/S6K and MAPK ( De Fea and Roth 1997a ; Aguirre et al. 2000 ; Gao et al. 2002 ; Gual et al. 2003 ; Li et al. 2004 ; Zhang et al. 2008a ; Boura-Halfon and Zick 2009 ). Increased IR serine phosphorylation associated with decreased tyrosine kinase activity has been observed in insulin-resistant states, both in rodents and in humans ( Karasik et al. 1990 ; Dunaif et al. 1995 ; Zhou et al. 1999 ; Shao et al. 2000 ). An increase in cAMP concentration also induces inhibitory serine phosphorylation of IR in a PKA-dependent manner ( Stadtmauer and Rosen 1986 ; Roth and Beaudoin 1987 ).

Activation of Ser/Thr kinases causes inhibitory phosphorylation on insulin-signaling molecules. Lipotoxicity, inflammation, hyperglycemia, and subsequently oxidative stress, as well as mitochondrial dysfunction and ER stress, all converge on activation of Ser/Thr kinases, inducing inhibitory Ser/Thr phosphorylation of IR, IRS proteins, and Akt on multiple residues, causing insulin resistance.
Although inhibitory IRS-1 serine phosphorylation occurs at many different sites ( Boura-Halfon and Zick 2009 ), the best studied of these modifications occurs at Ser-307 ( Aguirre et al. 2002 ). IRS-1 Ser-307 phosphorylation is increased in obese and diabetic mice ( Hirosumi et al. 2002 ; Um et al. 2004 ). Although this is widely believed to contribute to insulin resistance by inhibiting insulin receptor kinase activity, recent studies have made this association less clear. Thus, insulin itself can stimulate phosphorylation of IRS-1 on Ser-307 in humans ( Yi et al. 2007 ), and mice with a knock-in of IRS-1 Ser307Ala mutant developed more severe insulin resistance than control mice when fed a high-fat diet, indicating that Ser-307 is required to maintain normal insulin signaling ( Copps et al. 2010 ). Thus, increased IRS-1 Ser-307 phosphorylation observed in insulin-resistance states may be associated with, but not cause, insulin resistance.
Lipids, through their metabolic product diacylglycerols, can activate classical (α, β, γ) and novel PKC members (δ, θ, ε) and impair insulin signaling by inducing multiple serine phosphorylation of IRS proteins and IR specifically at Thr-1336, Thr-1348, and Ser-1305/1306 ( Bollag et al. 1986 ; Karasik et al. 1990 ; Lewis et al. 1990 ; Chin et al. 1993 ; De Fea and Roth 1997b ; Turban and Hajduch 2011 ). Thus, deletion of any member of the novel PKC family prevents the development of insulin resistance in skeletal muscle and liver by decreasing IRS-1 Ser-307 phosphorylation ( Kim et al. 2004a ; Samuel et al. 2007 ; Mack et al. 2008 ; Bezy et al. 2011 ). Atypical PKC-ζ also inhibits insulin signaling by inducing serine phosphorylation of IRS-1 ( Ravichandran et al. 2001 ) and Thr-34 phosphorylation of Akt, thereby inhibiting its recruitment to the plasma membrane ( Powell et al. 2003 , 2004 ).
Another component of the negative feedback loops in insulin signaling is mTORC1. Activation of mTOR and S6K is not only downstream from insulin signaling, but also inhibits it by increasing serine phosphorylation and reducing IRS tyrosine phosphorylation. This is illustrated by the phenotype of S6K null mice, which are lean and display enhanced insulin sensitivity ( Um et al. 2004 ). In addition, IRS-1 is hyperphosphorylated and degraded in TSC-2 KO fibroblasts, which show constitutive S6K activation ( Harrington et al. 2004 ; Shah et al. 2004 ). mTORC1 also mediates phosphorylation and stabilization of Grb10, leading to feedback inhibition of insulin signaling ( Hsu et al. 2011 ; Yu et al. 2011b ).
MECHANISMS OF INSULIN RESISTANCE
A central feature of type-2 diabetes is insulin resistance, a condition in which cells cannot respond properly to insulin. This occurs primarily at the level of so-called insulin-sensitive tissues, such as liver, muscle, and fat, and can be caused by multiple mechanisms ( Fig. 3 and Table 1 ).
Molecular mechanisms of insulin resistance
Multiple molecular mechanisms of insulin resistance have been described, in addition to inhibitory Ser/Thr phosphorylation on insulin-signaling molecules ( Fig. 3 ). Genetic mutations, dephosphorylation events, posttranslational modifications, and formation of inhibitory complexes have all been shown to cause insulin resistance.
Genetic Causes of Insulin Resistance
Insulin receptor.
Mutations in the insulin receptor gene have been identified in several rare forms of severe insulin resistance, including leprechaunism, Rabson-Mendenhall syndrome, or the type-A syndrome of insulin resistance. These patients often require a hundredfold or more insulin than a typical diabetic patient ( Kahn et al. 1976 ; Cochran et al. 2005 ). Most of these patients have nonsense or missense mutations in the extracellular ligand-binding domain or intracellular tyrosine kinase domain of the receptor, which leads to severely reduced insulin binding, altered kinetics of insulin binding, or reduced tyrosine kinase activity, but some also have presumed promoter defects leading to reduced receptor mRNA expression ( Taylor et al. 1991 ; Haruta et al. 1995 ). Insulin receptor mutations have not been observed in patients with routine type-2 diabetes (T2D).
Insulin Receptor Substrate Proteins
The G972R polymorphism of IRS-1 is observed with higher frequency in patients with T2D and leads to decreased insulin signaling, mostly decreasing PI3K activity ( Almind et al. 1996 ; Hribal et al. 2008 ). Although this finding has not been confirmed in all large-scale population analyses ( Florez et al. 2004 ; van Dam et al. 2004 ), recent studies have continued to show an association between a single-nucleotide polymorphism (SNP) in IRS-1 and T2D ( Burguete-Garcia et al. 2010 ; Martinez-Gomez et al. 2011 ). A T608R missense mutation in IRS-1 resulting in decreased insulin signaling has been reported in a patient with T2D, but appears to be very rare ( Esposito et al. 2003 ). Numerous polymorphisms have been identified in the human IRS-2 gene, but a clear association between these polymorphisms and T2D has not been found ( Bernal et al. 1998 ).
Phosphoinositide 3-Kinase
An M326I polymorphism in the p85α regulatory subunit of the PI3K was identified in Pima Indian women and is associated with decreased prevalence for T2D ( Baier et al. 1998 ). However, this M326I mutation only has modest effects on insulin signaling in vitro by decreasing p85α binding to IRS-1 and increasing p85α degradation ( Almind et al. 2002 ). Another polymorphism in p85α (SNP42) is associated with fasting hyperglycemia, but its molecular mechanism so far remains elusive ( Barroso et al. 2003 ).
Phosphatase and Tensin Homolog
In diabetes, mutations of PTEN have not been reported yet. However, three Japanese type-2 diabetic subjects have been identified with polymorphisms in the PTEN gene, one of which was associated with T2D. This SNP caused a higher expression rate of PTEN and reduced insulin-induced Akt activation in cells ( Ishihara et al. 2003 ). Very recently, it has been found that individuals with PTEN haploinsufficiency are both obese and insulin sensitive, with a decreased risk of T2D but increased risk of cancer ( Pal et al. 2012 ). The impact of this in the general population is unknown.
AKT and Related Targets
A rare missense mutation (R274H) in Akt2 leading to loss of kinase activity has been identified in a patient with diabetes ( George et al. 2004 ). Two other missense mutations (R208 K and R467W) have also been identified in diabetic patients, but surprisingly, these mutant forms display unaltered insulin-stimulated kinase activities in vitro ( Tan et al. 2007 ). In type-2 diabetic patients, a gain-of-function mutation (Q84R) in Trb3 has been associated with insulin resistance and decreased insulin-stimulated Akt phosphorylation ( Prudente et al. 2005 , 2009 ). A mutation in AS160 at position 363, resulting in a premature stop codon, was identified in a patient with severe postprandial hyperinsulinemia, and acts in a dominant-negative manner to reduce glucose transport ( Dash et al. 2009 ).
Lipotoxicity
One feature of metabolic syndrome is ectopic accumulation of lipids, especially fatty acids (FA), which is believed to cause insulin resistance via multiple mechanisms. Tissue-specific increase in lipid content in nonadipose tissues provides direct evidence of lipotoxicity. Increased hydrolysis of circulating triglycerides owing to muscle-specific overexpression of lipoprotein lipase leads to skeletal muscle insulin resistance ( Ferreira et al. 2001 ), whereas increased lipid transport in heart or liver leads to lipotoxic cardiomyopathy and nonalcoholic fatty liver disease, respectively ( Chiu et al. 2005 ; Koonen et al. 2007 ). Besides the effect of increased lipid flux on insulin sensitivity, multiple lipid intermediates have been shown to promote insulin resistance.
Elevated circulating free fatty acids (FFA) are observed in obesity and induce activation of JNK, IKK, and PKC and IRS-1 Ser-307 phosphorylation ( Schenk et al. 2008 ). The fatty acid palmitate plays a particular role in promoting insulin resistance as it induces endoplasmic reticulum (ER) stress, cytokine production, and activates JNK ( Ozcan et al. 2004 ; Shi et al. 2006 ). In addition, palmitate activates NF-κB signaling while inhibition of this pathway reverses lipid-induced insulin resistance ( Kim et al. 2001a ; Sinha et al. 2004 ). Interestingly, the detrimental effect of palmitate on skeletal muscle insulin resistance can be reversed by coinfusion with oleate, thereby changing its conversion from phospholipids and diacylglycerol (DAG) to triglycerides ( Peng et al. 2011 ). This indicates that FFA induces insulin resistance through multiple mechanisms, and combinations of FA can influences insulin signaling and highlight the crucial interplay of lipids with respect to dietary interventions.
The lipid metabolite DAG has also been shown to induce insulin resistance. Increased muscle DAG (intramyocellular lipid) leads to muscle insulin resistance by activating PKC-θ and inducing IRS-1 Ser-307 phosphorylation ( Yu et al. 2002 ). Conversely, reducing DAG levels in skeletal muscle and liver protects mice against high-fat-diet-induced insulin resistance ( Liu et al. 2007 ; Ahmadian et al. 2009 ; Samuel et al. 2010 ).
Increased plasma concentration of the sphingolipid ceramide is observed in obese and diabetic patients and is associated with severe insulin resistance ( Haus et al. 2009 ). Ceramide has been shown to induce insulin resistance via PKC and JNK activation ( Westwick et al. 1995 ; Schenk et al. 2008 ) and, thus, inhibition of ceramide synthesis ameliorates insulin resistance ( Holland et al. 2007 ). Ceramides also inhibit Akt activation by increasing the interaction of PP2A with Akt, and phosphorylation of Akt at Thr-34 by PKC ζ, resulting in reduced binding of PIP 3 to Akt ( Teruel et al. 2001 ; Powell et al. 2003 ; Blouin et al. 2010 ).
In addition to effects on kinases, alteration of membrane–lipid composition affects insulin signaling. An increase in the saturated-to-unsaturated FA ratio is observed in type-2 diabetic patients and is thought to reduce membrane fluidity and insulin sensitivity ( Field et al. 1990 ; Bakan et al. 2006 ). Moreover, an increase in the phosphatidylcholine (PC) to phosphatidylethanolamine (PE) ratio in endoplasmic reticulum leads to the activation of ER stress and is associated with insulin resistance ( Fu et al. 2011 ).
Inflammation
Obesity is characterized by the development of a chronic low-grade inflammatory state, which is considered a key component in promoting obesity-associated insulin resistance ( Osborn and Olefsky 2012 ). Adipose tissue expansion occurs in response to caloric overload, and is associated with an increase in immune cell infiltration and a subsequent proinflammatory response ( Sun et al. 2011 ). Two cell types are especially important in this scenario: adipocytes and macrophages, both of them capable of secreting proinflammatory cytokines and inducing insulin resistance. Increased secretion of the chemokine MCP-1 by adipocytes drives macrophage accumulation into adipose tissues and induces insulin resistance ( Kamei et al. 2006 ). Deletion of MCP-1 or its receptor CCR2 improves insulin sensitivity and ameliorates inflammation in mice ( Kanda et al. 2006 ; Weisberg et al. 2006 ). Increased secretion of cytokines, such as TNF-α, IL1β, or IL-6, by both immune cells and adipocytes is observed with obesity and induces insulin resistance via multiple mechanisms, including activation of Ser/Thr kinases ( Ozes et al. 2001 ; Yuan et al. 2001 ; Hirosumi et al. 2002 ; Zhang et al. 2008a ; Fan et al. 2010 ), decreasing IRS-1, GLUT4, and PPARγ expression ( Rotter et al. 2003 ; Jager et al. 2007 ), or activation of SOCS3 in adipocytes ( Steppan et al. 2005 ).
Another driving factor in obesity-associated inflammation is caused by activation of Toll-like receptor (TLR), especially activation of TLR-2 and -4. TLRs belong to the innate immune system and are generally activated by pathogen-associated molecular patterns such as LPS, and induce inflammation via activation of the NF-κB pathway ( Akira and Takeda 2004 ). TLRs are ubiquitously expressed and TLR-4 is elevated in skeletal muscle ( Reyna et al. 2008 ) and adipose tissue ( Shi et al. 2006 ) with obesity. Interestingly, saturated FA can also activate this pathway ( Lee et al. 2001 ; Shi et al. 2006 ), indicating a potential role for these receptors in obesity-driven inflammation. Thus, mice with reduced TLR-2- or TLR-4-signaling proteins ( Shi et al. 2006 ; Kleinridders et al. 2009 ; Himes and Smith 2010 ) are protected from obesity and obesity-associated insulin resistance.
Negative Regulation by Hyperglycemia
Glucose itself, at supraphysiological concentrations, is able to alter insulin sensitivity in muscle and fat, as well as decrease insulin secretion from β cells ( Leahy et al. 1986 ; Hager et al. 1991 ). Hyperglycemia induced by decreased glucose transport in skeletal muscle impairs adipose and hepatic insulin action ( Zisman et al. 2000 ; Kim et al. 2001b ) and induces insulin resistance through several pathways, which are all believed to be linked to oxidative stress ( Evans et al. 2005 ). Advanced glycosylation end products (AGE) inhibit insulin signaling by increasing Ser-307 phosphorylation of IRS-1 and forming methylglyoxal-IRS-1 adducts ( Riboulet-Chavey et al. 2006 ).
Hyperglycemia increases the flux through the polyol pathway, which causes JNK activation and increases the hexosamine-biosynthetic pathway. This has been shown to promote insulin resistance in adipose tissue, skeletal muscle, liver, and pancreas in part by O -GlcNAcylation of IRS proteins ( Marshall et al. 1991 ; Patti et al. 1999 ; McClain 2002 ; McClain et al. 2002 ). Furthermore, hyperglycemia also leads to O -GlcNAcylation of IR, which impairs receptor dimerization ( Federici et al. 1999 ), and of Foxo1 leading to increased gluconeogenic gene expression ( Housley et al. 2008 ).
Hyperglycemia also activates the PKC pathway by inducing de novo synthesis of DAG ( Xia et al. 1994 ) and causes insulin resistance by forming a multimolecular complex, including receptor of AGE/IRS-1/Src, thereby activating PKC-α and increasing IRS-1 Ser-307 phosphorylation ( Miele et al. 2003 ; Cassese et al. 2008 ).
Mitochondrial Dysfunction and ROS Formation
Although low levels of reactive oxygen species (ROS) can enhance insulin action ( Krieger-Brauer et al. 1992 ; Mahadev et al. 2001 ), high concentration of ROS causes oxidative stress when unresolved. ROS formation occurs as a by-product of the electron transport chain and is a major consequence of mitochondrial dysfunction ( Chang and Chuang 2010 ). Increased ROS levels have been observed in obese and diabetic states and can be caused by an increased metabolite flux into mitochondria, alterations in mitochondrial proteins, and reduced expression of antioxidant enzymes ( West 2000 ; Rosen et al. 2001 ; Evans et al. 2005 ; Fridlyand and Philipson 2006 ). Increased oxidative stress leads to the activation of stress kinases that induce insulin resistance by serine phosphorylation of IRS proteins ( Rudich et al. 1998 ; Evans et al. 2005 ; Dokken et al. 2008 ). Besides the aspect of ROS-mediated insulin resistance, altered mitochondrial dynamics in the form of increased mitochondrial fission leads to insulin resistance and can be rescued by inhibiting fission, which decreases the activity of p38 MAP kinase and increases IRS-1 and Akt activation ( Jheng et al. 2012 ). Impairment of mitochondrial FA oxidation in liver can also lead to elevated DAG content, resulting in PKC-ε activation and decreased IRS-2 phosphorylation and PI3-kinase activity ( Koh et al. 2005 ; Zhang et al. 2007 ).
The ER stress response, also known as unfolded protein response (UPR), is an adaptive process to ensure proper protein folding, maturation, and quality control in the ER. The three crucial pathways of the UPR (PERK, IRE1α, and ATF6) are all activated with obesity and act together to reduce the burden of unfolded proteins ( Hotamisligil 2010 ). Obese mice display enhanced PERK and IRE1α activity in adipose tissue and liver, causing JNK and IKK activation and insulin resistance by phosphorylation of IRS-1 on Ser-307 ( Ozcan et al. 2004 , 2009 ; Hu et al. 2006 ; Zhang et al. 2008b ). The transcription factor XBP-1 is activated by splicing during ER stress and increases gene expression of molecular chaperones to restore ER homeostasis. Overexpression of spliced XBP-1 reduces ER stress response, decreases activation of JNK, and increases insulin signaling by decreasing IRS-1 serine phosphorylation ( Ozcan et al. 2004 ).
CONCLUDING REMARKS
Insulin and IGF-1 acting via specific tyrosine kinase receptors propagate signals via two main branches: the PI3K-PDK-1-Akt and the Grb2-SOS-Ras-MAPK pathways that control proliferation, differentiation, and survival at the cellular level, and growth and metabolism in organisms. These signaling pathways contain several points of regulation, signal divergence, and cross talk with other signaling cascades that define critical nodes ( Taniguchi et al. 2006a ). The complexity of this signaling system is essential to mediate the variety of insulin and IGF-1 biological responses. Many steps are negatively regulated by action of phosphatases or inhibitory proteins. One of the great challenges remaining is deciphering the complexity of insulin-resistance pathogenesis. Causes of insulin resistance are numerous and the mechanisms are multifactorial. In rare cases, the cause is genetic, but in most others, insulin resistance is triggered by cellular perturbations, such as lipotoxicity, inflammation, glucotoxicity, mitochondrial dysfunction, and ER stress, which lead to deregulation of genes and inhibitory protein modifications, resulting in impaired insulin and IGF-1 action. Identifying new molecules that impact insulin signaling and new levels of control, as well as better understanding the causes and mechanisms leading to insulin resistance, will be essential for a more effective treatment of type-2 diabetes and associated diseases.
Editors: Joseph Schlessinger and Mark A. Lemmon
Additional Perspectives on Signaling by Receptor Tyrosine Kinases available at www.cshperspectives.org
- Aguirre V, Uchida T, Yenush L, Davis R, White MF 2000. The c-Jun NH(2)-terminal kinase promotes insulin resistance during association with insulin receptor substrate-1 and phosphorylation of Ser(307) . J Biol Chem 275 : 9047–9054 [ PubMed ] [ Google Scholar ]
- Aguirre V, Werner ED, Giraud J, Lee YH, Shoelson SE, White MF 2002. Phosphorylation of Ser307 in insulin receptor substrate-1 blocks interactions with the insulin receptor and inhibits insulin action . J Biol Chem 277 : 1531–1537 [ PubMed ] [ Google Scholar ]
- Ahmadian M, Duncan RE, Varady KA, Frasson D, Hellerstein MK, Birkenfeld AL, Samuel VT, Shulman GI, Wang Y, Kang C, et al. 2009. Adipose overexpression of desnutrin promotes fatty acid use and attenuates diet-induced obesity . Diabetes 58 : 855–866 [ PMC free article ] [ PubMed ] [ Google Scholar ]
- Akira S, Takeda K 2004. Toll-like receptor signalling . Nat Rev Immunol 4 : 499–511 [ PubMed ] [ Google Scholar ]
- Alessi DR, James SR, Downes CP, Holmes AB, Gaffney PR, Reese CB, Cohen P 1997. Characterization of a 3-phosphoinositide-dependent protein kinase, which phosphorylates and activates protein kinase Bα . Curr Biol 7 : 261–269 [ PubMed ] [ Google Scholar ]
- Almind K, Inoue G, Pedersen O, Kahn CR 1996. A common amino acid polymorphism in insulin receptor substrate-1 causes impaired insulin signaling. Evidence from transfection studies . J Clin Invest 97 : 2569–2575 [ PMC free article ] [ PubMed ] [ Google Scholar ]
- Almind K, Delahaye L, Hansen T, Van Obberghen E, Pedersen O, Kahn CR 2002. Characterization of the Met326Ile variant of phosphatidylinositol 3-kinase p85α . Proc Natl Acad Sci 99 : 2124–2128 [ PMC free article ] [ PubMed ] [ Google Scholar ]
- An D, Toyoda T, Taylor EB, Yu H, Fujii N, Hirshman MF, Goodyear LJ 2010. TBC1D1 regulates insulin- and contraction-induced glucose transport in mouse skeletal muscle . Diabetes 59 : 1358–1365 [ PMC free article ] [ PubMed ] [ Google Scholar ]
- Andreozzi F, Procopio C, Greco A, Mannino GC, Miele C, Raciti GA, Iadicicco C, Beguinot F, Pontiroli AE, Hribal ML, et al. 2011. Increased levels of the Akt-specific phosphatase PH domain leucine-rich repeat protein phosphatase (PHLPP)-1 in obese participants are associated with insulin resistance . Diabetologia 54 : 1879–1887 [ PubMed ] [ Google Scholar ]
- Araki E, Lipes MA, Patti ME, Bruning JC, Haag B III, Johnson RS, Kahn CR 1994. Alternative pathway of insulin signalling in mice with targeted disruption of the IRS-1 gene . Nature 372 : 186–190 [ PubMed ] [ Google Scholar ]
- Bai D, Ueno L, Vogt PK 2009. Akt-mediated regulation of NF-κB and the essentialness of NF-κB for the oncogenicity of PI3K and Akt . Int J Cancer 125 : 2863–2870 [ PMC free article ] [ PubMed ] [ Google Scholar ]
- Baier LJ, Wiedrich C, Hanson RL, Bogardus C 1998. Variant in the regulatory subunit of phosphatidylinositol 3-kinase (p85α): Preliminary evidence indicates a potential role of this variant in the acute insulin response and type 2 diabetes in Pima women . Diabetes 47 : 973–975 [ PubMed ] [ Google Scholar ]
- Bailyes EM, Nave BT, Soos MA, Orr SR, Hayward AC, Siddle K 1997. Insulin receptor/IGF-I receptor hybrids are widely distributed in mammalian tissues: Quantification of individual receptor species by selective immunoprecipitation and immunoblotting . Biochem J 327 : 209–215 [ PMC free article ] [ PubMed ] [ Google Scholar ]
- Bakan E, Yildirim A, Kurtul N, Polat MF, Dursun H, Cayir K 2006. Effects of type 2 diabetes mellitus on plasma fatty acid composition and cholesterol content of erythrocyte and leukocyte membranes . Acta Diabetol 43 : 109–113 [ PubMed ] [ Google Scholar ]
- Barroso I, Luan J, Middelberg RP, Harding AH, Franks PW, Jakes RW, Clayton D, Schafer AJ, O'Rahilly S, Wareham NJ 2003. Candidate gene association study in type 2 diabetes indicates a role for genes involved in β-cell function as well as insulin action . PLoS Biol 1 : E20. [ PMC free article ] [ PubMed ] [ Google Scholar ]
- Baumann CA, Ribon V, Kanzaki M, Thurmond DC, Mora S, Shigematsu S, Bickel PE, Pessin JE, Saltiel AR 2000. CAP defines a second signalling pathway required for insulin-stimulated glucose transport . Nature 407 : 202–207 [ PubMed ] [ Google Scholar ]
- Bayascas JR 2010. PDK1: The major transducer of PI 3-kinase actions . Curr Top Microbiol Immunol 346 : 9–29 [ PubMed ] [ Google Scholar ]
- Belfiore A, Frasca F, Pandini G, Sciacca L, Vigneri R 2009. Insulin receptor isoforms and insulin receptor/insulin-like growth factor receptor hybrids in physiology and disease . Endocr Rev 30 : 586–623 [ PubMed ] [ Google Scholar ]
- Benyoucef S, Surinya KH, Hadaschik D, Siddle K 2007. Characterization of insulin/IGF hybrid receptors: Contributions of the insulin receptor L2 and Fn1 domains and the alternatively spliced exon 11 sequence to ligand binding and receptor activation . Biochem J 403 : 603–613 [ PMC free article ] [ PubMed ] [ Google Scholar ]
- Bernal D, Almind K, Yenush L, Ayoub M, Zhang Y, Rosshani L, Larsson C, Pedersen O, White MF 1998. IRS-2 amino acid polymorphisms are not associated with random type 2 diabetes amoung caucasians . Diabetes 47 : 976–979 [ PubMed ] [ Google Scholar ]
- Bezy O, Tran TT, Pihlajamaki J, Suzuki R, Emanuelli B, Winnay J, Mori MA, Haas J, Biddinger SB, Leitges M, et al. 2011. PKCδ regulates hepatic insulin sensitivity and hepatosteatosis in mice and humans . J Clin Invest 121 : 2504–2517 [ PMC free article ] [ PubMed ] [ Google Scholar ]
- Bjornholm M, He AR, Attersand A, Lake S, Liu SCH, Lienhard GE, Taylor S, Arner P, Zierath JR 2002. Absence of functional insulin receptor substrate-3 (IRS-3) gene in humans . Diabetologia 45 : 1697–1702 [ PubMed ] [ Google Scholar ]
- Blouin CM, Prado C, Takane KK, Lasnier F, Garcia-Ocana A, Ferre P, Dugail I, Hajduch E 2010. Plasma membrane subdomain compartmentalization contributes to distinct mechanisms of ceramide action on insulin signaling . Diabetes 59 : 600–610 [ PMC free article ] [ PubMed ] [ Google Scholar ]
- Bollag GE, Roth RA, Beaudoin J, Mochly Rosen D, Koshland DE Jr 1986. Protein kinase C directly phosphorylates the insulin receptor in vitro and reduces its protein-tyrosine kinase activity . Proc Natl Acad Sci 83 : 5822–5824 [ PMC free article ] [ PubMed ] [ Google Scholar ]
- Boucher J, Tseng YH, Kahn CR 2010. Insulin and insulin-like growth factor-1 receptors act as ligand-specific amplitude modulators of a common pathway regulating gene transcription . J Biol Chem 285 : 17235–17245 [ PMC free article ] [ PubMed ] [ Google Scholar ]
- Boura-Halfon S, Zick Y 2009. Phosphorylation of IRS proteins, insulin action, and insulin resistance . Am J Physiol Endocrinol Metab 296 : E581–E591 [ PubMed ] [ Google Scholar ]
- Bouzakri K, Zachrisson A, Al Khalili L, Zhang BB, Koistinen HA, Krook A, Zierath JR 2006. siRNA-based gene silencing reveals specialized roles of IRS-1/Akt2 and IRS-2/Akt1 in glucose and lipid metabolism in human skeletal muscle . Cell Metab 4 : 89–96 [ PubMed ] [ Google Scholar ]
- Bozulic L, Surucu B, Hynx D, Hemmings BA 2008. PKBα/Akt1 acts downstream of DNA-PK in the DNA double-strand break response and promotes survival . Mol Cell 30 : 203–213 [ PubMed ] [ Google Scholar ]
- Brady MJ, Saltiel AR 2001. The role of protein phosphatase-1 in insulin action . Recent Prog Horm Res 56 : 157–173 [ PubMed ] [ Google Scholar ]
- Brognard J, Newton AC 2008. PHLiPPing the switch on Akt and protein kinase C signaling . Trends Endocrinol Metab 19 : 223–230 [ PMC free article ] [ PubMed ] [ Google Scholar ]
- Bruhn MA, Pearson RB, Hannan RD, Sheppard KE 2010. Second AKT: The rise of SGK in cancer signalling . Growth Factors 28 : 394–408 [ PubMed ] [ Google Scholar ]
- Burguete-Garcia AI, Cruz-Lopez M, Madrid-Marina V, Lopez-Ridaura R, Hernandez-Avila M, Cortina B, Gomez RE, Velasco-Mondragon E 2010. Association of Gly972Arg polymorphism of IRS1 gene with type 2 diabetes mellitus in lean participants of a national health survey in Mexico: A candidate gene study . Metabolism 59 : 38–45 [ PubMed ] [ Google Scholar ]
- Burke JE, Vadas O, Berndt A, Finegan T, Perisic O, Williams RL 2011. Dynamics of the phosphoinositide 3-kinase p110δ interaction with p85α and membranes reveals aspects of regulation distinct from p110α . Structure 19 : 1127–1137 [ PMC free article ] [ PubMed ] [ Google Scholar ]
- Cai D, Dhe-Paganon S, Melendez PA, Lee J, Shoelson SE 2003. Two new substrates in insulin signaling, IRS5/DOK4 and IRS6/DOK5 . J Biol Chem 278 : 25323–25330 [ PubMed ] [ Google Scholar ]
- Cantley LC, Neel BG 1999. New insights into tumor suppression: PTEN suppresses tumor formation by restraining the phosphoinositide 3-kinase/AKT pathway . Proc Natl Acad Sci 96 : 4240–4245 [ PMC free article ] [ PubMed ] [ Google Scholar ]
- Cardone MH, Roy N, Stennicke HR, Salvesen GS, Franke TF, Stanbridge E, Frisch S, Reed JC 1998. Regulation of cell death protease caspase-9 by phosphorylation . Science 282 : 1318–1321 [ PubMed ] [ Google Scholar ]
- Cariou B, Capitaine N, Le M, Vega VN, Bereziat V, Kergoat M, Laville M, Girard J, Vidal H, Burnol AF 2004. Increased adipose tissue expression of Grb14 in several models of insulin resistance . FASEB J 18 : 965–967 [ PubMed ] [ Google Scholar ]
- Carracedo A, Pandolfi PP 2008. The PTEN-PI3K pathway: Of feedbacks and cross-talks . Oncogene 27 : 5527–5541 [ PubMed ] [ Google Scholar ]
- Cassese A, Esposito I, Fiory F, Barbagallo AP, Paturzo F, Mirra P, Ulianich L, Giacco F, Iadicicco C, Lombardi A, et al. 2008. In skeletal muscle advanced glycation end products (AGEs) inhibit insulin action and induce the formation of multimolecular complexes including the receptor for AGEs . J Biol Chem 283 : 36088–36099 [ PMC free article ] [ PubMed ] [ Google Scholar ]
- Chagpar RB, Links PH, Pastor MC, Furber LA, Hawrysh AD, Chamberlain MD, Anderson DH 2010. Direct positive regulation of PTEN by the p85 subunit of phosphatidylinositol 3-kinase . Proc Natl Acad Sci 107 : 5471–5476 [ PMC free article ] [ PubMed ] [ Google Scholar ]
- Chakraborty A, Koldobskiy MA, Bello NT, Maxwell M, Potter JJ, Juluri KR, Maag D, Kim S, Huang AS, Dailey MJ, et al. 2010. Inositol pyrophosphates inhibit Akt signaling, thereby regulating insulin sensitivity and weight gain . Cell 143 : 897–910 [ PMC free article ] [ PubMed ] [ Google Scholar ]
- Chang YC, Chuang LM 2010. The role of oxidative stress in the pathogenesis of type 2 diabetes: From molecular mechanism to clinical implication . Am J Transl Res 2 : 316–331 [ PMC free article ] [ PubMed ] [ Google Scholar ]
- Cheng X, Xia W, Yang JY, Hsu JL, Lang JY, Chou CK, Du Y, Sun HL, Wyszomierski SL, Mills GB, et al. 2010. Activation of murine double minute 2 by Akt in mammary epithelium delays mammary involution and accelerates mammary tumorigenesis . Cancer Res 70 : 7684–7689 [ PMC free article ] [ PubMed ] [ Google Scholar ]
- Chin JE, Dickens M, Tavare JM, Roth RA 1993. Overexpression of protein kinase C isoenzymes α, β I, γ, and ε in cells overexpressing the insulin receptor. Effects on receptor phosphorylation and signaling . J Biol Chem 268 : 6338–6347 [ PubMed ] [ Google Scholar ]
- Chiu HC, Kovacs A, Blanton RM, Han X, Courtois M, Weinheimer CJ, Yamada KA, Brunet S, Xu H, Nerbonne JM, et al. 2005. Transgenic expression of fatty acid transport protein 1 in the heart causes lipotoxic cardiomyopathy . Circ Res 96 : 225–233 [ PubMed ] [ Google Scholar ]
- Cho H, Mu J, Kim JK, Thorvaldsen JL, Chu Q, Crenshaw EB III, Kaestner KH, Bartolomei MS, Shulman GI, Birnbaum MJ 2001. Insulin resistance and a diabetes mellitus-like syndrome in mice lacking the protein kinase Akt2 (PKB β) . Science 292 : 1728–1731 [ PubMed ] [ Google Scholar ]
- Clement S, Krause U, Desmedt F, Tanti JF, Behrends J, Pesesse X, Sasaki T, Penninger J, Doherty M, Malaisse W, et al. 2001. The lipid phosphatase SHIP2 controls insulin sensitivity . Nature 409 : 92–97 [ PubMed ] [ Google Scholar ]
- Cochran E, Musso C, Gorden P 2005. The use of U-500 in patients with extreme insulin resistance . Diabetes Care 28 : 1240–1244 [ PubMed ] [ Google Scholar ]
- Cooney GJ, Lyons RJ, Crew AJ, Jensen TE, Molero JC, Mitchell CJ, Biden TJ, Ormandy CJ, James DE, Daly RJ 2004. Improved glucose homeostasis and enhanced insulin signalling in Grb14-deficient mice . EMBO J 23 : 582–593 [ PMC free article ] [ PubMed ] [ Google Scholar ]
- Copps KD, Hancer NJ, Opare-Ado L, Qiu W, Walsh C, White MF 2010. Irs1 serine 307 promotes insulin sensitivity in mice . Cell Metab 11 : 84–92 [ PMC free article ] [ PubMed ] [ Google Scholar ]
- Cozzone D, Frojdo S, Disse E, Debard C, Laville M, Pirola L, Vidal H 2008. Isoform-specific defects of insulin stimulation of Akt/protein kinase B (PKB) in skeletal muscle cells from type 2 diabetic patients . Diabetologia 51 : 512–521 [ PubMed ] [ Google Scholar ]
- Dash S, Sano H, Rochford JJ, Semple RK, Yeo G, Hyden CS, Soos MA, Clark J, Rodin A, Langenberg C, et al. 2009. A truncation mutation in TBC1D4 in a family with acanthosis nigricans and postprandial hyperinsulinemia . Proc Natl Acad Sci 106 : 9350–9355 [ PMC free article ] [ PubMed ] [ Google Scholar ]
- Datta SR, Dudek H, Tao X, Masters S, Fu H, Gotoh Y, Greenberg ME 1997. Akt phosphorylation of BAD couples survival signals to the cell-intrinsic death machinery . Cell 91 : 231–241 [ PubMed ] [ Google Scholar ]
- De Fea K, Roth RA 1997a. Modulation of insulin receptor substrate-1 tyrosine phosphorylation and function by mitogen-activated protein kinase . J Biol Chem 272 : 31400–31406 [ PubMed ] [ Google Scholar ]
- De Fea K, Roth RA 1997b. Protein kinase C modulation of insulin receptor substrate-1 tyrosine phosphorylation requires serine 612 . Biochemistry 36 : 12939–12947 [ PubMed ] [ Google Scholar ]
- Degerman E, Ahmad F, Chung YW, Guirguis E, Omar B, Stenson L, Manganiello V 2011. From PDE3B to the regulation of energy homeostasis . Curr Opin Pharmacol 11 : 676–682 [ PMC free article ] [ PubMed ] [ Google Scholar ]
- Deyev IE, Sohet F, Vassilenko KP, Serova OV, Popova NV, Zozulya SA, Burova EB, Houillier P, Rzhevsky DI, Berchatova AA, et al. 2011. Insulin receptor-related receptor as an extracellular alkali sensor . Cell Metab 13 : 679–689 [ PMC free article ] [ PubMed ] [ Google Scholar ]
- Dimmeler S, Fleming I, Fisslthaler B, Hermann C, Busse R, Zeiher AM 1999. Activation of nitric oxide synthase in endothelial cells by Akt-dependent phosphorylation . Nature 399 : 601–605 [ PubMed ] [ Google Scholar ]
- Dokken BB, Saengsirisuwan V, Kim JS, Teachey MK, Henriksen EJ 2008. Oxidative stress-induced insulin resistance in rat skeletal muscle: Role of glycogen synthase kinase-3 . Am J Physiol Endocrinol Metab 294 : E615–E621 [ PubMed ] [ Google Scholar ]
- Du K, Herzig S, Kulkarni RN, Montminy M 2003. TRB3: A tribbles homolog that inhibits Akt/PKB activation by insulin in liver . Science 300 : 1574–1577 [ PubMed ] [ Google Scholar ]
- Dunaif A, Xia J, Book CB, Schenker E, Tang Z 1995. Excessive insulin receptor serine phosphorylation in cultured fibroblasts and in skeletal muscle. A potential mechanism for insulin resistance in the polycystic ovary syndrome . J Clin Invest 96 : 801–810 [ PMC free article ] [ PubMed ] [ Google Scholar ]
- Duvel K, Yecies JL, Menon S, Raman P, Lipovsky AI, Souza AL, Triantafellow E, Ma Q, Gorski R, Cleaver S, et al. 2010. Activation of a metabolic gene regulatory network downstream of mTOR complex 1 . Mol Cell 39 : 171–183 [ PMC free article ] [ PubMed ] [ Google Scholar ]
- Elchebly M, Payette P, Michaliszyn E, Cromlish W, Collins S, Loy AL, Normandin DCA, imms-Hagen J, Chan C, Ramachandran C, et al. 1999. Increased insulin sensitivity and obesity resistance in mice lacking the protein tyrosine phosphatase-1B gene . Science 283 : 1544–1548 [ PubMed ] [ Google Scholar ]
- Emanuelli B, Peraldi P, Filloux C, Sawka-Verhelle D, Hilton D, Van OE 2000. SOCS-3 is an insulin-induced negative regulator of insulin signaling . J Biol Chem 275 : 15985–15991 [ PubMed ] [ Google Scholar ]
- Emanuelli B, Peraldi P, Filloux C, Chavey C, Freidinger L, Hilton DJ, Hotamisligil GS, Van OE 2001. SOCS-3 inhibits insulin signaling and is up-regulated in response to tumor necrosis factor-α in the adipose tissue of obese mice . J Biol Chem 276 : 47944–47949 [ PubMed ] [ Google Scholar ]
- Esposito DL, Li Y, Vanni C, Mammarella S, Veschi S, Della LF, Mariani-Costantini R, Battista P, Quon MJ, Cama A 2003. A novel T608R missense mutation in insulin receptor substrate-1 identified in a subject with type 2 diabetes impairs metabolic insulin signaling . J Clin Endocrinol Metab 88 : 1468–1475 [ PubMed ] [ Google Scholar ]
- Evans JL, Maddux BA, Goldfine ID 2005. The molecular basis for oxidative stress-induced insulin resistance . Antioxid Redox Signal 7 : 1040–1052 [ PubMed ] [ Google Scholar ]
- Fan Y, Yu Y, Shi Y, Sun W, Xie M, Ge N, Mao R, Chang A, Xu G, Schneider MD, et al. 2010. Lysine 63-linked polyubiquitination of TAK1 at lysine 158 is required for tumor necrosis factor α- and interleukin-1β-induced IKK/NF-κB and JNK/AP-1 activation . J Biol Chem 285 : 5347–5360 [ PMC free article ] [ PubMed ] [ Google Scholar ]
- Fantin VR, Lavan BE, Wang Q, Jenkins NA, Gilbert DJ, Copeland NG, Keller SR, Lienhard GE 1999. Cloning, tissue expression, and chromosomal location of the mouse insulin receptor substrate 4 gene . Endocrinology 140 : 1329–1337 [ PubMed ] [ Google Scholar ]
- Fantin VR, Wang Q, Lienhard GE, Keller SR 2000. Mice lacking insulin receptor substrate 4 exhibit mild defects in growth, reproduction, and glucose homeostasis . Am J Physiol Endocrinol Metab 278 : E127–E133 [ PubMed ] [ Google Scholar ]
- Farese RV, Sajan MP 2010. Metabolic functions of atypical protein kinase C: “Good” and “bad” as defined by nutritional status . Am J Physiol Endocrinol Metab 298 : E385–E394 [ PMC free article ] [ PubMed ] [ Google Scholar ]
- Farese RV, Sajan MP, Yang H, Li P, Mastorides S, Gower WR Jr, Nimal S, Choi CS, Kim S, Shulman GI, et al. 2007. Muscle-specific knockout of PKC-λ impairs glucose transport and induces metabolic and diabetic syndromes . J Clin Invest 117 : 2289–2301 [ PMC free article ] [ PubMed ] [ Google Scholar ]
- Federici M, Giaccari A, Hribal ML, Giovannone B, Lauro D, Morviducci L, Pastore L, Tamburrano G, Lauro R, Sesti G 1999. Evidence for glucose/hexosamine in vivo regulation of insulin/IGF-I hybrid receptor assembly . Diabetes 48 : 2277–2285 [ PubMed ] [ Google Scholar ]
- Ferreira LD, Pulawa LK, Jensen DR, Eckel RH 2001. Overexpressing human lipoprotein lipase in mouse skeletal muscle is associated with insulin resistance . Diabetes 50 : 1064–1068 [ PubMed ] [ Google Scholar ]
- Field CJ, Ryan EA, Thomson AB, Clandinin MT 1990. Diet fat composition alters membrane phospholipid composition, insulin binding, and glucose metabolism in adipocytes from control and diabetic animals . J Biol Chem 265 : 11143–11150 [ PubMed ] [ Google Scholar ]
- Florez JC, Sjogren M, Burtt N, Orho-Melander M, Schayer S, Sun M, Almgren P, Lindblad U, Tuomi T, Gaudet D, et al. 2004. Association testing in 9,000 people fails to confirm the association of the insulin receptor substrate-1 G972R polymorphism with type 2 diabetes . Diabetes 53 : 3313–3318 [ PubMed ] [ Google Scholar ]
- Frasca F, Pandini G, Scalia P, Sciacca L, Mineo R, Costantino A, Goldfine ID, Belfiore A, Vigneri R 1999. Insulin receptor isoform A, a newly recognized, high-affinity insulin-like growth factor II receptor in fetal and cancer cells . Mol Cell Biol 19 : 3278–3288 [ PMC free article ] [ PubMed ] [ Google Scholar ]
- Fridlyand LE, Philipson LH 2006. Reactive species and early manifestation of insulin resistance in type 2 diabetes . Diabetes Obes Metab 8 : 136–145 [ PubMed ] [ Google Scholar ]
- Fu S, Yang L, Li P, Hofmann O, Dicker L, Hide W, Lin X, Watkins SM, Ivanov AR, Hotamisligil GS 2011. Aberrant lipid metabolism disrupts calcium homeostasis causing liver endoplasmic reticulum stress in obesity . Nature 473 : 528–531 [ PMC free article ] [ PubMed ] [ Google Scholar ]
- Fulton D, Gratton JP, McCabe TJ, Fontana J, Fujio Y, Walsh K, Franke TF, Papapetropoulos A, Sessa WC 1999. Regulation of endothelium-derived nitric oxide production by the protein kinase Akt . Nature 399 : 597–601 [ PMC free article ] [ PubMed ] [ Google Scholar ]
- Gao Z, Hwang D, Bataille F, Lefevre M, York D, Quon M, Ye J 2002. Serine phosphorylation of insulin receptor substrate 1 (IRS-1) by inhibitor KB kinase (IKK) complex . J Biol Chem 277 : 48115–48121 [ PubMed ] [ Google Scholar ]
- Gardai SJ, Hildeman DA, Frankel SK, Whitlock BB, Frasch SC, Borregaard N, Marrack P, Bratton DL, Henson PM 2004. Phosphorylation of Bax Ser184 by Akt regulates its activity and apoptosis in neutrophils . J Biol Chem 279 : 21085–21095 [ PubMed ] [ Google Scholar ]
- George S, Rochford JJ, Wolfrum C, Gray SL, Schinner S, Wilson JC, Soos MA, Murgatroyd PR, Williams RM, Acerini CL, et al. 2004. A family with severe insulin resistance and diabetes due to a mutation in AKT2 . Science 304 : 1325–1328 [ PMC free article ] [ PubMed ] [ Google Scholar ]
- Goldstein BJ, Ahmad F, Ding W, Li PM, Zhang WR 1998. Regulation of the insulin signalling pathway by cellular protein-tyrosine phosphatases . Mol Cell Biochem 182 : 91–99 [ PubMed ] [ Google Scholar ]
- Gual P, Gonzalez T, Gremeaux T, Barres R, Marchand-Brustel Y, Tanti JF 2003. Hyperosmotic stress inhibits insulin receptor substrate-1 function by distinct mechanisms in 3T3-L1 adipocytes . J Biol Chem 278 : 26550–26557 [ PubMed ] [ Google Scholar ]
- Hager SR, Jochen AL, Kalkhoff RK 1991. Insulin resistance in normal rats infused with glucose for 72 h . Am J Physiol 260 : E353–E362 [ PubMed ] [ Google Scholar ]
- Hanke S, Mann M 2009. The phosphotyrosine interactome of the insulin receptor family and its substrates IRS-1 and IRS-2 . Mol Cell Proteomics 8 : 519–534 [ PMC free article ] [ PubMed ] [ Google Scholar ]
- Harrington LS, Findlay GM, Gray A, Tolkacheva T, Wigfield S, Rebholz H, Barnett J, Leslie NR, Cheng S, Shepherd PR, et al. 2004. The TSC1-2 tumor suppressor controls insulin-PI3K signaling via regulation of IRS proteins . J Cell Biol 166 : 213–223 [ PMC free article ] [ PubMed ] [ Google Scholar ]
- Haruta T, Imamura T, Iwanishi M, Egawa K, Goji K, Kobayashi M 1995. Amplification and analysis of promoter region of insulin receptor gene in a patient with leprechaunism associated with severe insulin resistance . Metabolism 44 : 430–437 [ PubMed ] [ Google Scholar ]
- Haus JM, Kashyap SR, Kasumov T, Zhang R, Kelly KR, DeFronzo RA, Kirwan JP 2009. Plasma ceramides are elevated in obese subjects with type 2 diabetes and correlate with the severity of insulin resistance . Diabetes 58 : 337–343 [ PMC free article ] [ PubMed ] [ Google Scholar ]
- Himes RW, Smith CW 2010. Tlr2 is critical for diet-induced metabolic syndrome in a murine model . FASEB J 24 : 731–739 [ PMC free article ] [ PubMed ] [ Google Scholar ]
- Hirosumi J, Tuncman G, Chang L, Gorgun CZ, Uysal KT, Maeda K, Karin M, Hotamisligil GS 2002. A central role for JNK in obesity and insulin resistance . Nature 420 : 333–336 [ PubMed ] [ Google Scholar ]
- Holland WL, Brozinick JT, Wang LP, Hawkins ED, Sargent KM, Liu Y, Narra K, Hoehn KL, Knotts TA, Siesky A, et al. 2007. Inhibition of ceramide synthesis ameliorates glucocorticoid-, saturated-fat-, and obesity-induced insulin resistance . Cell Metab 5 : 167–179 [ PubMed ] [ Google Scholar ]
- Holt LJ, Siddle K 2005. Grb10 and Grb14: Enigmatic regulators of insulin action—and more? Biochem J 388 : 393–406 [ PMC free article ] [ PubMed ] [ Google Scholar ]
- Holt LJ, Lyons RJ, Ryan AS, Beale SM, Ward A, Cooney GJ, Daly RJ 2009. Dual ablation of Grb10 and Grb14 in mice reveals their combined role in regulation of insulin signaling and glucose homeostasis . Mol Endocrinol 23 : 1406–1414 [ PMC free article ] [ PubMed ] [ Google Scholar ]
- Hotamisligil GS 2010. Endoplasmic reticulum stress and the inflammatory basis of metabolic disease . Cell 140 : 900–917 [ PMC free article ] [ PubMed ] [ Google Scholar ]
- Housley MP, Rodgers JT, Udeshi ND, Kelly TJ, Shabanowitz J, Hunt DF, Puigserver P, Hart GW 2008. O -GlcNAc regulates FoxO activation in response to glucose . J Biol Chem 283 : 16283–16292 [ PMC free article ] [ PubMed ] [ Google Scholar ]
- Hribal ML, Tornei F, Pujol A, Menghini R, Barcaroli D, Lauro D, Amoruso R, Lauro R, Bosch F, Sesti G, et al. 2008. Transgenic mice overexpressing human G972R IRS-1 show impaired insulin action and insulin secretion . J Cell Mol Med 12 : 2096–2106 [ PMC free article ] [ PubMed ] [ Google Scholar ]
- Hsu PP, Kang SA, Rameseder J, Zhang Y, Ottina KA, Lim D, Peterson TR, Choi Y, Gray NS, Yaffe MB, et al. 2011. The mTOR-regulated phosphoproteome reveals a mechanism of mTORC1-mediated inhibition of growth factor signaling . Science 332 : 1317–1322 [ PMC free article ] [ PubMed ] [ Google Scholar ]
- Hu P, Han Z, Couvillon AD, Kaufman RJ, Exton JH 2006. Autocrine tumor necrosis factor α links endoplasmic reticulum stress to the membrane death receptor pathway through IRE1α-mediated NF-κB activation and down-regulation of TRAF2 expression . Mol Cell Biol 26 : 3071–3084 [ PMC free article ] [ PubMed ] [ Google Scholar ]
- Huang C, Thirone AC, Huang X, Klip A 2005. Differential contribution of insulin receptor substrates 1 versus 2 to insulin signaling and glucose uptake in l6 myotubes . J Biol Chem 280 : 19426–19435 [ PubMed ] [ Google Scholar ]
- Ishihara H, Sasaoka T, Kagawa S, Murakami S, Fukui K, Kawagishi Y, Yamazaki K, Sato A, Iwata M, Urakaze M, et al. 2003. Association of the polymorphisms in the 5′-untranslated region of PTEN gene with type 2 diabetes in a Japanese population . Growth Regul 554 : 450–454 [ PubMed ] [ Google Scholar ]
- Jager J, Gremeaux T, Cormont M, Le Marchand-Brustel Y, Tanti JF 2007. Interleukin-1β-induced insulin resistance in adipocytes through down-regulation of insulin receptor substrate-1 expression . Endocrinology 148 : 241–251 [ PMC free article ] [ PubMed ] [ Google Scholar ]
- Jheng HF, Tsai PJ, Guo SM, Kuo LH, Chang CS, Su IJ, Chang CR, Tsai YS 2012. Mitochondrial fission contributes to mitochondrial dysfunction and insulin resistance in skeletal muscle . Mol Cell Biol 32 : 309–319 [ PMC free article ] [ PubMed ] [ Google Scholar ]
- Jia S, Liu Z, Zhang S, Liu P, Zhang L, Lee SH, Zhang J, Signoretti S, Loda M, Roberts TM, et al. 2008. Essential roles of PI3K-p110β in cell growth, metabolism and tumorigenesis . Nature 454 : 776–779 [ PMC free article ] [ PubMed ] [ Google Scholar ]
- Kagawa S, Soeda Y, Ishihara H, Oya T, Sasahara M, Yaguchi S, Oshita R, Wada T, Tsuneki H, Sasaoka T 2008. Impact of transgenic overexpression of SH2-containing inositol 5′-phosphatase 2 on glucose metabolism and insulin signaling in mice . Endocrinology 149 : 642–650 [ PubMed ] [ Google Scholar ]
- Kahn CR, Flier JS, Bar RS, Archer JA, Gorden P, Martin MM, Roth J 1976. The syndromes of insulin resistance and acanthosis nigricans. Insulin-receptor disorders in man . N Engl J Med 294 : 739–745 [ PubMed ] [ Google Scholar ]
- Kamei N, Tobe K, Suzuki R, Ohsugi M, Watanabe T, Kubota N, Ohtsuka-Kowatari N, Kumagai K, Sakamoto K, Kobayashi M, et al. 2006. Overexpression of monocyte chemoattractant protein-1 in adipose tissues causes macrophage recruitment and insulin resistance . J Biol Chem 281 : 26602–26614 [ PubMed ] [ Google Scholar ]
- Kanda H, Tateya S, Tamori Y, Kotani K, Hiasa K, Kitazawa R, Kitazawa S, Miyachi H, Maeda S, Egashira K, et al. 2006. MCP-1 contributes to macrophage infiltration into adipose tissue, insulin resistance, and hepatic steatosis in obesity . J Clin Invest 116 : 1494–1505 [ PMC free article ] [ PubMed ] [ Google Scholar ]
- Karasik A, Rothenberg PL, Yamada K, White MF, Kahn CR 1990. Increased protein kinase C activity is linked to reduced insulin receptor autophosphorylation in liver of starved rats . J Biol Chem 265 : 10226–10231 [ PubMed ] [ Google Scholar ]
- Kim JK, Kim YJ, Fillmore JJ, Chen Y, Moore I, Lee J, Yuan M, Li ZW, Karin M, Perret P, et al. 2001a. Prevention of fat-induced insulin resistance by salicylate . J Clin Invest 108 : 437–446 [ PMC free article ] [ PubMed ] [ Google Scholar ]
- Kim JK, Zisman A, Fillmore JJ, Peroni OD, Kotani K, Perret P, Zong H, Kahn CR, Kahn BB, Shulman GI 2001b. Glucose toxicity and the development of diabetes in mice with muscle-specific inactivation of GLUT4 . J Clin Invest 108 : 153–160 [ PMC free article ] [ PubMed ] [ Google Scholar ]
- Kim JK, Fillmore JJ, Sunshine MJ, Albrecht B, Higashimori T, Kim DW, Liu ZX, Soos TJ, Cline GW, O'Brien WR, et al. 2004a. PKC-θ knockout mice are protected from fat-induced insulin resistance . J Clin Invest 114 : 823–827 [ PMC free article ] [ PubMed ] [ Google Scholar ]
- Kim KH, Song JJ, Yoo EJ, Choe SS, Park SD, Kim JB 2004b. Regulatory role of glycogen synthase kinase 3 for criptional activity of ADD1/SREBP1c . J Biol Chem 279 : 51999–52006 [ PubMed ] [ Google Scholar ]
- Kitamura T, Kitamura Y, Kuroda S, Hino Y, Ando M, Kotani K, Konishi H, Matsuzaki H, Kikkawa U, Ogawa W, et al. 1999. Insulin-induced phosphorylation and activation of cyclic nucleotide phosphodiesterase 3B by the serine-threonine kinase Akt . Mol Cell Biol 19 : 6286–6296 [ PMC free article ] [ PubMed ] [ Google Scholar ]
- Klaman LD, Boss O, Peroni OD, Kim JK, Martino JL, Zabolotny JM, Moghal N, Lubkin M, Kim YB, Sharpe AH, et al. 2000. Increased energy expenditure, decreased adiposity, and tissue-specific insulin sensitivity in protein-tyrosine phosphatase 1B-deficient mice . Mol Cell Biol 20 : 5479–5489 [ PMC free article ] [ PubMed ] [ Google Scholar ]
- Kleinridders A, Schenten D, Konner AC, Belgardt BF, Mauer J, Okamura T, Wunderlich FT, Medzhitov R, Bruning JC 2009. MyD88 signaling in the CNS is required for development of fatty acid-induced leptin resistance and diet-induced obesity . Cell Metab 10 : 249–259 [ PMC free article ] [ PubMed ] [ Google Scholar ]
- Koh EH, Lee WJ, Kim MS, Park JY, Lee IK, Lee KU 2005. Intracellular fatty acid metabolism in skeletal muscle and insulin resistance . Curr Diabetes Rev 1 : 331–336 [ PubMed ] [ Google Scholar ]
- Koo SH, Satoh H, Herzig S, Lee CH, Hedrick S, Kulkarni R, Evans RM, Olefsky J, Montminy M 2004. PGC-1 promotes insulin resistance in liver through PPAR-α-dependent induction of TRB-3 . Nat Med 10 : 530–534 [ PubMed ] [ Google Scholar ]
- Koonen DP, Jacobs RL, Febbraio M, Young ME, Soltys CL, Ong H, Vance DE, Dyck JR 2007. Increased hepatic CD36 expression contributes to dyslipidemia associated with diet-induced obesity . Diabetes 56 : 2863–2871 [ PubMed ] [ Google Scholar ]
- Kowluru A, Matti A 2012. Hyperactivation of protein phosphatase 2A in models of glucolipotoxicity and diabetes: Potential mechanisms and functional consequences . Biochem Pharmacol 84 : 591–597 [ PubMed ] [ Google Scholar ]
- Krieger-Brauer HI, Kather H 1992. Human fat cells possess a plasma membrane-bound H 2 O 2 -generating system that is activated by insulin via a mechanism bypassing the receptor kinase . J Clin Invest 89 : 1006–1013 [ PMC free article ] [ PubMed ] [ Google Scholar ]
- Kurlawalla-Martinez C, Stiles B, Wang Y, Devaskar SU, Kahn BB, Wu H 2005. Insulin hypersensitivity and resistance to streptozotocin-induced diabetes in mice lacking PTEN in adipose tissue . Mol Cell Biol 25 : 2498–2510 [ PMC free article ] [ PubMed ] [ Google Scholar ]
- Laustsen PG, Michael MD, Crute BE, Cohen SE, Ueki K, Kulkarni RN, Keller SR, Lienhard GE, Kahn CR 2002. Lipoatrophic diabetes in Irs1 −/− /Irs3 −/− double knockout mice . Genes Dev 16 : 3213–3222 [ PMC free article ] [ PubMed ] [ Google Scholar ]
- Lavan BE, Fantin VR, Chang ET, Lane WS, Keller SR, Lienhard GE 1997a. A novel 160-kDa phosphotyrosine protein in insulin-treated embryonic kidney cells is a new member of the insulin receptor substrate family . J Biol Chem 272 : 21403–21407 [ PubMed ] [ Google Scholar ]
- Lavan BE, Lane WS, Lienhard GE 1997b. The 60-kDa phosphotyrosine protein in insulin-treated adipocytes is a new member of the insulin receptor substrate family . J Biol Chem 272 : 11439–11443 [ PubMed ] [ Google Scholar ]
- Leahy JL, Cooper HE, Deal DA, Weir DG 1986. Chronic hyperglycemia is associated with impaired glucose influence on insulin secretion. A study in normal rats using chronic in vivo glucose infusions . J Clin Invest 77 : 908–915 [ PMC free article ] [ PubMed ] [ Google Scholar ]
- Lee JY, Sohn KH, Rhee SH, Hwang D 2001. Saturated fatty acids, but not unsaturated fatty acids, induce the expression of cyclooxygenase-2 mediated through Toll-like receptor 4 . J Biol Chem 276 : 16683–16689 [ PubMed ] [ Google Scholar ]
- Lewis RE, Cao L, Perregaux D, Czech MP 1990. Threonine 1336 of the human insulin receptor is a major target for phosphorylation by protein kinase C . Biochemistry 29 : 1807–1813 [ PubMed ] [ Google Scholar ]
- Li Y, Soos TJ, Li X, Wu J, Degennaro M, Sun X, Littman DR, Birnbaum MJ, Polakiewicz RD 2004. Protein kinase C θ inhibits insulin signaling by phosphorylating IRS1 at Ser(1101) . J Biol Chem 279 : 45304–45307 [ PubMed ] [ Google Scholar ]
- Li X, Monks B, Ge Q, Birnbaum MJ 2007. Akt/PKB regulates hepatic metabolism by directly inhibiting PGC-1α cription coactivator . Nature 447 : 1012–1016 [ PubMed ] [ Google Scholar ]
- Liu L, Zhang Y, Chen N, Shi X, Tsang B, Yu BH 2007. Upregulation of myocellular DGAT1 augments triglyceride synthesis in skeletal muscle and protects against fat-induced insulin resistance . J Clin Invest 117 : 1679–1689 [ PMC free article ] [ PubMed ] [ Google Scholar ]
- Lu M, Wan M, Leavens KF, Chu Q, Monks BR, Fernandez S, Ahima RS, Ueki K, Kahn CR, Birnbaum MJ 2012. Insulin regulates liver metabolism in vivo in the absence of hepatic Akt and Foxo1 . Nat Med 18 : 388–395 [ PMC free article ] [ PubMed ] [ Google Scholar ]
- Mack E, Ziv E, Reuveni H, Kalman R, Niv MY, Jorns A, Lenzen S, Shafrir E 2008. Prevention of insulin resistance and β-cell loss by abrogating PKCε-induced serine phosphorylation of muscle IRS-1 in Psammomys obesus . Diabetes Metab Res Rev 24 : 577–584 [ PubMed ] [ Google Scholar ]
- Mahadev K, Zilbering A, Zhu L, Goldstein BJ 2001. Insulin-stimulated hydrogen peroxide reversibly inhibits protein-tyrosine phosphatase 1b in vivo and enhances the early insulin action cascade . J Biol Chem 276 : 21938–21942 [ PubMed ] [ Google Scholar ]
- Manning BD, Cantley LC 2007. AKT/PKB signaling: Navigating downstream . Cell 129 : 1261–1274 [ PMC free article ] [ PubMed ] [ Google Scholar ]
- Marshall S, Bacote V, Traxinger RR 1991. Discovery of a metabolic pathway mediating glucose-induced desensitization of the glucose transport system. Role of hexosamine biosynthesis in the induction of insulin resistance . J Biol Chem 266 : 4706–4712 [ PubMed ] [ Google Scholar ]
- Martinez-Gomez LE, Cruz M, Martinez-Nava GA, Madrid-Marina V, Parra E, Garcia-Mena J, Espinoza-Rojo M, Estrada-Velasco BI, Piza-Roman LF, Aguilera P, et al. 2011. A replication study of the IRS1, CAPN10, TCF7L2, and PPARG gene polymorphisms associated with type 2 diabetes in two different populations of Mexico . Ann Hum Genet 75 : 612–620 [ PubMed ] [ Google Scholar ]
- Matsumoto M, Ogawa W, Akimoto K, Inoue H, Miyake K, Furukawa K, Hayashi Y, Iguchi H, Matsuki Y, Hiramatsu R, et al. 2003. PKCλ in liver mediates insulin-induced SREBP-1c expression and determines both hepatic lipid content and overall insulin sensitivity . J Clin Invest 112 : 935–944 [ PMC free article ] [ PubMed ] [ Google Scholar ]
- Matsushima R, Harada N, Webster NJ, Tsutsumi YM, Nakaya Y 2006. Effect of TRB3 on insulin and nutrient-stimulated hepatic p70 S6 kinase activity . J Biol Chem 281 : 29719–29729 [ PubMed ] [ Google Scholar ]
- McClain DA 2002. Hexosamines as mediators of nutrient sensing and regulation in diabetes . J Diabetes Complications 16 : 72–80 [ PubMed ] [ Google Scholar ]
- McClain DA, Lubas WA, Cooksey RC, Hazel M, Parker GJ, Love DC, Hanover JA 2002. Altered glycan-dependent signaling induces insulin resistance and hyperleptinemia . Proc Natl Acad Sci 99 : 10695–10699 [ PMC free article ] [ PubMed ] [ Google Scholar ]
- Miele C, Riboulet A, Maitan MA, Oriente F, Romano C, Formisano P, Giudicelli J, Beguinot F, Van Obberghen E 2003. Human glycated albumin affects glucose metabolism in L6 skeletal muscle cells by impairing insulin-induced insulin receptor substrate (IRS) signaling through a protein kinase C α-mediated mechanism . J Biol Chem 278 : 47376–47387 [ PubMed ] [ Google Scholar ]
- Miki H, Yamauchi T, Suzuki R, Komeda K, Tsuchida A, Kubota N, Terauchi Y, Kamon J, Kaburagi Y, Matsui J, et al. 2001. Essential role of insulin receptor substrate 1 (IRS-1) and IRS-2 in adipocyte differentiation . Mol Cell Biol 21 : 2521–2532 [ PMC free article ] [ PubMed ] [ Google Scholar ]
- Millward TA, Zolnierowicz S, Hemmings BA 1999. Regulation of protein kinase cascades by protein phosphatase 2A . Trends Biochem Sci 24 : 186–191 [ PubMed ] [ Google Scholar ]
- Mosthaf L, Grako K, Dull TJ, Coussens L, Ullrich A, McClain DA 1990. Functionally distinct insulin receptors generated by tissue-specific alternative splicing . EMBO J 9 : 2409–2413 [ PMC free article ] [ PubMed ] [ Google Scholar ]
- Motti ML, De MC, Califano D, Fusco A, Viglietto G 2004. Akt-dependent T198 phosphorylation of cyclin-dependent kinase inhibitor p27kip1 in breast cancer . Cell Cycle 3 : 1074–1080 [ PubMed ] [ Google Scholar ]
- Myers MG Jr, Backer JM, Sun XJ, Shoelson S, Hu P, Schlessinger J, Yoakim M, Schaffhausen B, White MF 1992. IRS-1 activates phosphatidylinositol 3′-kinase by associating with src homology 2 domains of p85 Proc Natl Acad Sci 89 : 10350–10354 [ PMC free article ] [ PubMed ] [ Google Scholar ]
- Nef S, Verma-Kurvari S, Merenmies J, Vassalli JD, Efstratiadis A, Accili D, Parada LF 2003. Testis determination requires insulin receptor family function in mice . Nature 426 : 291–295 [ PubMed ] [ Google Scholar ]
- Ni YG, Wang N, Cao DJ, Sachan N, Morris DJ, Gerard RD, Kuro O, Rothermel BA, Hill JA 2007. FoxO cription factors activate Akt and attenuate insulin signaling in heart by inhibiting protein phosphatases . Proc Natl Acad Sci 104 : 20517–20522 [ PMC free article ] [ PubMed ] [ Google Scholar ]
- Oh WJ, Jacinto E 2011. mTOR complex 2 signaling and functions . Cell Cycle 10 : 2305–2316 [ PMC free article ] [ PubMed ] [ Google Scholar ]
- Osborn O, Olefsky JM 2012. The cellular and signaling networks linking the immune system and metabolism in disease . Nat Med 18 : 363–374 [ PubMed ] [ Google Scholar ]
- Ozcan U, Cao Q, Yilmaz E, Lee AH, Iwakoshi NN, Ozdelen E, Tuncman G, Gorgun C, Glimcher LH, Hotamisligil GS 2004. Endoplasmic reticulum stress links obesity, insulin action, and type 2 diabetes . Science 306 : 457–461 [ PubMed ] [ Google Scholar ]
- Ozcan L, Ergin AS, Lu A, Chung J, Sarkar S, Nie D, Myers MG Jr, Ozcan U 2009. Endoplasmic reticulum stress plays a central role in development of leptin resistance . Cell Metab 9 : 35–51 [ PubMed ] [ Google Scholar ]
- Ozes ON, Akca H, Mayo LD, Gustin JA, Maehama T, Dixon JE, Donner DB 2001. A phosphatidylinositol 3-kinase/Akt/mTOR pathway mediates and PTEN antagonizes tumor necrosis factor inhibition of insulin signaling through insulin receptor substrate-1 . Proc Natl Acad Sci 98 : 4640–4645 [ PMC free article ] [ PubMed ] [ Google Scholar ]
- Pal A, Barber TM, Van de Bunt M, Rudge SA, Zhang Q, Lachlan KL, Cooper NS, Linden H, Levy JC, Wakelam MJ, et al. 2012. PTEN mutations as a cause of constitutive insulin sensitivity and obesity . N Engl J Med 367 : 1002–1011 [ PMC free article ] [ PubMed ] [ Google Scholar ]
- Pandini G, Vigneri R, Costantino A, Frasca F, Ippolito A, Fujita-Yamaguchi Y, Siddle K, Goldfine ID, Belfiore A 1999. Insulin and insulin-like growth factor-I (IGF-I) receptor overexpression in breast cancers leads to insulin/IGF-I hybrid receptor overexpression: Evidence for a second mechanism of IGF-I signaling . Clin Cancer Res 5 : 1935–1944 [ PubMed ] [ Google Scholar ]
- Park SW, Zhou Y, Lee J, Lu A, Sun C, Chung J, Ueki K, Ozcan J 2010. The regulatory subunits of PI3K, p85α and p85β, interact with XBP-1 and increase its nuclear translocation . Nat Med 16 : 429–437 [ PMC free article ] [ PubMed ] [ Google Scholar ]
- Patti ME, Virkamaki A, Landaker EJ, Kahn CR, Yki-Jarvinen H 1999. Activation of the hexosamine pathway by glucosamine in vivo induces insulin resistance of early postreceptor insulin signaling events in skeletal muscle . Diabetes 48 : 1562–1571 [ PubMed ] [ Google Scholar ]
- Pearce LR, Komander D, Alessi DR 2010. The nuts and bolts of AGC protein kinases . Nat Rev Mol Cell Biol 11 : 9–22 [ PubMed ] [ Google Scholar ]
- Peng G, Li L, Liu Y, Pu J, Zhang S, Yu J, Zhao J, Liu P 2011. Oleate blocks palmitate-induced abnormal lipid distribution, endoplasmic reticulum expansion and stress, and insulin resistance in skeletal muscle . Endocrinology 152 : 2206–2218 [ PubMed ] [ Google Scholar ]
- Powell DJ, Hajduch E, Kular G, Hundal HS 2003. Ceramide disables 3-phosphoinositide binding to the pleckstrin homology domain of protein kinase B (PKB)/Akt by a PKCζ-dependent mechanism . Mol Cell Biol 23 : 7794–7808 [ PMC free article ] [ PubMed ] [ Google Scholar ]
- Powell DJ, Turban S, Gray A, Hajduch E, Hundal HS 2004. Intracellular ceramide synthesis and protein kinase Cζ activation play an essential role in palmitate-induced insulin resistance in rat L6 skeletal muscle cells . Biochem J 382 : 619–629 [ PMC free article ] [ PubMed ] [ Google Scholar ]
- Prudente S, Hribal ML, Flex E, Turchi F, Morini E, De Cosmo S, Bacci S, Tassi V, Cardellini M, Lauro R, et al. 2005. The functional Q84R polymorphism of mammalian Tribbles homolog TRB3 is associated with insulin resistance and related cardiovascular risk in Caucasians from Italy . Diabetes 54 : 2807–2811 [ PubMed ] [ Google Scholar ]
- Prudente S, Scarpelli D, Chandalia M, Zhang YY, Morini E, Del Guerra S, Perticone F, Li R, Powers C, Andreozzi F, et al. 2009. The TRIB3 Q84R polymorphism and risk of early-onset type 2 diabetes . J Clin Endocrinol Metab 94 : 190–196 [ PMC free article ] [ PubMed ] [ Google Scholar ]
- Qi L, Heredia JE, Altarejos JY, Screaton R, Goebel N, Niessen S, Macleod IX, Liew cW, Kulkarni RN, Bain J, et al. 2006. TRB3 links the E3 ubiquitin ligase COP1 to lipid metabolism . Science 312 : 1763–1766 [ PubMed ] [ Google Scholar ]
- Ravichandran LV, Esposito DL, Chen J, Quon MJ 2001. Protein kinase C-ζ phosphorylates insulin receptor substrate-1 and impairs its ability to activate phosphatidylinositol 3-kinase in response to insulin . J Biol Chem 276 : 3543–3549 [ PubMed ] [ Google Scholar ]
- Reyna SM, Ghosh S, Tantiwong P, Meka CS, Eagan P, Jenkinson CP, Cersosimo E, DeFronzo RA, Coletta DK, Sriwijitkamol A, et al. 2008. Elevated toll-like receptor 4 expression and signaling in muscle from insulin-resistant subjects . Diabetes 57 : 2595–2602 [ PMC free article ] [ PubMed ] [ Google Scholar ]
- Riboulet-Chavey A, Pierron A, Durand I, Murdaca J, Giudicelli J, Van OE 2006. Methylglyoxal impairs the insulin signaling pathways independently of the formation of intracellular reactive oxygen species . Diabetes 55 : 1289–1299 [ PubMed ] [ Google Scholar ]
- Rosen P, Nawroth PP, King G, Moller W, Tritschler HJ, Packer L 2001. The role of oxidative stress in the onset and progression of diabetes and its complications: A summary of a Congress Series sponsored by UNESCO-MCBN, the American Diabetes Association and the German Diabetes Society . Diabetes Metab Res Rev 17 : 189–212 [ PubMed ] [ Google Scholar ]
- Roth RA, Beaudoin J 1987. Phosphorylation of purified insulin receptor by cAMP kinase . Diabetes 36 : 123–126 [ PubMed ] [ Google Scholar ]
- Rotter V, Nagaev I, Smith U 2003. Interleukin-6 (IL-6) induces insulin resistance in 3T3-L1 adipocytes and is, like IL-8 and tumor necrosis factor-α, overexpressed in human fat cells from insulin-resistant subjects . J Biol Chem 278 : 45777–45784 [ PubMed ] [ Google Scholar ]
- Rudich A, Tirosh A, Potashnik R, Hemi R, Kanety H, Bashan N 1998. Prolonged oxidative stress impairs insulin-induced GLUT4 translocation in 3T3-L1 adipocytes . Diabetes 47 : 1562–1569 [ PubMed ] [ Google Scholar ]
- Rui L, Yuan M, Frantz D, Shoelson S, White MF 2002. SOCS-1 and SOCS-3 block insulin signaling by ubiquitin-mediated degradation of IRS1 and IRS2 . J Biol Chem 277 : 42394–42398 [ PubMed ] [ Google Scholar ]
- Sachithanandan N, Fam BC, Fynch S, Dzamko N, Watt MJ, Wormald S, Honeyman J, Galic S, Proietto J, Andrikopoulos S, et al. 2010. Liver-specific suppressor of cytokine signaling-3 deletion in mice enhances hepatic insulin sensitivity and lipogenesis resulting in fatty liver and obesity . Hepatology 52 : 1632–1642 [ PubMed ] [ Google Scholar ]
- Sajan MP, Rivas J, Li P, Standaert ML, Farese RV 2006. Repletion of atypical protein kinase C following RNA interference-mediated depletion restores insulin-stimulated glucose transport . J Biol Chem 281 : 17466–17473 [ PubMed ] [ Google Scholar ]
- Sakamoto K, Holman GD 2008. Emerging role for AS160/TBC1D4 and TBC1D1 in the regulation of GLUT4 traffic . Am J Physiol Endocrinol Metab 295 : E29–E37 [ PMC free article ] [ PubMed ] [ Google Scholar ]
- Samuel VT, Liu ZX, Wang A, Beddow SA, Geisler JG, Kahn M, Zhang XM, Monia BP, Bhanot S, Shulman GI 2007. Inhibition of protein kinase Cε prevents hepatic insulin resistance in nonalcoholic fatty liver disease . J Clin Invest 117 : 739–745 [ PMC free article ] [ PubMed ] [ Google Scholar ]
- Samuel VT, Petersen KF, Shulman GI 2010. Lipid-induced insulin resistance: Unravelling the mechanism . Lancet 375 : 2267–2277 [ PMC free article ] [ PubMed ] [ Google Scholar ]
- Sano H, Kane S, Sano E, Miinea CP, Asara JM, Lane WS, Garner CW, Lienhard GE 2003. Insulin-stimulated phosphorylation of a Rab GTPase-activating protein regulates GLUT4 translocation . J Biol Chem 278 : 14599–14602 [ PubMed ] [ Google Scholar ]
- Sarbassov DD, Guertin DA, Ali SM, Sabatini DM 2005. Phosphorylation and regulation of Akt/PKB by the rictor-mTOR complex . Science 307 : 1098–1101 [ PubMed ] [ Google Scholar ]
- Schenk S, Saberi M, Olefsky JM 2008. Insulin sensitivity: Modulation by nutrients and inflammation . J Clin Invest 118 : 2992–3002 [ PMC free article ] [ PubMed ] [ Google Scholar ]
- Schultze SM, Jensen J, Hemmings BA, Tschopp O, Niessen M 2011. Promiscuous affairs of PKB/AKT isoforms in metabolism . Arch Physiol Biochem 117 : 70–77 [ PubMed ] [ Google Scholar ]
- Sciacchitano S, Taylor SI 1997. Cloning, tissue expression, and chromosomal localization of the mouse IRS-3 gene . Endocrinology 138 : 4931–4940 [ PubMed ] [ Google Scholar ]
- Shah OJ, Wang Z, Hunter T 2004. Inappropriate activation of the TSC/Rheb/mTOR/S6K cassette induces IRS1/2 depletion, insulin resistance, and cell survival deficiencies . Curr Biol 14 : 1650–1656 [ PubMed ] [ Google Scholar ]
- Shao J, Catalano PM, Yamashita H, Ruyter I, Smith S, Youngren J, Friedman JE 2000. Decreased insulin receptor tyrosine kinase activity and plasma cell membrane glycoprotein-1 overexpression in skeletal muscle from obese women with gestational diabetes mellitus (GDM): Evidence for increased serine/threonine phosphorylation in pregnancy and GDM . Diabetes 49 : 603–610 [ PubMed ] [ Google Scholar ]
- Shaw LM 2011. The insulin receptor substrate (IRS) proteins: At the intersection of metabolism and cancer . Cell Cycle 10 : 1750–1756 [ PMC free article ] [ PubMed ] [ Google Scholar ]
- Shi H, Kokoeva MV, Inouye K, Tzameli I, Yin H, Flier JS 2006. TLR4 links innate immunity and fatty acid-induced insulin resistance . J Clin Invest 116 : 3015–3025 [ PMC free article ] [ PubMed ] [ Google Scholar ]
- Shiura H, Miyoshi N, Konishi A, Wakisaka-Saito N, Suzuki K, Muguruma R, Kohda T, Wakana S, Yokoyama M, Ishino F, et al. 2005. Meg1/Grb10 overexpression causes postnatal growth retardation and insulin resistance via negative modulation of the IGF1R and IR cascades . Biochem Biophys Res Commun 329 : 909–916 [ PubMed ] [ Google Scholar ]
- Siddle K 2012. Molecular basis of signaling specificity of insulin and IGF receptors: Neglected corners and recent advances . Front Endocrinol (Lausanne) 3 : 34. [ PMC free article ] [ PubMed ] [ Google Scholar ]
- Sinha S, Perdomo G, Brown NF, O'Doherty RM 2004. Fatty acid-induced insulin resistance in L6 myotubes is prevented by inhibition of activation and nuclear localization of nuclear factor κ B . J Biol Chem 279 : 41294–41301 [ PubMed ] [ Google Scholar ]
- Sleeman MW, Wortley KE, Lai KM, Gowen LC, Kintner J, Kline WO, Garcia K, Stitt TN, Yancopoulos GD, Wiegand SJ, et al. 2005. Absence of the lipid phosphatase SHIP2 confers resistance to dietary obesity . Nat Med 11 : 199–205 [ PubMed ] [ Google Scholar ]
- Smith FM, Holt LJ, Garfield AS, Charalambous M, Koumanov F, Perry M, Bazzani R, Sheardown SA, Hegarty BD, Lyons RJ, et al. 2007. Mice with a disruption of the imprinted Grb10 gene exhibit altered body composition, glucose homeostasis, and insulin signaling during postnatal life . Mol Cell Biol 27 : 5871–5886 [ PMC free article ] [ PubMed ] [ Google Scholar ]
- Sopasakis VR, Liu P, Suzuki R, Kondo T, Winnay J, Tran TT, Asano T, Smyth G, Sajan MP, Farese RV, et al. 2010. Specific roles of the p110α isoform of phosphatidylinsositol 3-kinase in hepatic insulin signaling and metabolic regulation . Cell Metab 11 : 220–230 [ PMC free article ] [ PubMed ] [ Google Scholar ]
- Stadtmauer LA, Rosen OM 1986. Increasing the cAMP content of IM-9 cells alters the phosphorylation state and protein kinase activity of the insulin receptor . J Biol Chem 261 : 3402–3407 [ PubMed ] [ Google Scholar ]
- Steppan CM, Wang J, Whiteman EL, Birnbaum MJ, Lazar MA 2005. Activation of SOCS-3 by resistin . Mol Cell Biol 25 : 1569–1575 [ PMC free article ] [ PubMed ] [ Google Scholar ]
- Stiles B, Wang Y, Stahl A, Bassilian S, Lee WP, Kim YJ, Sherwin R, Devaskar S, Lesche R, Magnuson MA, et al. 2004. Liver-specific deletion of negative regulator Pten results in fatty liver and insulin hypersensitivity [corrected] . Proc Natl Acad Sci 101 : 2082–2087 [ PMC free article ] [ PubMed ] [ Google Scholar ]
- Sun XJ, Rothenberg PL, Kahn CR, Backer JM, Araki E, Wilden PA, Cahill DA, Goldstein BJ, White MF 1991. Structure of the insulin receptor substrate IRS-1 defines a unique signal duction protein . Nature 352 : 73–77 [ PubMed ] [ Google Scholar ]
- Sun XJ, Crimmins DL, Myers MR Jr, Miralpeix M, White MF 1993. Pleiotropic insulin signals are engaged by multisite phosphorylation of IRS-1 . Mol Cell Biol 13 : 7418–7428 [ PMC free article ] [ PubMed ] [ Google Scholar ]
- Sun XJ, Wang LM, Zhang Y, Yenush L, Myers MG Jr, Glasheen E, Lane WS, Pierce JH, White MF 1995. Role of IRS-2 in insulin and cytokine signalling . Nature 377 : 173–177 [ PubMed ] [ Google Scholar ]
- Sun K, Kusminski CM, Scherer PE 2011. Adipose tissue remodeling and obesity . J Clin Invest 121 : 2094–2101 [ PMC free article ] [ PubMed ] [ Google Scholar ]
- Suwa A, Kurama T, Shimokawa T 2010. SHIP2 and its involvement in various diseases . Expert Opin Ther Targets 14 : 727–737 [ PubMed ] [ Google Scholar ]
- Tan K, Kimber WA, Luan J, Soos MA, Semple RK, Wareham NJ, O'Rahilly S, Barroso I 2007. Analysis of genetic variation in Akt2/PKB-β in severe insulin resistance, lipodystrophy, type 2 diabetes, and related metabolic phenotypes . Diabetes 56 : 714–719 [ PMC free article ] [ PubMed ] [ Google Scholar ]
- Taniguchi CM, Emanuelli B, Kahn CR 2006a. Critical nodes in signalling pathways: Insights into insulin action . Nat Rev Mol Cell Biol 7 : 85–96 [ PubMed ] [ Google Scholar ]
- Taniguchi CM, Tran TT, Kondo T, Luo J, Ueki K, Cantley LC, Kahn CR 2006b. Phosphoinositide 3-kinase regulatory subunit p85α suppresses insulin action via positive regulation of PTEN . Proc Natl Acad Sci 103 : 12093–12097 [ PMC free article ] [ PubMed ] [ Google Scholar ]
- Taniguchi CM, Winnay J, Kondo T, Bronson RT, Guimaraes AR, Aleman JO, Luo J, Stephanopoulos G, Weissleder R, Cantley LC, et al. 2010. The phosphoinositide 3-kinase regulatory subunit p85α can exert tumor suppressor properties through negative regulation of growth factor signaling . Cancer Res 70 : 5305–5315 [ PMC free article ] [ PubMed ] [ Google Scholar ]
- Taylor SI, Accili D, Cama A, Kadowaki H, Kadowaki T, Imano E, Sierra ML 1991. Mutations in the insulin receptor gene in patients with genetic syndromes of insulin resistance . Adv Exp Med Biol 293 : 197–213 [ PubMed ] [ Google Scholar ]
- Taylor EB, An D, Kramer HF, Yu H, Fujii NL, Roeckl KS, Bowles N, Hirshman MF, Xie J, Feener EP, et al. 2008. Discovery of TBC1D1 as an insulin-, AICAR-, and contraction-stimulated signaling nexus in mouse skeletal muscle . J Biol Chem 283 : 9787–9796 [ PMC free article ] [ PubMed ] [ Google Scholar ]
- Terauchi Y, Tsuji Y, Satoh S, Minoura H, Murakami K, Okuno A, Inukai K, Asano T, Kaburagi Y, Ueki K, et al. 1999. Increased insulin sensitivity and hypoglycaemia in mice lacking the p85 α subunit of phosphoinositide 3-kinase . Nat Genet 21 : 230–235 [ PubMed ] [ Google Scholar ]
- Teruel T, Hernandez R, Lorenzo M 2001. Ceramide mediates insulin resistance by tumor necrosis factor-α in brown adipocytes by maintaining Akt in an inactive dephosphorylated state . Diabetes 50 : 2563–2571 [ PubMed ] [ Google Scholar ]
- Tseng YH, Butte AJ, Kokkotou E, Yechoor VK, Taniguchi CM, Kriauciunas KM, Cypess AM, Niinobe M, Yoshikawa K, Patti ME, et al. 2005. Prediction of preadipocyte differentiation by gene expression reveals role of insulin receptor substrates and necdin . Nat Cell Biol 7 : 601–611 [ PubMed ] [ Google Scholar ]
- Turban S, Hajduch E 2011. Protein kinase C isoforms: Mediators of reactive lipid metabolites in the development of insulin resistance . Growth Regul 585 : 269–274 [ PubMed ] [ Google Scholar ]
- Tzivion G, Dobson M, Ramakrishnan G 2011. FoxO cription factors; Regulation by AKT and 14–3-3 proteins . Biochim Biophys Acta 1813 : 1938–1945 [ PubMed ] [ Google Scholar ]
- Ueki K, Yballe CM, Brachmann SM, Vicent D, Watt JM, Kahn CR, Cantley LC 2002. Increased insulin sensitivity in mice lacking p85β subunit of phosphoinositide 3-kinase . Proc Natl Acad Sci 99 : 419–424 [ PMC free article ] [ PubMed ] [ Google Scholar ]
- Ueki K, Kondo T, Kahn CR 2004a. Suppressor of cytokine signaling 1 (SOCS-1) and SOCS-3 cause insulin resistance through inhibition of tyrosine phosphorylation of insulin receptor substrate proteins by discrete mechanisms . Mol Cell Biol 24 : 5434–5446 [ PMC free article ] [ PubMed ] [ Google Scholar ]
- Ueki K, Kondo T, Tseng YH, Kahn CR 2004b. Central role of suppressors of cytokine signaling proteins in hepatic steatosis, insulin resistance, and the metabolic syndrome in the mouse . Proc Natl Acad Sci 101 : 10422–10427 [ PMC free article ] [ PubMed ] [ Google Scholar ]
- Um SH, Frigerio F, Watanabe M, Picard F, Joaquin M, Sticker M, Fumagalli S, Allegrini PR, Kozma SC, Auwerx J, et al. 2004. Absence of S6K1 protects against age- and diet-induced obesity while enhancing insulin sensitivity . Nature 431 : 200–205 [ PubMed ] [ Google Scholar ]
- Vadas O, Burke JE, Zhang X, Berndt A, Williams RL 2011. Structural basis for activation and inhibition of class I phosphoinositide 3-kinases . Sci Signal 4 : re2. [ PubMed ] [ Google Scholar ]
- van Dam RM, Hoebee B, Seidell JC, Schaap MM, Blaak EE, Feskens EJ 2004. The insulin receptor substrate-1 Gly972Arg polymorphism is not associated with type 2 diabetes mellitus in two population-based studies . Diabet Med 21 : 752–758 [ PubMed ] [ Google Scholar ]
- Versteyhe S, Blanquart C, Hampe C, Mahmood S, Christeff N, De MP, Gray SG, Issad T 2010. Insulin receptor substrates-5 and -6 are poor substrates for the insulin receptor . Mol Med Report 3 : 189–193 [ PubMed ] [ Google Scholar ]
- Voliovitch H, Schindler DG, Hadari YR, Taylor SI, Accili D, Zick Y 1995. Tyrosine phosphorylation of insulin receptor substrate-1 in vivo depends upon the presence of its pleckstrin homology region . J Biol Chem 270 : 18083–18087 [ PubMed ] [ Google Scholar ]
- Wang L, Balas B, Christ-Roberts CY, Kim RY, Ramos FJ, Kikani CK, Li C, Deng C, Reyna S, Musi N, et al. 2007. Peripheral disruption of the Grb10 gene enhances insulin signaling and sensitivity in vivo . Mol Cell Biol 27 : 6497–6505 [ PMC free article ] [ PubMed ] [ Google Scholar ]
- Weisberg SP, Hunter D, Huber R, Lemieux J, Slaymaker S, Vaddi K, Charo I, Leibel RL, Ferrante AW Jr 2006. CCR2 modulates inflammatory and metabolic effects of high-fat feeding . J Clin Invest 116 : 115–124 [ PMC free article ] [ PubMed ] [ Google Scholar ]
- West IC 2000. Radicals and oxidative stress in diabetes . Diabet Med 17 : 171–180 [ PubMed ] [ Google Scholar ]
- Westwick JK, Bielawska AE, Dbaibo G, Hannun YA, Brenner DA 1995. Ceramide activates the stress-activated protein kinases . J Biol Chem 270 : 22689–22692 [ PubMed ] [ Google Scholar ]
- White MF 2006. Regulating insulin signaling and β-cell function through IRS proteins . Can J Physiol Pharmacol 84 : 725–737 [ PubMed ] [ Google Scholar ]
- Wijesekara N, Konrad D, Eweida M, Jefferies C, Liadis N, Giacca A, Crackower M, Suzuki A, Mak TW, Kahn CR, et al. 2005. Muscle-specific Pten deletion protects against insulin resistance and diabetes . Mol Cell Biol 25 : 1135–1145 [ PMC free article ] [ PubMed ] [ Google Scholar ]
- Winnay JN, Boucher J, Mori MA, Ueki K, Kahn CR 2010. A regulatory subunit of phosphoinositide 3-kinase increases the nuclear accumulation of X-box-binding protein-1 to modulate the unfolded protein response . Nat Med 16 : 438–445 [ PMC free article ] [ PubMed ] [ Google Scholar ]
- Withers DJ, Gutierrez JS, Towery H, Burks DJ, Ren JM, Previs S, Zhang Y, Bernal D, Pons S, Shulman Gi, et al. 1998. Disruption of IRS-2 causes type 2 diabetes in mice . Nature 391 : 900–904 [ PubMed ] [ Google Scholar ]
- Wong JT, Kim PT, Peacock JW, Yau TY, Mui AL, Chung SW, Sossi V, Doudet D, Green D, Ruth TJ, et al. 2007. Pten (phosphatase and tensin homologue gene) haploinsufficiency promotes insulin hypersensitivity . Diabetologia 50 : 395–403 [ PMC free article ] [ PubMed ] [ Google Scholar ]
- Wong RH, Chang I, Hudak CS, Hyun S, Kwan HY, Sul HS 2009. A role of DNA-PK for the metabolic gene regulation in response to insulin . Cell 136 : 1056–1072 [ PMC free article ] [ PubMed ] [ Google Scholar ]
- Xia P, Inoguchi T, Kern TS, Engerman RL, Oates PJ, King GL 1994. Characterization of the mechanism for the chronic activation of diacylglycerol-protein kinase C pathway in diabetes and hypergalactosemia . Diabetes 43 : 1122–1129 [ PubMed ] [ Google Scholar ]
- Yamaguchi H, Wang HG 2001. The protein kinase PKB/Akt regulates cell survival and apoptosis by inhibiting Bax conformational change . Oncogene 20 : 7779–7786 [ PubMed ] [ Google Scholar ]
- Yi Z, Langlais P, De Filippis EA, Luo M, Flynn CR, Schroeder S, Weintraub ST, Mapes R, Mandarino LJ 2007. Global assessment of regulation of phosphorylation of insulin receptor substrate-1 by insulin in vivo in human muscle . Diabetes 56 : 1508–1516 [ PubMed ] [ Google Scholar ]
- Yu J, Zhang Y, McIlroy J, Rordorf-Nikolic T, Orr GA, Backer JM 1998. Regulation of the p85/p110 phosphatidylinositol 3′-kinase: Stabilization and inhibition of the p110α catalytic subunit by the p85 regulatory subunit . Mol Cell Biol 18 : 1379–1387 [ PMC free article ] [ PubMed ] [ Google Scholar ]
- Yu C, Chen Y, Zong H, Wang Y, Bergeron R, Kim JK, Cline GW, Cushman SW, Cooney GJ, Atcheson B, et al. 2002. Mechanism by which fatty acids inhibit insulin activation of IRS-1 associated phosphatidylinositol 3-kinase activity in muscle . J Biol Chem 277 : 50230–50236 [ PubMed ] [ Google Scholar ]
- Yu Q, Gao F, Ma XL 2011a. Insulin says NO to cardiovascular disease . Cardiovasc Res 89 : 516–524 [ PubMed ] [ Google Scholar ]
- Yu Y, Yoon SO, Poulogiannis G, Yang Q, Ma XM, Villen J, Kubica N, Hoffman GR, Cantley LC, Gygi SP, et al. 2011b. Phosphoproteomic analysis identifies Grb10 as an mTORC1 substrate that negatively regulates insulin signaling . Science 332 : 1322–1326 [ PMC free article ] [ PubMed ] [ Google Scholar ]
- Yuan M, Konstantopoulos N, Lee J, Hansen L, Li ZW, Karin M, Shoelson SE 2001. Reversal of obesity- and diet-induced insulin resistance with salicylates or targeted disruption of Ikkβ . Science 293 : 1673–1677 [ PubMed ] [ Google Scholar ]
- Zhang D, Liu ZX, Choi CS, Tian L, Kibbey R, Dong J, Cline GW, Wood PA, Shulman GI 2007. Mitochondrial dysfunction due to long-chain Acyl-CoA dehydrogenase deficiency causes hepatic steatosis and hepatic insulin resistance . Proc Natl Acad Sci 104 : 17075–17080 [ PMC free article ] [ PubMed ] [ Google Scholar ]
- Zhang J, Gao Z, Yin J, Quon MJ, Ye J 2008a. S6K directly phosphorylates IRS-1 on Ser-270 to promote insulin resistance in response to TNF-α signaling through IKK2 . J Biol Chem 283 : 35375–35382 [ PMC free article ] [ PubMed ] [ Google Scholar ]
- Zhang X, Zhang G, Zhang H, Karin M, Bai H, Cai D 2008b. Hypothalamic IKK-β/NF-κB and ER stress link overnutrition to energy imbalance and obesity . Cell 135 : 61–73 [ PMC free article ] [ PubMed ] [ Google Scholar ]
- Zhang X, Vadas O, Perisic O, Anderson KE, Clark J, Hawkins PT, Stephens LR, Williams RL 2011. Structure of lipid kinase p110β/p85β elucidates an unusual SH2-domain-mediated inhibitory mechanism . Mol Cell 41 : 567–578 [ PMC free article ] [ PubMed ] [ Google Scholar ]
- Zhou Q, Dolan PL, Dohm GL 1999. Dephosphorylation increases insulin-stimulated receptor kinase activity in skeletal muscle of obese Zucker rats . Mol Cell Biochem 194 : 209–216 [ PubMed ] [ Google Scholar ]
- Zhou BP, Liao Y, Xia W, Zou Y, Spohn B, Hung MC 2001. HER-2/neu induces p53 ubiquitination via Akt-mediated MDM2 phosphorylation . Nat Cell Biol 3 : 973–982 [ PubMed ] [ Google Scholar ]
- Zisman A, Peroni OD, Abel ED, Michael MD, Mauvais-Jarvis F, Lowell BB, Wojtaszewski JF, Hirshman MF, Virkamaki A, Goodyear LJ, et al. 2000. Targeted disruption of the glucose transporter 4 selectively in muscle causes insulin resistance and glucose intolerance . Nat Med 6 : 924–928 [ PubMed ] [ Google Scholar ]
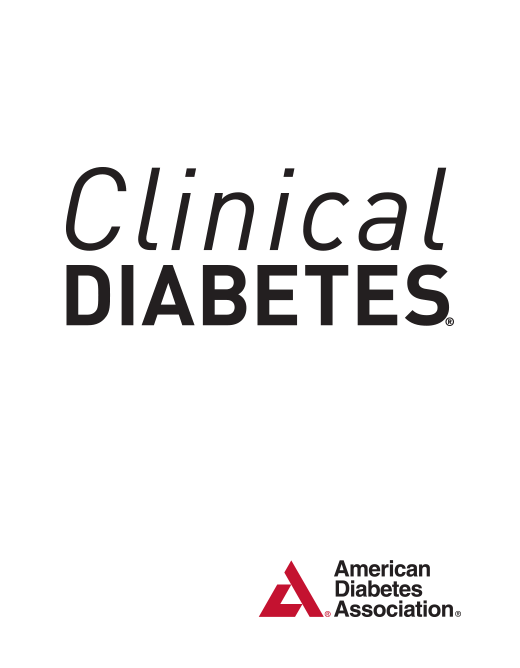
- Previous Article
- Next Article
Case Study 1
Case study 2, case study 3, case study 4, case study 5, case studies 6 and 7, case studies in insulin therapy: the last arrow in the treatment quiver.
The authors are from the Department of Endocrinology at the Institute of Post Graduate Medical Education & Research in Kolkata, West Bengal, India. Anubhav Thukral, MD, Chitra Selvan, MD, Partha Pratim Chakraborty, MD, Ajitesh Roy, MD, Soumik Goswami, MD, and Rana Bhattacharjee, MD, are postdoctoral trainees. Sujoy Ghosh, DM, is an assistant professor; Satinath Mukherjee, DM, is a professor; and Subhankar Chowdhury, DM, is a professor and head of the department.
- Split-Screen
- Article contents
- Figures & tables
- Supplementary Data
- Peer Review
- Open the PDF for in another window
- Cite Icon Cite
- Get Permissions
Anubhav Thukral , Chitra Selvan , Partha Pratim Chakraborty , Ajitesh Roy , Soumik Goswami , Rana Bhattacharjee , Sujoy Ghosh , Satinath Mukherjee , Subhankar Chowdhury; Case Studies in Insulin Therapy: The Last Arrow in the Treatment Quiver. Clin Diabetes 1 October 2013; 31 (4): 175–178. https://doi.org/10.2337/diaclin.31.4.175
Download citation file:
- Ris (Zotero)
- Reference Manager
B ecause type 2 diabetes is a progressive disease, its natural course requires initiation of insulin in a significant proportion of patients, especially when oral agents fail to achieve glycemic targets. Most practicing physicians and endocrinologists acknowledge that neither the transition to nor the journey with insulin treatment is often as smooth as one would hope. Most have occasionally met with patients who plead for help in controlling their blood glucose levels with oral drugs alone. Often, doctors struggle to persuade such patients to start insulin.
In this article, we share some of our experiences—some common throughout the world and others unique to our country—in caring for patients who are prescribed insulin. It is important to learn from these experiences with insulin because it represents, more often than not, the last arrow in our treatment quiver.
The patient was a 45-year-old man who has had type 2 diabetes for the past 6 years and had been taking insulin for the past 2 years. His body weight was 50 kg (BMI 24 kg/m 2 ). He presented with uncontrolled and recently increased blood glucose levels and a dramatic increase in insulin dose during the past 5 months without any apparent cause. He had no history of fever, infections, or steroid use. He had had multiple hospital visits during the past few months.
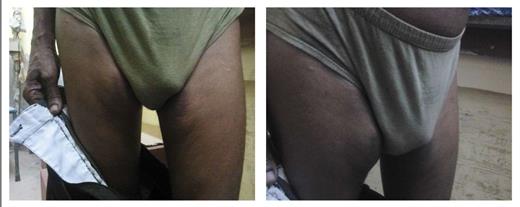
Discoloration with hypertrophy on medial aspects of both thighs on the site where the patient described in Case Study 1 was injecting insulin.
The patient appeared to be well educated and concerned about this situation. To our surprise, however, we found he was injecting insulin on the medial aspect of his thighs. Examination showed lipohypertrophy with swelling in that region ( Figure 1 ). The crowded clinic with its lack of trained diabetes educators and nurses was the perfect setting for this gross oversight in checking injection sites.
The patient was a 55-year-old obese (BMI 28 kg/m 2 ) man with known type 2 diabetes for 12 years who had been taking insulin for the past 6 months. He presented with haywire blood glucose levels over several visits, with gradually increasing doses of insulin. There were no documented episodes of hypoglycemia.
The patient was injecting his own insulin, and his injection technique was confirmed to be good. The insulin injection sites were healthy. Insulin was properly stored at his home, and he was using a 40 IU syringe and a compatible vial.
Surprisingly, his A1C level was 7.2%, which was discordant with his venous blood glucose readings. He was not performing self-monitoring of blood glucose at home because of logistical issues. What was perplexing was that, although his dose of premixed insulin before breakfast had increased from 12 to 36 units in the past 6 months, his morning fasting levels were controlled with a predinner dose of 6 units, and although his post-breakfast values crept up from 200 to 340 mg/dl during the past four visits, his fasting blood glucose had decreased from 98 to 66 mg/dl in the same time period.
Through detailed questioning, we learned that dietary irregularities on the days of the test were at the heart of the matter. On a normal day, the patient injected insulin at about 7:40 a.m., ate breakfast at 8:00 a.m., and ate lunch at noon. However, on the days of the test, he had an extended fast, skipping breakfast. He then performed SMBG and took insulin at noon and, instead of a normal lunch, ended up overeating with a large meal essentially comprising both breakfast and lunch. Hence, his insulin dose was always insufficient, and his postprandial glucose values were always high. This would not have happened if the test center had been closer to his house or had opened at 8:00 a.m., which would have allowed him the flexibility to follow his normal dietary routine on test days.
The patient was asked to divide his meals, take voglibose before breakfast and lunch, and perform SMBG at the proper time. We split his doses, with 16 units of regular insulin before breakfast and 16 units before lunch and 6 units of premixed insulin before dinner. His blood glucose was then controlled.
A man with type 2 diabetes who had been taking insulin for the past 4 years with good glycemic control was started on twice-daily premixed insulin with a pen device for the past month. Although he was happy with the convenience and ease of using a pen device, his blood glucose levels became erratic. We decided to confirm his injection technique and asked him to bring his pen device to the clinic. He brought along a pen device with the cartridge in place, but the needle was missing. On questioning, we learned that the patient was injecting insulin without a needle. He thought it normal that he would not feel anything because pen devices were supposed to be painless. In actuality, he was trying to give himself insulin transdermally rather than subcutaneously. This case illustrates that a combination of less-than-adequate education and aggressive marketing by device sales companies can lead to misconceptions on the part of patients.
A woman with type 2 diabetes who had been taking insulin for the past 6 years with reasonable glycemic control presented with high glucose levels and injection site abscesses for the past month ( Figure 2 ). Through thoughtful questioning, we learned that she had been wiping the insulin injection tip with a cotton swab before and after injecting.
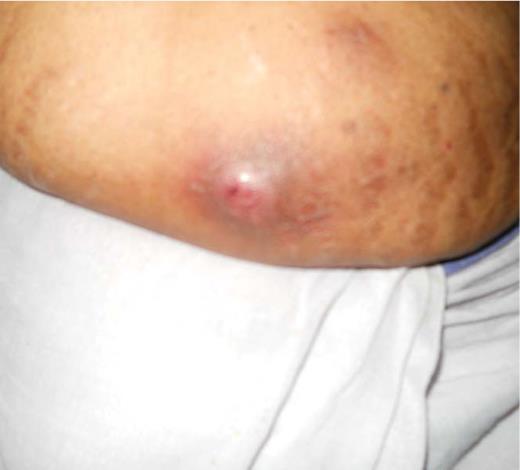
Insulin site abscess in patient described in Case Study 4.
A 46-year-old man with type 2 diabetes started noticing nodular swellings and subsequent discharging sinuses from these swellings at the insulin injection site for the past 6 months ( Figure 3 ). These swellings continued to appear when he took insulin from a particular vial. When that vial got finished, new lesions did not appear.
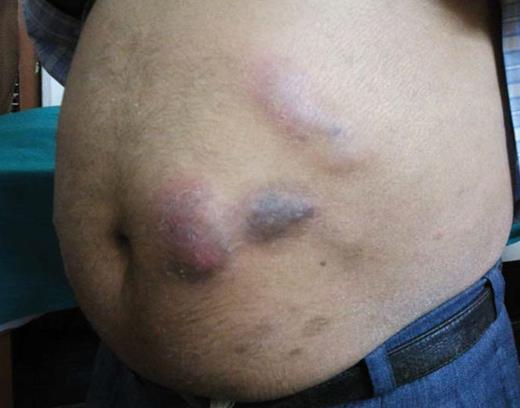
Chronic granulomatous infection at injection site in patient described in Case Study 5.
Patient was treated with multiple courses of different antibiotics with minimal response. Grams stain and multiple bacterial cultures performed on the discharge were negative for any growth. A fine needle aspiration cytology was positive for acid-fast bacilli. The patient refused a biopsy. He was started on anti-tubercular treatment with which the lesions began to regress.
The patient denied having used an insulin syringe that was used earlier for Bacillus Calmette-Guérin vaccination. However, he was sure that a particular vial of insulin was associated with these lesions because no new lesions appeared after he changed vials. Unfortunately, the vial in question could not be retrieved.
The patient was thoroughly evaluated but was not found to have any tubercular foci elsewhere in the body. Although tuberculosis is endemic in our country, tuberculosis at insulin injection sites caught us by surprise. There are no similar case reports or descriptions, although we did find case reports from Columbia of cutaneous tuberculosis after subcutaneous injections for cosmetic mesotherapy. 1
A young woman with diabetes and uncontrolled blood glucose was found to be injecting insulin into her forearm ( Figure 4 ), and yet another man was found to be injecting insulin intradermally on his thigh with residual post-inflammatory hyperpigmentation ( Figure 5 ).
What are some of the causes of insulin therapy failure?
How can clinicians be vigilant in identifying patients with nonadherence to or problems carrying out their insulin regimen?
What are the consequences of nonadherence to insulin therapy?
How can we ensure better adherence to insulin therapy?
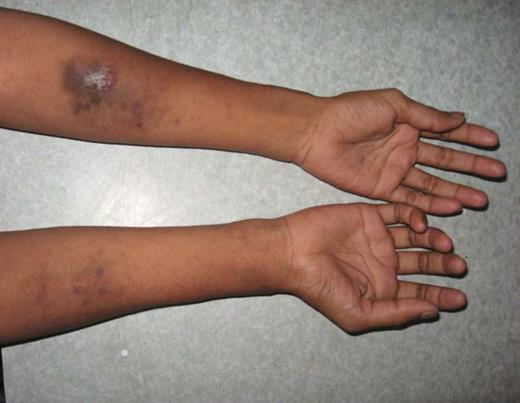
Forearm injection sites of the female patient described in Case Study 6.
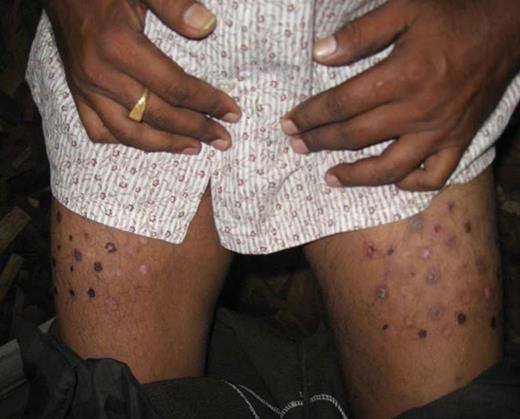
Post-inflammatory hyperpigmentation in the thighs of the male patient described in Case Study 7.
Incomplete or incorrect initial education and a lack of continuing education are at the root of all of the cases reported above. Apart from the obvious effects they have on glycemic status, incidents such as these leave an indelible trauma in the minds of patients and act as strong deterrents to continuing insulin therapy.
Doctors and patients expend great effort to start insulin, and the manner in which we use this last arrow in our treatment quiver matters. In a busy outpatient department, the easiest thing to do for patients with uncontrolled blood glucose levels is simply to increase the dose of insulin. However, this not only destroys patients' confidence in insulin, but also leads to decreasing self-confidence and depression in patients on insulin. Hence, it would seem prudent and rewarding to devote some time to educating patients and using every visit to reinforce their knowledge and verify their insulin therapy practices. Logistical issues and test conditions need to be factored in when interpreting blood glucose data. This also highlights the importance of the role of diabetes educators and paramedical staff in care of diabetes patients. Unfortunately, these professionals and the services they provide are missing in most diabetes clinics in underdeveloped or developing countries, where the gap between the disease burden and the availability of care providers is widening rapidly.
Recent reviews on insulin adherence in Western countries show adherence rates as low as 62–64%. 2 Estimates of diabetes medication adherence in our country (India) are even worse, especially in populations in which illiteracy and poverty levels are very high. Nonadherence rates as high as 74% (95% CI 69.2–78.3) have been reported from south India. 3
Insulin nonadherence has been shown to be a significant risk factor for increased mortality and increased costs of therapy in the diabetic population. 4 , 5 Many factors determine adherence to insulin. Misinformation and miscommunication between doctors and patients is one of the foremost causes. Illness and treatment perceptions have also been shown to be an important determinant of adherence. 6 Among various suggestions to improve treatment adherence is a participatory model in which patients are made an integral part of decision-making. This has been shown to improve adherence to insulin and antidiabetic drugs and, hence, to improve outcomes. 7
All insulin-requiring patients must learn to position the needle perpendicular to their skin when injecting insulin, rotate injection sites, discard syringes after a single use, inject at appropriate sites, inject subcutaneously into fat, count to 10 after injections, store insulin in a cool and airy place away from direct sunlight, and discard open vials after 1 month or the prescribed shelf life indicated on the vial. 8 Apart from this basic education, they should be taught methods that may reduce the pain of injection, such as allowing the insulin to come to room temperature before injecting, making sure there are no air bubbles in the syringe, and keeping underlying muscles relaxed. This information may help to boost patients' morale. 8
A change in temperature can change the concentration of insulin in the cartridge of a pen device. 9 Hence, it is recommended to remove the needle from the pen immediately after use so the temperature of the insulin is not affected by the leak in thermo insulation caused by the attached needle. 10 Another common mistake is using a 100 IU/ml pen cartridge but injecting insulin through a 40 IU/ml syringe. Patients also should be educated about the color coding of syringes and vials of different insulin concentrations (e.g., a red syringe is 40 IU/ml, whereas an orange syringe is 100 IU/ml).
Although the cases described here expose our shortcomings in diabetes care, highlighting such unusual clinical scenarios might alert other health care providers to recognize similar instances in their practice and enable better outcomes from insulin therapy.
CLINICAL PEARLS
Unexplained deterioration in glycemic control and discrepancies between plasma glucose, SMBG values, and A1C test results should alert physicians to the possibility of patients' nonadherence with or poor practices in carrying out their insulin regimen.
Nonadherence is associated with a significantly higher mortality, higher complication rates, low self-confidence, poor self-image, and adverse disease-related perceptions.
In addition to patient illiteracy and poverty, failure on the part of the health care system to ensure a proper, planned, and staged patient education and confidence-building program before instituting insulin therapy is a major barrier to successful insulin therapy.
A few hours spent by physicians, nurses, or other diabetes educators with patients initiating insulin may save many years of life for these patients.
Email alerts
- Online ISSN 1945-4953
- Print ISSN 0891-8929
- Diabetes Care
- Clinical Diabetes
- Diabetes Spectrum
- Standards of Medical Care in Diabetes
- Scientific Sessions Abstracts
- BMJ Open Diabetes Research & Care
- ShopDiabetes.org
- ADA Professional Books
Clinical Compendia
- Clinical Compendia Home
- Latest News
- DiabetesPro SmartBrief
- Special Collections
- DiabetesPro®
- Diabetes Food Hub™
- Insulin Affordability
- Know Diabetes By Heart™
- About the ADA
- Journal Policies
- For Reviewers
- Advertising in ADA Journals
- Reprints and Permission for Reuse
- Copyright Notice/Public Access Policy
- ADA Professional Membership
- ADA Member Directory
- Diabetes.org
- X (Twitter)
- Cookie Policy
- Accessibility
- Terms & Conditions
- Get Adobe Acrobat Reader
- © Copyright American Diabetes Association
This Feature Is Available To Subscribers Only
Sign In or Create an Account
Thank you for visiting nature.com. You are using a browser version with limited support for CSS. To obtain the best experience, we recommend you use a more up to date browser (or turn off compatibility mode in Internet Explorer). In the meantime, to ensure continued support, we are displaying the site without styles and JavaScript.
- View all journals
- My Account Login
- Explore content
- About the journal
- Publish with us
- Sign up for alerts
- Open access
- Published: 16 April 2024
Placental IGFBP1 levels during early pregnancy and the risk of insulin resistance and gestational diabetes
- Marie-France Hivert ORCID: orcid.org/0000-0001-7752-2585 1 , 2 , 3 ,
- Frédérique White 4 ,
- Catherine Allard ORCID: orcid.org/0000-0002-8829-4984 3 ,
- Kaitlyn James 5 ,
- Sana Majid 1 ,
- François Aguet 6 ,
- Kristin G. Ardlie 6 ,
- Jose C. Florez ORCID: orcid.org/0000-0002-1730-9325 2 , 6 , 7 , 8 ,
- Andrea G. Edlow ORCID: orcid.org/0000-0003-2915-5949 5 ,
- Luigi Bouchard 3 , 9 , 10 ,
- Pierre-Étienne Jacques 3 , 4 , 11 ,
- S. Ananth Karumanchi ORCID: orcid.org/0000-0002-2281-6831 12 &
- Camille E. Powe 2 , 5 , 6 , 8
Nature Medicine ( 2024 ) Cite this article
1872 Accesses
113 Altmetric
Metrics details
- Gene expression
- Gestational diabetes
- Predictive markers
Reduced insulin sensitivity (insulin resistance) is a hallmark of normal physiology in late pregnancy and also underlies gestational diabetes mellitus (GDM). We conducted transcriptomic profiling of 434 human placentas and identified a positive association between insulin-like growth factor binding protein 1 gene ( IGFBP1 ) expression in the placenta and insulin sensitivity at ~26 weeks gestation. Circulating IGFBP1 protein levels rose over the course of pregnancy and declined postpartum, which, together with high gene expression levels in our placenta samples, suggests a placental or decidual source. Higher circulating IGFBP1 levels were associated with greater insulin sensitivity (lesser insulin resistance) at ~26 weeks gestation in the same cohort and in two additional pregnancy cohorts. In addition, low circulating IGFBP1 levels in early pregnancy predicted subsequent GDM diagnosis in two cohorts of pregnant women. These results implicate IGFBP1 in the glycemic physiology of pregnancy and suggest a role for placental IGFBP1 deficiency in GDM pathogenesis.
Similar content being viewed by others
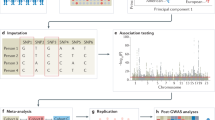
Genome-wide association studies
Emil Uffelmann, Qin Qin Huang, … Danielle Posthuma
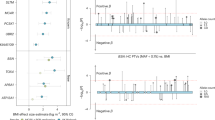
Protein-truncating variants in BSN are associated with severe adult-onset obesity, type 2 diabetes and fatty liver disease
Yajie Zhao, Maria Chukanova, … John R. B. Perry
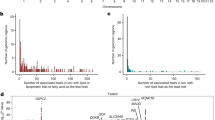
Genome-wide characterization of circulating metabolic biomarkers
Minna K. Karjalainen, Savita Karthikeyan, … Johannes Kettunen
Gestational diabetes mellitus (GDM) affects one in seven pregnancies worldwide 1 and is associated with maternal and offspring adverse health outcomes during pregnancy, at delivery and over the life course 2 . Previous research has established that a defect in insulin sensitivity (that is, excess insulin resistance) contributes to GDM 3 , 4 . In addition, we and others 5 , 6 , 7 , 8 , 9 , 10 have shown previously that, among individuals with GDM, those with the lowest insulin sensitivity (insulin-resistant GDM) have the greatest risk of hyperglycemia-associated pregnancy complications, suggesting that reduced insulin sensitivity is a key contributor not only to GDM itself, but also to the negative health outcomes that accompany it.
The placenta is the main driver of marked changes in insulin physiology during pregnancy, including the drastic decline in insulin sensitivity, which occurs even in those without GDM. This has been attributed to hormonal factors released by the placenta that lead to insulin resistance 11 . The specific placental circulating factors that mediate the profound change in insulin sensitivity during pregnancy are still unknown, and the classically implicated pregnancy hormones (for example, human chorionic gonadotropin, human placental lactogen and placental growth hormone) have been found to correlate poorly with insulin sensitivity in pregnancy in human studies 12 . A better understanding of the placental factors driving the pregnancy-related decline in insulin sensitivity could lead to new therapeutic approaches to hyperglycemia, early identification of those at risk of developing GDM and recognition of those most likely to have GDM-related pregnancy complications. Despite the hallmark reduction in insulin sensitivity in all pregnancies, most pregnant individuals do not develop GDM. This phenomenon suggests that additional factors, as yet unknown, may contribute to the maintenance of euglycemia in pregnancy. Indeed, a variable improvement in insulin sensitivity has been reported in early pregnancy in several studies 13 , 14 , 15 . A systematic search for placental factors that are related to insulin sensitivity in pregnancy may also uncover those that improve it.
The overarching goal of this study was to discover new placental factors implicated in physiologic changes in insulin sensitivity during pregnancy and that contribute to GDM pathophysiology. We conducted a placental genome-wide transcriptomic study using RNA sequencing (RNA-seq) to identify genes whose expression in the placenta was associated with insulin sensitivity in pregnancy. We identified the insulin-like growth factor binding protein 1 gene ( IGFBP1 ) as the most strongly associated placental transcript. IGFBP1 is a binding protein that is produced primarily by the liver outside of pregnancy and is highly expressed by the placenta 16 . IGFBP1 has been implicated in the modulation of the biological activity of insulin-like growth factor (IGF)-1 and IGF-2, which are key regulators of growth and metabolism in postnatal and fetal life 17 . Outside of pregnancy, low IGFBP1 concentrations are correlated with insulin resistance and associated with risk of incident type 2 diabetes (T2D) 18 , 19 , 20 but this has not been shown in pregnancy. In the current study, using plasma samples collected from three different pregnancy cohorts with diverse backgrounds, we measured circulating IGFBP1 protein levels at several time points during and after gestation. Using these data, we investigated associations between circulating IGFBP1 levels and insulin sensitivity, other pregnancy-related metabolic traits, birth anthropometric measurements and risk of GDM.
Participants in the placental genome-wide RNA-seq analyses
A genome-wide RNA-seq study was conducted using placental samples collected from 434 participants in the Genetic of Glucose regulation in Gestation and Growth (Gen3G) prospective pregnancy cohort 21 (Table 1 ). At study entry (median, 9 weeks gestation), participants’ mean ± s.d. age was 28.7 ± 4.4 years, and median interquartile range (IQR) body mass index (BMI) was 23.8 (21.4–27.9) kg m −2 . Individuals with diabetes present before pregnancy were excluded. Participants underwent a fasting 75 g oral glucose tolerance (75g-OGTT) in the late second trimester (median, 26 weeks gestation), during which extra plasma samples were collected to measure glucose and insulin levels at several time points to estimate insulin sensitivity (using the Matsuda index, which has been validated previously against euglycemic clamps in pregnancy 22 ). At delivery (median (IQR) = 39.6 (38.7–40.3) weeks), trained research staff collected samples from the maternal-facing side of the placenta using standardized protocols for collection and storage for future analyses by RNA-seq ( Methods ).
Differential placental RNA expression and insulin sensitivity
After processing and quality control (QC) of the placental RNA-seq dataset, differential expression of 15,202 genes were analyzed in relation to insulin sensitivity (Matsuda index, log 2 transformed) in late second trimester. We identified 14 genes whose placental RNA expression levels were associated with insulin sensitivity ( P < 1.0 × 10 −3 ; Extended Data Table 1 ) after accounting for technical variability (37 surrogate variables (SV)), precision variables (gestational age at delivery, fetal sex) and potential confounders (gravidity, maternal age and BMI) using multivariate linear regression models. The strongest association was found between insulin sensitivity and the IGFBP1 gene ( β = 0.43; P = 2.5 × 10 −5 ), where higher placental expression levels were associated with greater insulin sensitivity (Fig. 1 ). No strong associations were observed between the Matsuda index and other genes in IGF-related pathways, or genes encoding classic pregnancy-specific placental hormones (for example, human placental growth hormone ( GH2 ), human placental lactogen ( CSH1 ), prolactin ( PRL )) or genes encoding inflammatory proteins secreted by the placenta that have been found previously to be associated with insulin sensitivity in pregnancy 12 (Extended Data Table 2 ); some of the classic placental hormones ( CSH1 , GH2 ) had mappability scores <0.8, so these results should be interpreted with caution.
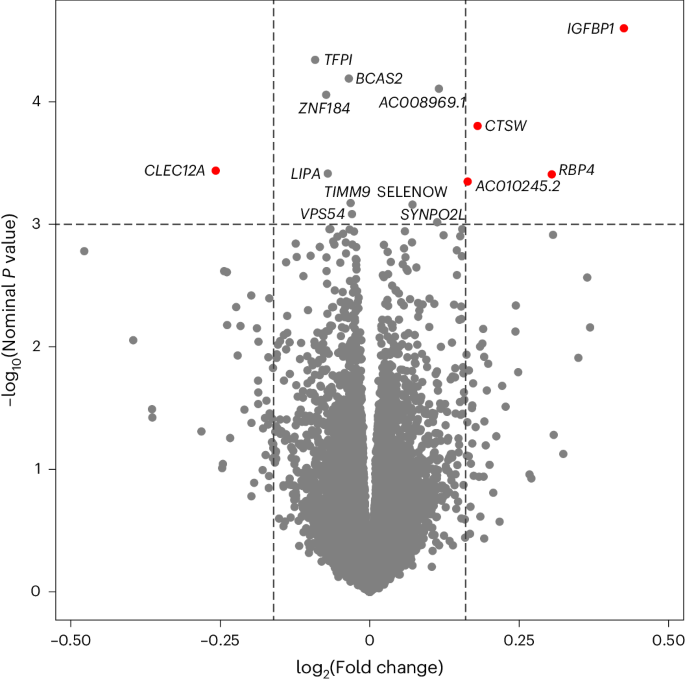
Linear model adjusted for maternal age, gravidity and maternal BMI at first trimester visit, sex of offspring, gestational age at delivery and 37 SVs (from SmartSVA package); gene names identified if P < 1.0 × 10 −3 . Red dots indicate genes with association P < 1.0 × 10 −3 (horizontal dotted line) and absolute log 2 fold changes greater than 5 s.d. from the mean (vertical dotted lines).
Circulating IGFBP1 levels during pregnancy
Given the high levels of placental expression of IGFBP1 (average transcript per million = 103.4) and its known secreted protein status, circulating levels of IGFBP1 (R&D systems enzyme-linked immunosorbent assay, catalog number DGB100) were measured in Gen3G participants ( n = 837; Extended Data Table 3 ). Circulating levels of IGFBP1 were correlated with placental RNA expression of IGFBP1 (Pearson r = 0.15; P = 0.002 with IGFBP1 levels at V1; r = 0.14; P = 0.005 at V2; adjusted for gestational age at each visit). Furthermore, circulating levels of IGFBP1 were measured in two additional pregnancy cohorts: the Study of Pregnancy Regulation of Insulin and Glucose (SPRING) 15 and the Massachusetts General Hospital (MGH) Obstetrical Maternal Study (MOMS). Characteristics of participants included in SPRING and MOMS are presented in Extended Data Table 4 ).
In SPRING participants who remained normoglycemic throughout pregnancy ( n = 65), the median plasma levels of IGFBP1 rose between the first trimester (66,610 pg ml −1 ) and 24 to 32 weeks gestation (79,379 pg ml −1 ), then declined dramatically postpartum (16,588 pg ml −1 ; paired t -tests P < 0.001 for differences between plasma levels across pregnancy and postpartum; Extended Data Fig. 1 ). This pattern, combined with high placental expression levels, suggests a possible placental origin of high circulating IGFBP1 levels during pregnancy.
In a subset of Gen3G participants ( n = 27) in whom we assayed serial IGFBP1 levels during the 75g-OGTT (Extended Data Fig. 2 ), circulating IGFPB1 levels were stable over the first hour of the OGTT (median levels, fasting = 87,008 pg ml −1 ; 1 h postload = 91,485 pg ml −1 ; paired t -test P = 0.13), but declined 2 h postglucose-load (median = 60,920 pg ml −1 ; paired t -test P = 0.0007 compared with fasting). The change in plasma insulin levels from baseline to 1 h (delta insulin 0–60 min) appeared to be associated inversely with the IGFBP1 levels at 1 h ( r = −0.39; P = 0.047) and at 2 h ( r = −0.31; P = 0.11) during the OGTT. This is consistent with the known negative feedback regulation of IGFBP1 expression by insulin, albeit shown previously only in hepatocytes 23 .
Circulating IGFBP1 and insulin sensitivity in pregnancy
Higher plasma IGFBP1 levels were associated with greater insulin sensitivity in all three pregnancy cohorts examined (Table 2 ). The strong positive correlations (Pearson r = 0.5 to 0.6; P < 0.001) between plasma IGFBP1 levels and insulin sensitivity were consistent across different periods of pregnancy, as well as in the postpartum period (SPRING). Adjusting for maternal age and gestational age at the time of blood sampling did not influence correlations. The strength of association was attenuated modestly by further adjustment for maternal BMI, but remained highly statistically significant ( r = 0.34–0.48, P < 0.001; Table 2 ).
In Gen3G, correlations between plasma IGFBP1 (in the first and second trimester) and various maternal metabolic traits and neonatal anthropometric measures were assessed using Pearson correlations (Extended Data Table 5 ). Higher maternal BMI was associated with lower plasma IGFBP1 in the first trimester ( r = −0.27) and in the late second trimester ( r = −0.54; both P < 0.001). Plasma IGFBP1 in the late second trimester was correlated negatively with glucose ( r = −0.28 to −0.30) and insulin levels ( r = −0.40) during the OGTT (all P < 0.001). Lower IGFBP1 levels at both time points were also associated with higher birthweight z -scores (standardized for gestational age and sex) at delivery ( r = −0.15 and r = −0.21 for IGFBP1 at the first and second trimester visits, respectively; both P < 0.001; Extended Data Table 5 ). Adjusting for maternal BMI or for maternal glucose reduced the strength of associations, but the correlations remained statistically significant (for example, second trimester IGFBP1 partial correlations with birthweight z -score adjusted for maternal BMI r = −0.12; P < 0.001; or adjusted for maternal glucose (glucose area under the curve (AUC) during the OGTT) r = −0.17; P < 0.001). Lower IGFBP1 at the second trimester visit was associated with higher risk of large-for-gestational (LGA) birthweight (odds ratio (OR) = 0.60 (95% confidence interval (CI) = 0.46–0.78); P = 0.0001); this association was reduced but remained statistically significant after adjustment for maternal BMI (OR = 0.73 (95% CI = 0.54–0.99); P = 0.045).
Early pregnancy circulating IGFBP1 and GDM incidence
Prediction analyses were conducted using plasma IGFBP1 measured in early pregnancy (median 9 weeks gestation) and GDM (diagnosed with International Association of the Diabetes and Pregnancy Study Groups (IADPSG) criteria applied to a 75g-OGTT at a median of 26 weeks gestation) in Gen3G participants ( n = 837) in addition to known clinical risk factors. Overall, 70 participants (8.4%) developed GDM (Extended Data Table 3 ). Early pregnancy IGFBP1 levels alone predicted risk of incident GDM with a modest receiver operating characteristic (ROC) AUC value of 0.64. A model including only clinical variables (maternal age, gravidity, family history of diabetes, maternal BMI, gestational week at blood sampling) without IGFBP1 levels yielded an ROC AUC of 0.66 (Fig. 2 ). A model with the same clinical variables but also incorporating early pregnancy IGFBP1 levels improved predictive ability (ROC AUC = 0.72 compared with 0.66; P = 0.008; Fig. 2 ). Using a logistic regression model, a 1 s.d. increase in plasma IGFBP1 levels in early pregnancy was associated with a greater than 50% reduction in the risk for GDM in Gen3G (OR = 0.44; IQR = 0.30–0.64; P < 0.001; adjusted for maternal age, gravidity, gestational age at plasma IGFBP1 measurements and maternal BMI; Table 3 ).
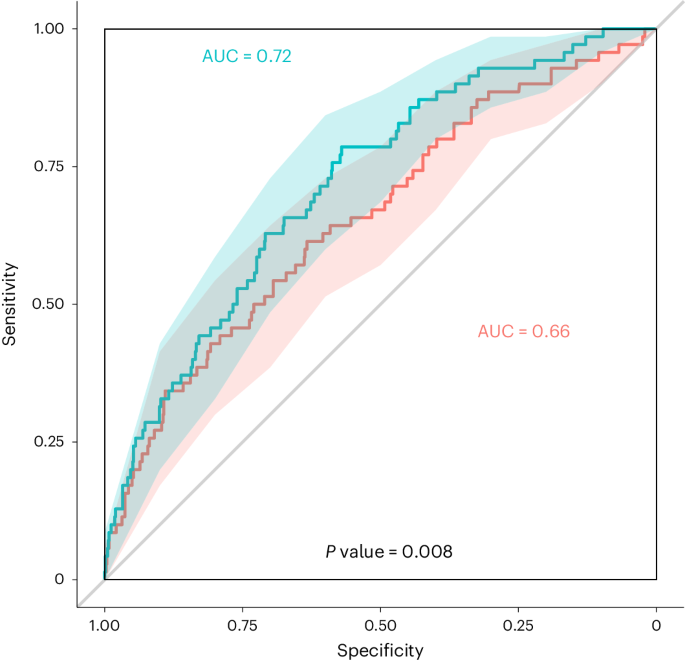
Red line (clinical variables only): ROC curve for GDM diagnosis based on maternal age, gravidity, family history of diabetes, gestational age at V1 and maternal BMI at V1; green line: ROC curve for GDM diagnosis based on all clinical variables plus plasma IGFBP1 levels (measured at a median of 9 weeks of gestation). GDM diagnosed by IADPSG criteria. Shaded areas: 95% CI for each curve (2,000 stratified bootstrap). Comparing AUC values with and without plasma IGFBP1 (Box–Cox transformation) using a two-sided DeLong’s test gave estimates (difference between 2 AUC) = −0.060, 95% CI = −0.104 to −0.015, z = −2.641, P = 0.008.
Predictive association between early pregnancy IGFBP1 levels and GDM incidence were replicated in a nested case–control study in the MOMS cohort ( n = 55 GDM cases, diagnosed based on Carpenter–Coustan criteria at a median of 29 weeks gestation; matched 1:2 with noncases): the OR was 0.40 (95% CI, 0.24–0.67; P < 0.001, adjusted for maternal age and BMI) per s.d. increase in plasma IGFBP1 (measured at a median of 17 weeks gestation). In the SPRING cohort, all GDM cases were combined ( n = 44 total, diagnosed either in early pregnancy or at 24–32 weeks gestation based on IADPSG criteria) and predictive analyses showed an OR of 0.75 (95% CI, 0.46–1.25; P = 0.28; adjusted for maternal age, BMI and gestational age at blood samples) for each SD increase in plasma IGFBP1 measured in the first trimester (median, 13 weeks gestation).
Circulating IGFBP1 in pregnancy by GDM physiologic subtype
Given the strong association between plasma IGFBP1 and insulin sensitivity in pregnancy, the longitudinal changes in plasma IGFBP1 across pregnancy in different physiologic subtypes of GDM (as defined previously 10 ) and in participants with normal glucose tolerance (NGT) were investigated in Gen3G (Fig. 3 ). All GDM subtypes had lower mean plasma IGFBP1 levels in early pregnancy compared with the NGT group. However, the insulin-resistant GDM group had a blunted increase in IGFBP1 levels between the first and second trimester; in contrast, in those with insulin-deficient GDM, IGFBP1 levels reached similar levels to those in the NGT group during the second trimester (Fig. 3 ). The group who had GDM with both insulin resistance and insulin deficiency (mixed defect GDM) showed an IGFBP1 trajectory that was intermediate between the other GDM subtypes.
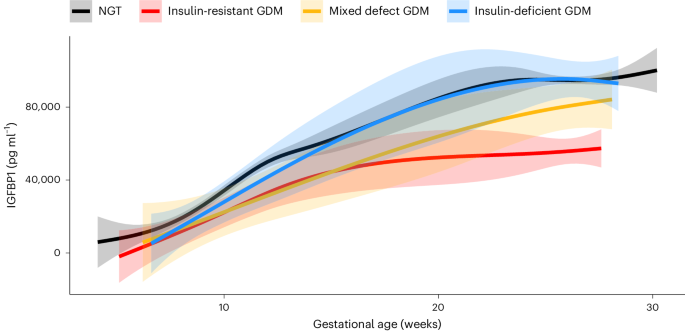
Sample size in each group: NGT, n = 767; insulin-resistant GDM, n = 34; insulin-deficient GDM, n = 19; and mixed defect GDM, n = 12. Lines represent the levels of circulating IGFBP1 (not transformed) from the first trimester visit to the late second trimester visit at exact gestational age of the measure, smoothed by a generalized additive model with parameter estimation via restricted maximum likelihood using ‘stat_smooth’ function from the ggplot2 package. Shaded areas represent 95% CIs.
In Gen3G, low IGFBP1 levels in first trimester were associated with subsequent diagnosis of both insulin-resistant GDM and insulin-deficient GDM with ORs ~0.4 (in fully adjusted models, including maternal BMI) similar to prediction models where the outcome was all GDM (Model 3; Table 3 ). However, IGFBP1 levels in the second trimester were associated only with insulin-resistant GDM (OR = 0.28 (0.16–0.47) per s.d. increase in IGFBP1 levels; P < 0.001); there was no statistically significant association between second trimester IGFBP1 plasma levels and insulin-deficient GDM (Table 3 ).
In this study, using genome-wide RNA-seq of placental tissue, we identify IGFBP1 as a key placental transcript associated with insulin sensitivity in human pregnancy. Our findings implicate IGFBP1 deficiency in GDM pathophysiology. We show that circulating IGFBP1 levels rise during pregnancy and are much higher in pregnancy than in the nonpregnant state, supporting the contribution of placental and/or decidual IGFBP1 to elevated circulating IGFBP1 in pregnancy. In three independent pregnancy cohorts, we demonstrate a strong and consistent correlation between higher circulating IGFBP1 and greater insulin sensitivity (lesser insulin resistance), uncovering a potential compensatory mechanism in euglycemic pregnancy. Moreover, we show that low plasma IGFBP1 levels in the first trimester of pregnancy predict the later diagnosis of GDM, independent of maternal clinical risk factors (including BMI). Finally, we note that the normal pregnancy rise in IGFBP1 levels is attenuated in insulin-resistant GDM, suggesting that a defect in placental IGFBP1 release may contribute specifically to this GDM physiologic subtype.
In placental tissues, IGFBP1 expression has been detected previously in decidual cells and in fetal placental macrophages or Hofbauer cells 24 ; however, there is limited knowledge of IGFBP1 regulation and actions in pregnancy. Given that our samples were collected from the maternal-facing side, it is possible that IGFBP1 expression and release from decidual cells contributed to IGFBP1 circulating levels. In vitro experiments using decidualized human endometrial stromal cells have shown IGFBP1 regulation by cAMP, progesterone and relaxin 25 , 26 —the latter two being critical hormones for the establishment and maintenance of pregnancy 27 . Outside of pregnancy, IGFBP1 is expressed almost exclusively by the liver 16 and its production is regulated by insulin, which inhibits its gene transcription in hepatocytes 28 . This is consistent with low IGFBP1 levels reflecting insulin resistance and hepatic steatosis in nonpregnant adults and children 18 , 19 , 29 . Our observations that plasma IGFBP1 levels decline after a plasma insulin rise in response to an oral glucose load introduce the possibility that insulin may downregulate the production and/or release of IGFBP1 from the placenta/decidua, similar to the downregulation observed in hepatocytes 28 . It is also possible that other insulin-sensitive endocrine factors, such as adiponectin, regulate IGFBP1 expression in placental cells 30 .
Functional studies suggest that IGFBP1 binds IGF-1 and IGF-2 with equal affinity and can either inhibit or enhance IGF actions, depending on the context 23 . In postnatal life, IGF-1 is the main active growth factor and is essential for normal growth during childhood and adolescence, whereas, during fetal development, both IGF-1 and IGF-2 are key regulators of fetal growth 23 , 31 . Outside of pregnancy, IGF-1 enhances insulin sensitivity by suppressing hepatic glucose production 32 , 33 and promoting glucose uptake in peripheral tissues 34 , 35 . IGF2 is a highly expressed placental imprinted gene that is a key regulator of fetal growth in mammals 36 . In a recent study, pregnant mice with an IGF2 deletion specific to placental endocrine cells did not develop the normal insulin resistance of pregnancy and gave birth to fetuses that were growth-restricted and hypoglycemic 37 . In general, IGFs have higher affinity for IGFBPs than for cellular IGF-receptors and, thus, IGFBPs, sometimes in combination with acid-labile subunits, often act as inhibitors of IGF biological activity 25 . PAPPA and PAPPA2 are two key proteinases released by the placenta that allow the cleavage of IGFBPs from IGFs; however, these proteinases do not cleave IGFBP1 (refs. 38 , 39 ). IGFBPs may also function as a circulating pool of IGFs by prolonging their half-lives and creating IGF reservoirs 17 , 23 . In addition, IGFBP1 has putative IGF-independent effects, and may activate PI3K/AKT signaling pathways involved in postreceptor insulin signaling directly 40 . In line with this, in vivo injection of an active IGFBP1 peptide improved insulin sensitivity in a diet-induced obesity mouse model 41 . These diverse mechanisms of action might explain some of the inconsistencies from previous animal studies attempting to establish the effects of IGFBP1 on glucose regulation 42 , 43 , 44 . Future studies of gestational glycemic pathophysiology should investigate the interrelations of IGFs with the different IGFBPs and their regulation from acid-labile subunits and PAPPAs in the context of pregnancy.
None of these previous studies provide insights into the specific role that IGFBP1 may have in pregnancy, when there are high circulating levels of IGFs, which are suspected to influence glucose metabolism 23 , 37 . We speculate that placental/decidual release of IGFBP1 may regulate insulin sensitivity in pregnancy—via direct and/or indirect effects—contributing physiologically to homeostatic mechanisms to balance maternal and fetal nutrient needs. An alternative explanation is that low levels of IGFBP1 in GDM are a consequence of hyperinsulinemia with another upstream cause, but this would not be consistent with the rise of circulating IGFBP1 throughout pregnancy (which is characterized by progressive hyperinsulinemia). In the context of GDM pathophysiology, based on our findings, in individuals with insulin-resistant GDM, we speculate that the placenta may be unable to produce increasing amounts of IGFBP1 as pregnancy progresses; this deficiency in circulating IGFBP1 could contribute to excessive insulin resistance, and thus to maternal hyperglycemia detected in the late second trimester in this GDM subtype. In individuals with insulin-deficient GDM, IGFBP1 amounts were low in the first trimester but amounts during the second semester were on par with those without GDM, suggesting that other pathophysiologic factors contribute to hyperglycemia in this GDM subtype. Given the differences in IGFBP1 in different GDM subtypes, and increasing recognition in the field that GDM is a heterogeneous condition 45 , our finding of persistently lower IGFBP1 levels in the second trimester of pregnancies affected by insulin-resistant GDM may have implications for GDM precision medicine 46 , 47 . Our findings suggest that, in cases of insulin-resistant GDM, the placenta does not increase IGFBP1 production sufficiently; if this association is demonstrated to be causal, this opens the door to a new therapeutic target for this GDM subtype. Beyond GDM, the association between lower circulating IGFBP1 levels and higher birthweight is in line with similar observations in an earlier report 48 and suggests a potential explanation for the greater risk of LGA birthweight that we observed previously in instances of insulin-resistant GDM 10 .
Accurately predicting GDM diagnosis in later pregnancy based on early pregnancy markers could allow development and implementation of interventions—such as counseling on diet and exercise—to prevent GDM and its complications. However, most predictive models that rely on established clinical risk factors perform poorly 49 , 50 and, thus, there has been a search for reliable and replicable biomarkers. We found that low levels of circulating IGFBP1 in early pregnancy predict later diagnosis of GDM in a large population-based cohort (Gen3G), with external replication and consistent effect sizes in a separate cohort (MOMS). The effect size was more modest and not statistically significant in a cohort study of participants who all had GDM risk factors (SPRING); these inclusion criteria may have diminished the predictive ability of circulating IGFBP1 in this population. Previous studies have been inconsistent with regard to circulating IGFBP1 as a predictive biomarker for GDM, with only one study reporting on IGFPB1 levels measured before 20 weeks of gestation 51 . Our ROC analyses showed that circulating IGFBP1 levels in early pregnancy have a predictive ability beyond that of established GDM risk factors (including maternal BMI and family history of diabetes); however, the moderate ROC AUC value in a model that included IGFBP1 levels along with these clinical factors suggests that additional biomarkers would be necessary for clinical utility. Future studies could also investigate whether urinary levels of IGFBP1 in pregnancy can predict GDM or characterize subtypes, which would be convenient for patients and clinicians. We do not know whether women who developed GDM in our study had low IGFBP1 levels before pregnancy, thus pre-pregnancy assessment of IGFBP1 should be considered in future studies of pre-conception interventions aiming to optimize metabolic outcomes in pregnancy.
Our investigation has several strengths. We included a large number of placental samples in our expression profiling, used transcriptome-wide RNA-seq and leveraged an agnostic approach to implicate genes and their products in insulin sensitivity during pregnancy. Furthermore, we examined not only placental expression of IGFBP1 , but also circulating IGFBP1 levels in three pregnancy cohorts. Our analyses included measurement of circulating IGFBP1 levels over a longitudinal timeframe that spanned both pregnancy and postpartum. In addition, we used an OGTT-based measure of insulin sensitivity that has been validated against euglycemic clamps in pregnancy. Our study also had some limitations. Although we had a large overall sample size, the number of GDM cases was somewhat modest, and the sample size for each GDM physiologic subtype was even more limited. We conducted RNA-seq on bulk samples, including placental and decidual cells, thus we cannot confirm the exact cellular source of IGFBP1. Although we were able to tie placental/decidual RNA expression and circulating IGFBP1 levels to detailed physiologic phenotyping, our study was observational and thus cannot establish mechanisms or causality for the associations we observed.
In conclusions, starting from agnostic and unbiased placental gene expression profiling, we implicated IGFBP1 in insulin sensitivity during pregnancy. IGFBP1 was expressed highly in our placental samples and maternal IGFBP1 levels are elevated markedly during gestation, increasing across pregnancy and dropping substantially postpartum. Both placental and circulating IGFBP1 levels are correlated strongly and consistently with maternal insulin sensitivity. A deficiency of circulating IGFBP1 in early pregnancy predicts the diagnosis of GDM in the late second trimester, independent of clinical GDM risk factors in two different pregnancy cohorts. We demonstrated distinct IGFBP1 trajectories in different physiologic subtypes of GDM, with insulin-resistant GDM lacking the expected increase in circulating IGFBP1 across gestation. Future studies should address whether IGFBP1 has direct or indirect effects on tissues that regulate maternal insulin sensitivity during pregnancy. If IGFBP1 is causally implicated in gestational glycemic regulation, new therapeutic approaches based on IGFBP1 replacement as an insulin sensitizer could be envisioned and tested for precision prevention or treatment of GDM.
All three human cohorts included in this study recruited pregnant individuals who are all female (sex as a biological determinant) given that only biological female can experience pregnancy. We acknowledge that not all pregnant individuals self-identify as women (gender). Our study aimed at understanding biology of glucose regulation in pregnancy, thus our analyses apply to female individuals. All participants provided informed consent.
Gen3G cohort
Gen3G is a prospective population-based cohort that recruited pregnant women from 1 January 2010 to 30 June 2013 at the Centre Hospitalier Universitaire de Sherbrooke (CHUS), located in the province of Quebec (Canada). Participants were demographically representative of the greater population of the region 21 . Each study participant provided informed written consent, and the study protocols were reviewed by the ethical committees from CHUS, and from the Harvard Pilgrim Health Care Institute.
We recruited 1,024 pregnant women without preexisting diabetes in the first trimester (diabetes diagnosis from self-report or biochemical screening with HbA1c ≥6.5%). Exclusion criteria for enrollment in the cohort were nonsingleton pregnancies or regular use of medications that influence glucose regulation. We collected measurements and blood samples from mothers at a first trimester visit (V1) conducted between 5 and 16 weeks of gestation (median 9 weeks), and in the late second trimester (V2) at 24 to 30 weeks of gestation (median 26 weeks; the time of universal GDM screening). We collected placental samples in addition to data on mothers and offspring at delivery.

Variables collection and measurements
At V1, we collected demographic data and previous medical and obstetric history; we performed standardized anthropometric measurements. Trained research staff measured weight with a calibrated scale and height with a standardized stadiometer. We calculated first trimester BMI as weight divided by squared height (kg m −2 ). At V1, we also collected additional blood samples that were drawn during the 50 g glucose challenge test (GCT, performed in 95% of participants). For the current study, we excluded participants who had a first trimester random glucose or 1 h-glucose post-GCT >10.3 mmol l −1 (overt hyperglycemia per national guidelines at the time) as we were interested in GDM incidence (ascertained with universal testing at 24–30 weeks).
At V2, we performed similar anthropometric measurements and questionnaires as at V1. V2 occurred at the time of the fasting 75g-OGTT, which was standard clinical practice for screening and diagnosis of GDM at CHUS. We collected additional blood samples at the fasting, 1 h and 2 h time points of the 75g-OGTT to measure insulin at each time point in addition to glucose. We measured glucose levels via the hexokinase method (Roche Diagnostics; CHUS biochemistry laboratory) as soon as samples were collected. We measured insulin levels via multiplexed particle-based flow cytometric assays (Human Milliplex MAP kits; EMD Millipore) from the previously frozen plasma samples (stored at −80°C until measurement). We estimated insulin sensitivity using the Matsuda Index 52 (using glucose and insulin values during the OGTT), as previously validated against euglycemic clamps performed in pregnancy 22 .
At delivery, we collected newborn age and sex at birth using medical records, in addition to details from the end of pregnancy and delivery complications. Trained study staff collected placentas within 30 min of delivery using a standardized protocol. In brief, a 1 cm 3 placental tissue sample was collected from the maternal-facing side, including decidual tissue (within a 5 cm radius of the corresponding location of cord insertion on the other side). Each collected sample was immediately put in RNA-Later for at least 24 h at 4 °C before storage at −80 °C until RNA extraction.
RNA extraction, sequencing and QC
We extracted total RNA (average, 19.7 ± 7.1 µg) and checked the quality of each sample using an Agilent Bioanalyzer to determine the RNA integrity number (average RNA integrity number = 6.7 ± 0.8). We shipped samples (3 µg) with an RNA integrity number value ≥5 to the Broad Institute for sequencing. In a second round of sample QC at the Broad Institute (Caliper Life Sciences LabChip GX system), the RNA quality score for each sample ranged from 3.3 to 7.8 (average RNA quality score = 5.9). We submitted all samples with an RNA quality score value of 4 or higher for RNA sequencing ( n = 466). We completed library preparation with 250 ng of each sample, using an automated variant of the Illumina TruSeq Stranded mRNA Sample Preparation Kit (Illumina, catalog number RS-122-2103). We performed Flowcell cluster amplification and sequencing according to the manufacturer’s protocols using the Illumina HiSeq 4000, to generate 101-bp paired-end reads, average of 113 million total reads (range 33 million to 378 million) per sample.
In line with best practice and the GTEx v.8 pipeline 53 , we applied STAR v.2.5.3a 54 to align FASTQ/FASTA files to the human GRCh38 reference genome, using the parameters specified at https://github.com/broadinstitute/gtex-pipeline . Duplicate reads were marked using Picard MarkDuplicates, and expression was quantified with RNASeQC v.2.3.6 using the GENCODE v.26 annotation 55 .
Following quantification, we applied additional QC steps. Of the 466 samples sequenced, we excluded those with >1% of outlier genes (>3 times the IQR above Q3 or >3 IQR below Q1), leaving 459 samples for our final analytical dataset. Among these, we had complete data on the phenotype of interest (Matsuda index) and covariates for 434 samples. Before differential gene expression analysis, we removed genes with low abundance, keeping only those genes with at least a count of six reads and a transcript per million values >0.5 in a minimum of 20% of samples, as well as average mappability ≥0.8. After QC, 15,202 genes remained. Before differential expression analysis, we performed between-sample normalization using the R statistical software package edgeR 56 , then normalized and transformed gene counts to log 2 counts per million reads using Voom from the Limma R package 57 . The Gen3G placental RNA-seq data are available on dbGAP ( https://www.ncbi.nlm.nih.gov/projects/gap/cgi-bin/study.cgi?study_id=phs003151.v1.p1 ).
Study of pregnancy regulation of insulin and glucose
SPRING is a longitudinal cohort study of pregnant participants with risk factors for diabetes that was conducted in 2015–2021. Participants were eligible if they were at <15 weeks gestation and had a history of GDM, family history of diabetes or GDM, or if they had BMI ≥ 25 kg m −2 and had one additional risk factor according to American Diabetes Association guidelines 58 . Participants gave informed consent and underwent a fasting 75g-OGTT at <15 weeks gestation, 24–28 weeks gestation and 6–12 weeks postpartum. The latter two study visit windows were widened to 24–32 weeks gestation and 6–24 weeks postpartum to promote participant retention (including during the COVID-19 pandemic). We measured glucose and insulin levels as previous described 15 . The Matsuda index was calculated from the glucose and insulin levels measured during the OGTT 15 . GDM was diagnosed according to IADPSG criteria applied to the OGTT at the pregnancy study visits. Most participants that met IADPSG criteria at the first visit were not retested at the second visit. Blood samples from each study visit were collected in EDTA plasma tubes, processed and frozen at −80 °C for future analysis. The study was approved by the Mass General Brigham Institutional Review Board.
MGH obstetrical maternal study
MOMS was conducted from 1998 to 2006 (ref. 59 ). Participants were eligible if they were receiving prenatal care at MGH. Participants provided written informed consent and were enrolled at their first prenatal visit where they donated an extra blood sample from a clinical blood draw. A subset of participants in 2001–2006 volunteered to return to donate fasting blood and urine samples at 16–20 weeks gestation. Glucose and insulin levels were measured as previously described 60 . Fasting plasma samples were frozen at −80 °C and stored for future analyses. At 24–28 weeks gestation, participants without preexisting diabetes underwent universal screening for GDM with a nonfasting 50 g GCT. If the venous blood glucose 1 h after the GCT was ≥140 mg dl −1 , patients were referred for a diagnostic 3-h 100g-OGTT. For this analysis, we included individuals whose OGTT results met Carpenter–Coustan criteria for GDM (≥2 abnormal values). Of these participants with GDM, 55 had remaining fasting samples available for analysis. We matched control participants with normal GCT results (two for each GDM case) on year of sample collection and gestational age at sample collection. We preferentially selected control samples on which fasting glucose and insulin had previously been measured on the sample collected at 16–20 weeks gestation. We calculated HOMA-2S from fasting glucose and insulin values to estimate insulin sensitivity 61 ( https://www.rdm.ox.ac.uk/about/our-clinical-facilities-and-units/DTU/software/homa ).
Institutional review board approval was obtained for participants from each of the cohorts (Gen3G, SPRING, MOMS) following the principles outlined in the Declaration of Helsinki. All enrolled participants provided written informed consent before study procedures.
Bioassays for circulating IGFBP1
We measured circulating IGFBP1 in plasma samples from all three cohorts in the same laboratory using a commercially available enzyme-linked immunosorbent assay that measures free IGFBP1 (R&D systems, catalog number DGB100). The precision for the assays were: intra-assay coefficients of variation of 5.6% and inter-assay coefficients of variation of 9.5%. We measured IGFBP1 levels in a blinded fashion, and we followed protocol for measurement per manufacturer’s instructions.
Statistical analyses
For characteristics of participants in all three cohorts, we reported normally distributed continuous variables as mean ± s.d., non-normally distributed continuous variables as median and IQR, and categorical variables as percentages. We used a log 2 transformation for Matsuda index (to approach a normal distribution) in the differential placental RNA expression analyses.
Placental differential expression analyses using RNA-seq data in Gen3G
We adjusted models for maternal age, gravidity, maternal BMI at the first trimester visit, sex of offspring and gestational age at delivery, in addition to computed SVs to account for unmeasured sources of variability, including batch effects and cell types. We used the EstDimRMT function from the R package isva 62 to estimate the number of SVs to include given the residuals from the regression of Matsuda and biological covariates from the normalized counts, which resulted in 37 SVs computed by the R package SmartSVA 63 recommended for our processed RNA-seq dataset. We used Limma 64 to identify differentially expressed genes with log 2 Matsuda as a continuous independent variable. We reported genes that had differential expression in relation to Matsuda with P < 1.0 × 10 −3 .
Circulating IGFBP1 correlation analyses
We carefully assessed plasma IGFBP1 distribution and, after considering different potential transformations, we used a Box–Cox transformation for plasma IGFBP1 levels in Gen3G (from MASS package 65 in R) since it was the best way to approximate a normal distribution. We conducted analyses in SPRING and MOMS cohorts using plasma IGFBP1 levels without transformation, given distributions that were relatively normal. We used Pearson correlations between circulating IGFBP1 levels and Matsuda index (log transformed) in all three cohorts; we used partial correlations to assess the associations while taking into account maternal age, gestational age at blood draw and maternal BMI. In Gen3G, we also used Pearson correlations to assess associations between plasma IGFBP1 (Box–Cox transformed) and maternal metabolic markers, as well as newborn anthropometry (transformed if needed).
Circulating IGFBP1 and risk of GDM analyses
We conducted logistic regression analyses with the levels of circulating IGFBP1 as the independent variable and GDM as the dependent variable in Gen3G and SPRING; in MOMS, due to the matched case–control design of GDM cases to controls, we used conditional logistic regression. In Gen3G and SPRING, we used international criteria (IADPSG) 66 to ascertain GDM, whereas in MOMS we used the Carpenter–Coustan criteria 67 . In Gen3G, we additionally subclassified GDM by the insulin physiology defect driving hyperglycemia (insulin-resistant GDM, insulin-deficient GDM or mixed defect GDM, as previously described 10 ). We first built unadjusted logistic regression analyses (Model 1). We adjusted for maternal characteristics (maternal age, gravidity, gestational age at plasma samples) in Model 2 and additionally adjusted for maternal BMI in Model 3. We calculated profiled log-likelihood CIs along with likelihood ratio test P values (using MASS 65 and glmglrt ( https://CRAN.R-project.org/package=glmglrt ) packages 47 in R). In SPRING and MOMS cohorts, we employed similar modeling strategies using maximum likelihood dichotomous logistic models.
We conducted GDM predictive analyses using ROC curves in Gen3G to compare the predictive ability of first trimester (V1) plasma IGFBP1 levels in addition to commonly measured GDM clinical risk factors (maternal age, gravidity, family history of diabetes, gestational age at V1 and maternal BMI at V1). We compared the ROC AUC values using all the clinical factors with and without first trimester (V1) plasma IGFBP1 levels (after Box–Cox transformation). We compared the ROC AUC values from nested models using DeLong’s test using the roc.test function from the pROC package in R 68 . We considered differences between AUC values to be statistically significant if P < 0.05. In Gen3G, we performed analyses using R v.4.3.0 ( https://www.R-project.org ), STATA and SPSS v.28 only for partial correlations. In SPRING and MOMS, we performed analyses using Stata/IC v.16.1. Original code developed for placenta RNA-seq differential expression analysis in Gen3G available at https://github.com/labjacquespe/diff-exp .
Reporting summary
Further information on research design is available in the Nature Portfolio Reporting Summary linked to this article.
Data availability
The Gen3G placental RNA-seq data and pregnancy phenotypes are available on dbGAP ( https://www.ncbi.nlm.nih.gov/projects/gap/cgi-bin/study.cgi?study_id=phs003151.v1.p1 ).
Code availability
Original code developed for placenta RNA-seq differential expression analysis in Gen3G is available at https://github.com/labjacquespe/diff-exp .
Magliano, D., Boyko, E. J. & IDF Diabetes Atlas 10th Edition Scientific Committee. in IDF Diabetes Atlas (eds Boyko, E. J. et al.) Ch. 3, Global picture (International Diabetes Federation, 2021); https://diabetesatlas.org/atlas/tenth-edition/
ElSayed, N. A. et al. 15. Management of diabetes in pregnancy: standards of care in diabetes—2023. Diabetes Care 46 , S254–S266 (2023).
Article CAS PubMed Google Scholar
Catalano, P. M., Kirwan, J. P., Haugel-de Mouzon, S. & King, J. Gestational diabetes and insulin resistance: role in short- and long-term implications for mother and fetus. J. Nutr. 133 , 1674S–1683S (2003).
Barbour, L. A. et al. Cellular mechanisms for insulin resistance in normal pregnancy and gestational diabetes. Diabetes Care 30 , S112–S119 (2007).
Sun, Y. et al. Increasing insulin resistance predicts adverse pregnancy outcomes in women with gestational diabetes mellitus. J. Diabetes 12 , 438–446 (2020).
Li, J. et al. Roles of insulin resistance and beta cell dysfunction in macrosomia among Chinese women with gestational diabetes mellitus. Prim. Care Diabetes 12 , 565–573 (2018).
Article PubMed PubMed Central Google Scholar
Wang, N. et al. Contribution of gestational diabetes mellitus heterogeneity and prepregnancy body mass index to large‐for‐gestational‐age infants—a retrospective case–control study. J. Diabetes 13 , 307–317 (2021).
Madsen, L. R. et al. Do variations in insulin sensitivity and insulin secretion in pregnancy predict differences in obstetric and neonatal outcomes? Diabetologia 64 , 304–312 (2021).
Immanuel, J. et al. Metabolic phenotypes of early gestational diabetes mellitus and their association with adverse pregnancy outcomes. Diabet. Med. 38 , e14413 (2021).
Powe, C. E. et al. Heterogeneous contribution of insulin sensitivity and secretion defects to gestational diabetes mellitus. Diabetes Care 39 , 1052–1055 (2016).
Article CAS PubMed PubMed Central Google Scholar
Beck, P. & Daughaday, W. H. Human placental lactogen: studies of its acute metabolic effects and disposition in normal man. J. Clin. Invest. 46 , 103–110 (1967).
Kirwan, J. P. et al. TNF-alpha is a predictor of insulin resistance in human pregnancy. Diabetes 51 , 2207–2213 (2002).
Powe, C. E., Huston Presley, L. P., Locascio, J. J. & Catalano, P. M. Augmented insulin secretory response in early pregnancy. Diabetologia 62 , 1445–1452 (2019).
García-Patterson, A. et al. Insulin requirements throughout pregnancy in women with type 1 diabetes mellitus: three changes of direction. Diabetologia 53 , 446–451 (2010).
Article PubMed Google Scholar
Thaweethai, T., Soetan, Z., James, K., Florez, J. C. & Powe, C. E. Distinct insulin physiology trajectories in euglycemic pregnancy and gestational diabetes mellitus. Diabetes Care 46 , 2137–2146 (2023).
Uhlén, M. et al. Proteomics. Tissue-based map of the human proteome. Science 347 , 1260419 (2015).
Duan, C. & Xu, Q. Roles of insulin-like growth factor (IGF) binding proteins in regulating IGF actions. Gen. Comp. Endocrinol. 142 , 44–52 (2005).
Petersson, U., Ostgren, C. J., Brudin, L., Brismar, K. & Nilsson, P. M. Low levels of insulin-like growth-factor-binding protein-1 (IGFBP-1) are prospectively associated with the incidence of type 2 diabetes and impaired glucose tolerance (IGT): the Söderåkra Cardiovascular Risk Factor Study. Diabetes Metab. 35 , 198–205 (2009).
Hagström, H., Stål, P., Hultcrantz, R., Brismar, K. & Ansurudeen, I. IGFBP-1 and IGF-I as markers for advanced fibrosis in NAFLD—a pilot study. Scand. J. Gastroenterol. 52 , 1427–1434 (2017).
Rajpathak, S. N. et al. Insulin-like growth factor axis and risk of type 2 diabetes in women. Diabetes 61 , 2248–2254 (2012).
Guillemette, L. et al. Genetics of glucose regulation in gestation and growth (Gen3G): a prospective prebirth cohort of mother-child pairs in Sherbrooke, Canada. BMJ Open 6 , e010031 (2016).
Kirwan, J. P., Huston-Presley, L., Kalhan, S. C. & Catalano, P. M. Clinically useful estimates of insulin sensitivity during pregnancy: validation studies in women with normal glucose tolerance and gestational diabetes mellitus. Diabetes Care 24 , 1602–1607 (2001).
Martín-Estal, I. & Castorena-Torres, F. Gestational diabetes mellitus and energy-dense diet: what is the role of the insulin/IGF axis? Front. Endocrinol. 13 , 916042 (2022).
Article Google Scholar
Suryawanshi, H. et al. A single-cell survey of the human first-trimester placenta and decidua. Sci. Adv. 4 , eaau4788 (2018).
Bae, J.-H., Song, D.-K. & Im, S.-S. Regulation of IGFBP-1 in metabolic diseases. J. Lifestyle Med. 3 , 73–79 (2013).
PubMed PubMed Central Google Scholar
Gao, J., Mazella, J., Suwanichkul, A., Powell, D. R. & Tseng, L. Activation of the insulin-like growth factor binding protein-1 promoter by progesterone receptor in decidualized human endometrial stromal cells. Mol. Cell. Endocrinol. 153 , 11–17 (1999).
Goldsmith, L. T. & Weiss, G. Relaxin in human pregnancy. Ann. N. Y. Acad. Sci. 1160 , 130–135 (2009).
Powell, D. R. et al. Insulin inhibits transcription of the human gene for insulin-like growth factor-binding protein-1. J. Biol. Chem. 266 , 18868–18876 (1991).
Lewitt, M. S., Dent, M. S. & Hall, K. The insulin-like growth factor system in obesity, insulin resistance and type 2 diabetes mellitus. J. Clin. Med. 3 , 1561–1574 (2014).
Qiao, L. et al. Knockout maternal adiponectin increases fetal growth in mice: potential role for trophoblast IGFBP-1. Diabetologia 59 , 2417–2425 (2016).
Kaur, H., Muhlhausler, B. S., Roberts, C. T. & Gatford, K. L. The growth hormone-insulin like growth factor axis in pregnancy. J. Endocrinol. 251 , R23–R29 (2021).
Article CAS Google Scholar
Laager, R., Ninnis, R. & Keller, U. Comparison of the effects of recombinant human insulin-like growth factor-I and insulin on glucose and leucine kinetics in humans. J. Clin. Invest. 92 , 1903–1909 (1993).
Jacob, R., Barrett, E., Plewe, G., Fagin, K. D. & Sherwin, R. S. Acute effects of insulin-like growth factor I on glucose and amino acid metabolism in the awake fasted rat. Comparison with insulin. J. Clin. Invest. 83 , 1717–1723 (1989).
Clemmons, D. R., Sleevi, M., Allan, G. & Sommer, A. Effects of combined recombinant insulin-like growth factor (IGF)-I and IGF binding protein-3 in type 2 diabetic patients on glycemic control and distribution of IGF-I and IGF-II among serum binding protein complexes. J. Clin. Endocrinol. Metab. 92 , 2652–2658 (2007).
Scavo, L. M., Karas, M., Murray, M. & Leroith, D. Insulin-like growth factor-I stimulates both cell growth and lipogenesis during differentiation of human mesenchymal stem cells into adipocytes. J. Clin. Endocrinol. Metab. 89 , 3543–3553 (2004).
Sferruzzi-Perri, A. N. Regulating needs: exploring the role of insulin-like growth factor-2 signalling in materno-fetal resource allocation. Placenta 64 , S16–S22 (2018).
Lopez-Tello, J. et al. Fetal manipulation of maternal metabolism is a critical function of the imprinted Igf2 gene. Cell Metab. 35 , 1195–1208.e6 (2023).
Oxvig, C. & Conover, C. A. The stanniocalcin-PAPP-A-IGFBP-IGF axis. J. Clin. Endocrinol. Metab. 108 , 1624–1633 (2023).
Oxvig, C. The role of PAPP-A in the IGF system: location, location, location. J. Cell Commun. Signal. 9 , 177–187 (2015).
Wheatcroft, S. B. & Kearney, M. T. IGF-dependent and IGF-independent actions of IGF-binding protein-1 and -2: implications for metabolic homeostasis. Trends Endocrinol. Metab. 20 , 153–162 (2009).
Haywood, N. J. et al. Insulin-like growth factor binding protein 1 could improve glucose regulation and insulin sensitivity through its RGD domain. Diabetes 66 , 287–299 (2017).
Crossey, P. A., Jones, J. S. & Miell, J. P. Dysregulation of the insulin/IGF binding protein-1 axis in transgenic mice is associated with hyperinsulinemia and glucose intolerance. Diabetes 49 , 457–465 (2000).
Rajwani, A. et al. Increasing circulating IGFBP1 levels improves insulin sensitivity, promotes nitric oxide production, lowers blood pressure, and protects against atherosclerosis. Diabetes 61 , 915–924 (2012).
Haywood, N. J. et al. IGFBP-1 in cardiometabolic pathophysiology-insights from loss-of-function and gain-of-function studies in male mice. J. Endocr. Soc. 4 , bvz006 (2020).
Powe, C. E., Hivert, M.-F. & Udler, M. S. Defining heterogeneity among women with gestational diabetes mellitus. Diabetes 69 , 2064–2074 (2020).
Tobias, D. K. et al. Second international consensus report on gaps and opportunities for the clinical translation of precision diabetes medicine. Nat. Med. 29 , 2438–2457 (2023).
Francis, E. C. et al. Refining the diagnosis of gestational diabetes mellitus: a systematic review and meta-analysis. Commun. Med. (Lond). 3 , 185 (2023).
Alekseenkova, E. N. et al. Maternal insulin-like growth factors and insulin-like growth factor-binding proteins for macrosomia prediction in diabetic and nondiabetic pregnancy: a prospective study. Int. J. Gynaecol. Obstet. 162 , 605–613 (2023).
Thong, E. P. et al. Optimising cardiometabolic risk factors in pregnancy: a review of risk prediction models targeting gestational diabetes and hypertensive disorders. J. Cardiovasc. Dev. Dis. 9 , 55 (2022).
CAS PubMed PubMed Central Google Scholar
Kotzaeridi, G. et al. Performance of early risk assessment tools to predict the later development of gestational diabetes. Eur. J. Clin. Invest. 51 , e13630 (2021).
Wang, X.-R. et al. Insulin-like growth factor axis biomarkers and gestational diabetes mellitus: a systematic review and meta-analysis. Front. Endocrinol. 10 , 444 (2019).
Matsuda, M. & DeFronzo, R. A. Insulin sensitivity indices obtained from oral glucose tolerance testing: comparison with the euglycemic insulin clamp. Diabetes Care 22 , 1462–1470 (1999).
The GTEx Consortium et al. The GTEx Consortium atlas of genetic regulatory effects across human tissues. Science 369 , 1318–1330 (2020).
Article PubMed Central Google Scholar
Dobin, A. et al. STAR: ultrafast universal RNA-seq aligner. Bioinformatics 29 , 15–21 (2013).
Graubert, A., Aguet, F., Ravi, A., Ardlie, K. G. & Getz, G. RNA-SeQC 2: efficient RNA-seq quality control and quantification for large cohorts. Bioinformatics 37 , 3048–3050 (2021).
Robinson, M. D., McCarthy, D. J. & Smyth, G. K. edgeR: a Bioconductor package for differential expression analysis of digital gene expression data. Bioinformatics 26 , 139–140 (2010).
Law, C. W., Chen, Y., Shi, W. & Smyth, G. K. voom: precision weights unlock linear model analysis tools for RNA-seq read counts. Genome Biol. 15 , R29 (2014).
ElSayed, N. A. et al. 10. Cardiovascular disease and risk management: standards of care in diabetes-2023. Diabetes Care 46 , S158–S190 (2023).
Wolf, M. et al. Obesity and preeclampsia: the potential role of inflammation. Obstet. Gynecol. 98 , 757–762 (2001).
CAS PubMed Google Scholar
Stuebe, A. M., McElrath, T. F., Thadhani, R. & Ecker, J. L. Second trimester insulin resistance, early pregnancy body mass index and gestational weight gain. Matern. Child Health J. 14 , 254–260 (2010).
Levy, J. C., Matthews, D. R. & Hermans, M. P. Correct homeostasis model assessment (HOMA) evaluation uses the computer program. Diabetes Care 21 , 2191–2192 (1998).
Teschendorff, A. E., Zhuang, J. & Widschwendter, M. Independent surrogate variable analysis to deconvolve confounding factors in large-scale microarray profiling studies. Bioinformatics 27 , 1496–1505 (2011).
Chen, J. et al. Fast and robust adjustment of cell mixtures in epigenome-wide association studies with SmartSVA. BMC Genomics 18 , 413 (2017).
Ritchie, M. E. et al. limma powers differential expression analyses for RNA-sequencing and microarray studies. Nucleic Acids Res. 43 , e47 (2015).
Venables, W. N. & Ripley, B. D. Modern Applied Statistics with S (Springer, New York, 2002).
International Association of Diabetes and Pregnancy Study Groups Consensus Panel. et al. International association of diabetes and pregnancy study groups recommendations on the diagnosis and classification of hyperglycemia in pregnancy. Diabetes Care 33 , 676–682 (2010).
ACOG Practice Bulletin No. 190: gestational diabetes mellitus. Obstet. Gynecol. 131 , e49–e64 (2018).
Robin, X. et al. pROC: an open-source package for R and S+ to analyze and compare ROC curves. BMC Bioinf. 12 , 77 (2011).
Download references
Acknowledgements
This work was supported by a grant from the National Institutes of Health (R01HD094150). Gen3G was initially supported by a Fonds de recherche du Québec—Santé operating grant (to M-.F.H., grant no. 20697), Canadian Institute of Health Research (CIHR) operating grants (to M.-F.H., grant no. MOP 115071, and to L.B., grant no. PJT-152989) and a Diabète Québec grant. J.C.F. is supported by National Heart, Lung, and Blood Institute grant no. K24 HL157960. L.B. and P-.E.J. are senior research scholars from the Fonds de recherche du Québec—Santé. M-.F.H. was a recipient of an American Diabetes Association Pathways To Stop Diabetes Accelerator Award (grant no. 1-15-ACE-26). The SPRING cohort was supported by the National Institute of Diabetes and Digestive and Kidney Diseases (K23DK113218), the Robert Wood Johnson Foundation’s Harold Amos Medical Faculty Development Program and the MGH Claflin Distinguished Scholar Award. SPRING data collection was also supported by grants UL1TR001102 and UL1TR000170 to the Harvard Clinical and Translational Science Center from the National Center for Advancing Translational Science. The work in the MOMS cohort was supported by the MGH Physician Scientist Development Award and the Claflin Distinguished Scholar Award.
Author information
Authors and affiliations.
Department of Population Medicine, Harvard Medical School, Harvard Pilgrim Health Care Institute, Boston, MA, USA
Marie-France Hivert & Sana Majid
Diabetes Unit, Endocrine Division, Department of Medicine, Massachusetts General Hospital, Boston, MA, USA
Marie-France Hivert, Jose C. Florez & Camille E. Powe
Centre de Recherche du Centre Hospitalier Universitaire de Sherbrooke (CRCHUS), Sherbrooke, Quebec, Canada
Marie-France Hivert, Catherine Allard, Luigi Bouchard & Pierre-Étienne Jacques
Département de Biologie, Université de Sherbrooke, Sherbrooke, Quebec, Canada
Frédérique White & Pierre-Étienne Jacques
Department of Obstetrics and Gynecology, Massachusetts General Hospital, Harvard Medical School, Boston, MA, USA
Kaitlyn James, Andrea G. Edlow & Camille E. Powe
Broad Institute of MIT and Harvard, Cambridge, MA, USA
François Aguet, Kristin G. Ardlie, Jose C. Florez & Camille E. Powe
Center for Genomic Medicine, Massachusetts General Hospital, Boston, MA, USA
Jose C. Florez
Department of Medicine, Harvard Medical School, Boston, MA, USA
Jose C. Florez & Camille E. Powe
Department of Biochemistry and Functional Genomics, Université de Sherbrooke, Sherbrooke, Quebec, Canada
Luigi Bouchard
Department of Medical Biology, CIUSSS of Saguenay-Lac-Saint-Jean, Saguenay, Quebec, Canada
Institut de Recherche sur le Cancer de l’Université de Sherbrooke (IRCUS), Sherbrooke, Quebec, Canada
Pierre-Étienne Jacques
Department of Medicine, Cedars-Sinai Medical Center, Los Angeles, CA, USA
S. Ananth Karumanchi
You can also search for this author in PubMed Google Scholar
Contributions
M-.F.H. wrote the paper, supervised analyses and obtained funding for the study. F.W., C.A., K.J. and S.M. performed analyses and prepared tables and figures. F.A. and K.G.A. supervised RNA-seq analyses, P-.E.J. supervised differential RNA expression analyses and S.A.K. supervised plasma IGFBP1 assays. C.E.P. obtained funding and directed the work in the SPRING and MOMS cohorts. F.A., J.C.F., A.G.E., L.B., P-.E.J., S.A.K. and C.E.P. provided critical input on interpretation of the findings. All authors reviewed and approved the final paper.
Corresponding author
Correspondence to Marie-France Hivert .
Ethics declarations
Competing interests.
C.E.P. is an Associate Editor of Diabetes Care, receives payments from Wolters Kluwer for UpToDate chapters on diabetes in pregnancy and has received payments for consulting and speaking from Mediflix. M.-F.H. is co-editor of textbook ‘Essentials of Clinical Nutrition in Healthcare’ published by McGraw Hill. F.A. has been an employee of Illumina since 8 November 2021. J.C.F. has received grant funding for an investigator-initiated proposal from Novo Nordisk, a one-time consulting honorarium from AstraZeneca and speaker fees from Merck and Novo Nordisk for scientific presentations over which he had full control of content. J.C.F.’s wife has received a one-time consulting honorarium from Novartis. A.G.E. serves as a consultant for Mirvie and receives research funding from Merck Pharmaceuticals outside of this work. None of these engagements are directly relevant to this work. The other authors declare no competing interests.
Peer review
Peer review information.
Nature Medicine thanks Amanda Sferuzzi-Perri and the other, anonymous, reviewer(s) for their contribution to the peer review of this work. Primary Handling Editor: Sonia Muliyil, in collaboration with the Nature Medicine team.
Additional information
Publisher’s note Springer Nature remains neutral with regard to jurisdictional claims in published maps and institutional affiliations.
Extended data
Extended data fig. 1 longitudinal changes in median plasma igfbp-1 levels across pregnancy and postpartum in normoglycemic spring participants (n = 65)..
IGFBP1 levels comparison using two-sided Wilcoxon signed-rank test, unadjusted for multiple comparisons. Exact P = 5.08 ×10 -15 for differences between V3 (median 9 weeks post-partum) and V1 (median = 13 weeks gestation) and P = 9.18 ×10 -19 for differences between V3 and V2 (median= 26 weeks gestation), denoted with *. Blue line is linking median value at each time point. Red bars represent interquartile range at each time point.
Extended Data Fig. 2 Longitudinal changes in median plasma levels of IGFBP-1, insulin, and glucose during 75g-OGTT conducted at median 26 weeks in 27 Gen3G participants.
Panel A: IGFBP1 levels comparison using two-sided Wilcoxon signed-rank test: V = 125, P = 0.13 for differences between 60 min and fasting (0 min); V = 324, P = 0.0007 (denoted with *) for differences between 120 min and fasting (0 min) without adjustment for multiple comparisons. Panel B: insulin levels over three time points of OGTT. Panel C: glucose levels over three time points of OGTT. Blue lines are linking median value between time points. Red bars represent interquartile range at each time point.
Supplementary information
Reporting summary, rights and permissions.
Open Access This article is licensed under a Creative Commons Attribution 4.0 International License, which permits use, sharing, adaptation, distribution and reproduction in any medium or format, as long as you give appropriate credit to the original author(s) and the source, provide a link to the Creative Commons licence, and indicate if changes were made. The images or other third party material in this article are included in the article’s Creative Commons licence, unless indicated otherwise in a credit line to the material. If material is not included in the article’s Creative Commons licence and your intended use is not permitted by statutory regulation or exceeds the permitted use, you will need to obtain permission directly from the copyright holder. To view a copy of this licence, visit http://creativecommons.org/licenses/by/4.0/ .
Reprints and permissions
About this article
Cite this article.
Hivert, MF., White, F., Allard, C. et al. Placental IGFBP1 levels during early pregnancy and the risk of insulin resistance and gestational diabetes. Nat Med (2024). https://doi.org/10.1038/s41591-024-02936-5
Download citation
Received : 18 October 2023
Accepted : 21 March 2024
Published : 16 April 2024
DOI : https://doi.org/10.1038/s41591-024-02936-5
Share this article
Anyone you share the following link with will be able to read this content:
Sorry, a shareable link is not currently available for this article.
Provided by the Springer Nature SharedIt content-sharing initiative
Quick links
- Explore articles by subject
- Guide to authors
- Editorial policies
Sign up for the Nature Briefing newsletter — what matters in science, free to your inbox daily.

Diane M. Omalley
Sharing Educational Goals
Our cheap essay service is a helping hand for those who want to reach academic success and have the perfect 4.0 GPA. Whatever kind of help you need, we will give it to you.
Essay Help Services – Sharing Educational Integrity
Hire an expert from our writing services to learn from and ace your next task. We are your one-stop-shop for academic success.
John N. Williams
Customer Reviews
Courtney Lees
Customer Reviews
Margurite J. Perez
Andersen, Jung & Co. is a San Francisco based, full-service real estate firm providing customized concierge-level services to its clients. We work to help our residential clients find their new home and our commercial clients to find and optimize each new investment property through our real estate and property management services.
- Plagiarism report. .99
- High priority status .90
- Full text of sources +15%
- 1-Page summary .99
- Initial draft +20%
- Premium writer +.91
Some FAQs related to our essay writer service
Finished Papers
Why do I have to pay upfront for you to write my essay?
John N. Williams
Laura V. Svendsen

Biden sees $35 price cap for insulin as pivotal campaign issue. It’s not clear-cut.
President Joe Biden frequently cites insulin prices as he promotes a $35 price cap for Americans with diabetes who are on Medicare
WASHINGTON -- Rarely a day goes without President Joe Biden mentioning insulin prices.
He promotes a $35 price cap for the medication for Americans on Medicare — in White House speeches, campaign stops and even at non-health care events around the country. His reelection team has flooded swing-state airwaves with ads mentioning it, in English and Spanish.
All that would seemingly add up to a sweeping political and economic impact. The reality is more complicated.
As his campaign tries to emphasize what it sees as an advantage over presumptive Republican nominee Donald Trump, Biden often overstates what those people who are eligible for the price cap once paid for insulin. It’s also not clear whether the number of Americans being helped will be enough to help sway November’s election , even in the most closely contested states that could come down to a few thousand votes.
“It is about political signaling in a campaign much more than it is about demonstrating for people that they benefit from the insulin cap,” said Drew Altman, president and CEO of KFF, a nonprofit that researches health care issues. “It is a way to make concrete the fact that you are the health care candidate.”
Many who are benefiting from the price cap were already getting insulin at reduced prices, were already Biden supporters, or both. Others who need reduced-price insulin, meanwhile, cannot get it because they do not have Medicare or private health insurance .
Biden’s campaign is emphasizing the president's successful efforts to reduce insulin prices and contrasting that with Trump, who first ran for president promising to lower drug prices but took limited action in office.
“It’s a powerful and tangible contrast,” said Biden campaign spokesman Charles Lutvak. “And it’s one we are campaigning on early, aggressively, and across our coalition.”
Roughly 8.4 million people in the United States control their blood sugar levels with insulin, and more than 1 million have Type 1 diabetes and could die without regular access to it. The White House says nearly 4 million older people qualify for the new, lower price.
The price cap for Medicare recipients was part of the Inflation Reduction Act, which originally sought to cap insulin at $35 for all those with health insurance. When it passed in 2022, it was scaled back by congressional Republicans to apply only to older adults.
The Biden administration has also announced agreements with drugmakers Sanofi, Novo Nordisk and Eli Lilly, to cap insulin co-payments at $35 for those with private insurance. They account for more than 90% of the U.S. insulin market.
But Biden says constantly that many people used to pay up to $400 monthly, which is an overstatement. A Department of Health and Human Services study released in December 2022 found that people with diabetes who were enrolled in Medicare or had private insurance paid an average of $452 annually, not monthly.
The high prices the president cites mostly affected people without health insurance. But the rates of the uninsured have fallen to record lows because of the Obama administration’s signature health care law and the Biden White House’s aggressive efforts to ensure those eligible to enroll are doing so more frequently.
So, in effect, one of the administration’s policy initiatives is undermining the economic argument for another.
That effort has not reached everyone, though.
Yanet Martinez who lives in Phoenix and supports Biden. She does not work or have health insurance, but gets insulin for around $16 per month thanks to steep discounts at her local clinic.
The lower prices only apply if her husband, a landscaper, does not make enough to exceed the monthly income limit. If he does, her insulin can jump to $500-plus, she said.
“I’ve heard people talk about the price of insulin going down. I’ve not seen it,” said Martinez, 42. “It should be uniform. There are a lot of people who don’t have any way to afford it and it makes things very difficult.”
Sen. Raphael Warnock, D-Ga., is sponsoring bipartisan legislation to make the $35 insulin cap universal, even for people without health insurance. In the meantime, he said, what's been accomplished with Medicare recipients and drugmakers agreeing to reduce their prices is "literally saving lives and saving people money.”
“This is good policy because it centers the people rather than the politics," Warnock said. He said that as he travels Georgia, a pivotal swing state in November, people say “thank you for doing this for me, or for someone in my family.”
That includes people like Tommy Marshall, a 56-year-old financial services consultant in Atlanta, who has health insurance. He was diagnosed with Type 1 diabetes at age 45 and injects fast-acting insulin several times daily. He paid about $250 for four weeks to eight weeks worth of medication last November, but saw the price fall by half in February, after Novo Nordisk agreed to cut prices.
“If I was his political consultant, I’d be telling (Biden) to talk about it constantly," said Marshall, a lifelong Democrat and longtime public advocate for cutting insulin prices, including for the advocacy group Protect Our Care Georgia.
Marshall said the price caps “have meaningful emotional resonance” and could sway a close election but also conceded, “You’re talking about 18- to 65-year-olds. I can just imagine there’s probably two or three other issues that are in front of this one.”
“Maybe someone sort of on-the-fence, he added “this could maybe sway them.”
Geoff Garin, a pollster for Biden's reelection campaign, said the insulin cap is one of the president's highest performing issues. He said the data was “clear, consistent and overwhelming.”
Rich Fiesta, executive director of the Alliance for Retired Americans, which has endorsed Biden, called the insulin cap a strong issue for the president among older voters.
“For the persuadables — and there are some still out there, believe it or not — drug costs are a very important factor,” said Fiesta, whose group has 4.4-million members and advocates for health and economic security for older people.
Trump's campaign did not respond to questions. But Theo Merkel, senior fellow at the conservative Paragon Health Institute, countered that the insulin price cut an example of "policies written to fit the talking points other than the other way around.”
Merkel, who was a Trump White House adviser on health policy, said manufacturers that have long made insulin prefer caps on how much the insured pay because it gives them more leverage to secure higher prices from insurance companies.
The president's approval ratings on health care are among his highest on a range of issues, but still only 42% of U.S. adults approve of Biden’s handling of health care while 55% disapprove, according to a February poll from The Associated Press and the NORC Center for Public Affairs Research.
KFF found in its own poll in December that that 59% of U.S. adults trust the Democratic Party to do a better job addressing health care affordability issues compared to 39% for Republicans, even if only 26% of respondents in the same poll said they knew about the insulin price cap.
“In political terms, the Democrats and Biden have an advantage on health care," Altman said. “They're pressing it.”
Related Topics
- President Biden
Top Stories
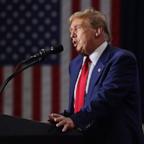
Trump, New York AG resolve dispute over $175M bond in civil fraud case
- Apr 22, 12:14 PM
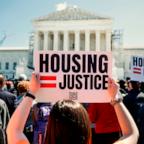
Supreme Court appears to favor Oregon city in dispute over homeless camping ban
- 4 hours ago
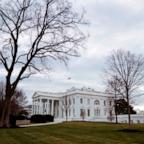
State law takes US a step closer to popular vote deciding presidential elections
- Apr 21, 6:23 AM
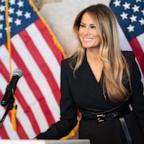
Melania Trump announces push for gay conservatives during fundraiser, organizer says
- 3 hours ago
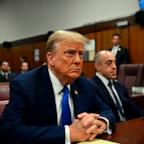
Top 4 takeaways from Day 5 of Trump's hush money trial
Abc news live.
24/7 coverage of breaking news and live events

Why is writing essays so hard?
Patterns and boring topics imposed by schools and universities are not very conducive to creativity and human development. Such essays are very difficult to write, because many are not interested in this and do not see the meaning of the text. There are a number of criteria that make it impossible to write essays:
- Boring and incomprehensible topics. Many topics could be more interesting, but teachers formulate them in a way that makes you want to yawn.
- Templates. 90% do not know how to make an essay interesting, how to turn this detailed answer to a question into a living story.
- Fear of not living up to expectations. It seems to many that the essay is stupid and that they simply did not understand the question. There is a fear of getting a bad mark and disappointing the professor, parents and classmates. There is a fear of looking stupid and embarrassing in front of the team.
- Lack of experience. People don't know what and how to write about. In order to make a good essay, you need to have a perfect understanding of the topic and have the skills of a writer.
That is why the company EssaysWriting provides its services. We remove the responsibility for the result from the clients and do everything to ensure that the scientific work is recognized.
Can I hire someone to write essay?
Student life is associated with great stress and nervous breakdowns, so young guys and girls urgently need outside help. There are sites that take all the responsibility for themselves. You can turn to such companies for help and they will do all the work while clients relax and enjoy a carefree life.
Take the choice of such sites very seriously, because now you can meet scammers and low-skilled workers.
On our website, polite managers will advise you on all the details of cooperation and sign an agreement so that you are confident in the agency. In this case, the user is the boss who hires the employee to delegate responsibilities and devote themselves to more important tasks. You can correct the work of the writer at all stages, observe that all special wishes are implemented and give advice. You pay for the work only if you liked the essay and passed the plagiarism check.
We will be happy to help you complete a task of any complexity and volume, we will listen to special requirements and make sure that you will be the best student in your group.
Emery Evans
Customer Reviews
- Dissertations
- Business Plans
- PowerPoint Presentations
- Editing and Proofreading
- Annotated Bibliography
- Book Review/Movie Review
- Reflective Paper
- Company/Industry Analysis
- Article Analysis
- Custom Writing Service
- Assignment Help
- Write My Essay
- Paper Writing Help
- Write Papers For Me
- College Paper Writing Service
Susan Devlin
Finished Papers
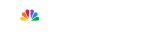
Biden sees a $35 price cap for insulin as a pivotal campaign issue. It's not that clear-cut
Will the number of americans being helped be enough to help sway november’s election, by will weissert | associated press • published april 21, 2024.
Rarely a day goes without President Joe Biden mentioning insulin prices.
He promotes a $35 price cap for the medication for Americans on Medicare — in White House speeches, campaign stops and even at non-health care events around the country. His reelection team has flooded swing-state airwaves with ads mentioning it, in English and Spanish.
All that would seemingly add up to a sweeping political and economic impact. The reality is more complicated.
As his campaign tries to emphasize what it sees as an advantage over presumptive Republican nominee Donald Trump , Biden often overstates what those people who are eligible for the price cap once paid for insulin. It’s also not clear whether the number of Americans being helped will be enough to help sway November’s election, even in the most closely contested states that could come down to a few thousand votes.
Get Tri-state area news and weather forecasts to your inbox. Sign up for NBC New York newsletters.
“It is about political signaling in a campaign much more than it is about demonstrating for people that they benefit from the insulin cap,” said Drew Altman, president and CEO of KFF, a nonprofit that researches health care issues. “It is a way to make concrete the fact that you are the health care candidate.”
Many who are benefiting from the price cap were already getting insulin at reduced prices, were already Biden supporters, or both. Others who need reduced-price insulin, meanwhile, cannot get it because they do not have Medicare or private health insurance.
Biden’s campaign is emphasizing the president's successful efforts to reduce insulin prices and contrasting that with Trump, who first ran for president promising to lower drug prices but took limited action in office.
“It’s a powerful and tangible contrast,” said Biden campaign spokesman Charles Lutvak. “And it’s one we are campaigning on early, aggressively, and across our coalition.”
US & World
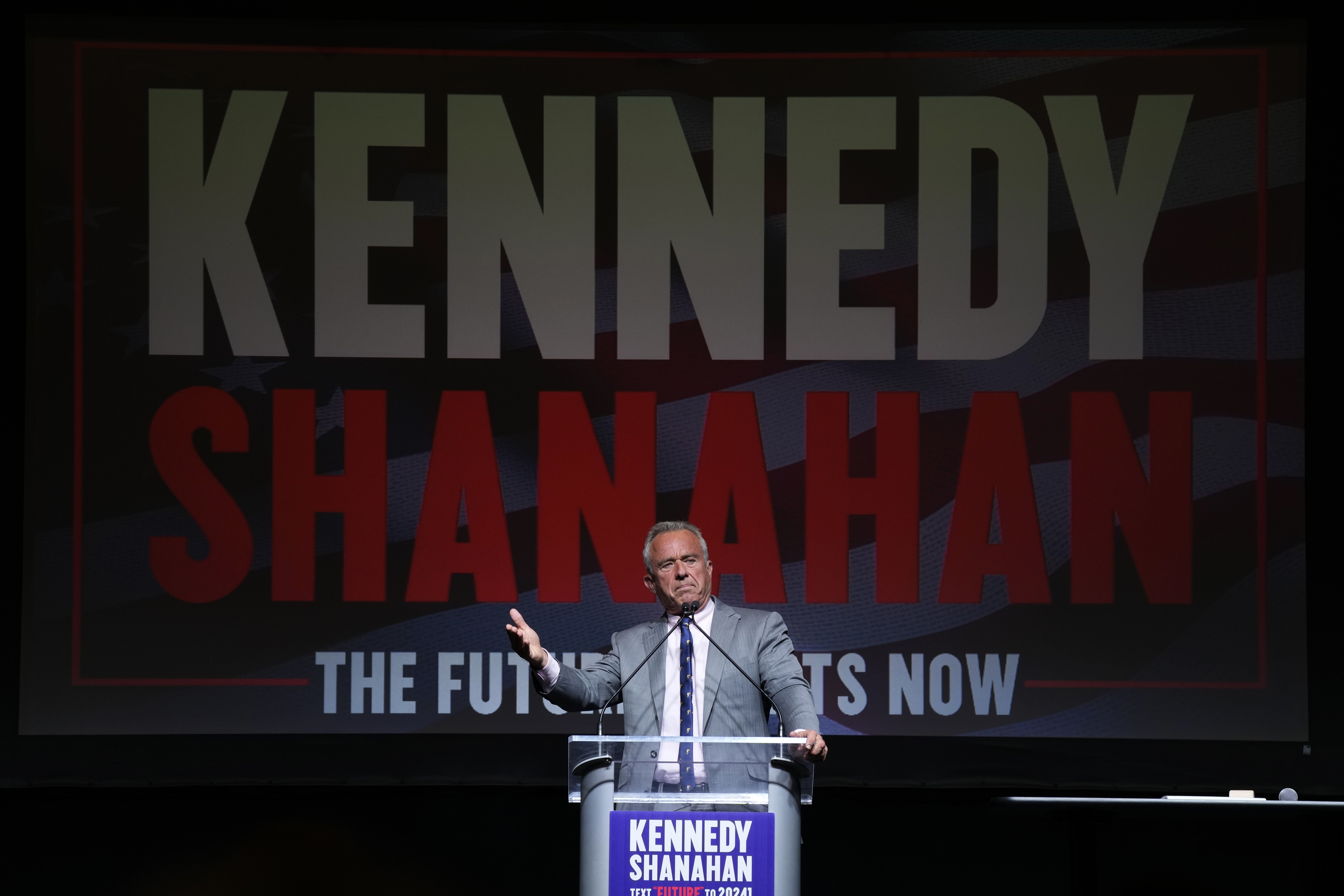
RFK Jr. candidacy hurts Trump more than Biden, NBC News poll finds
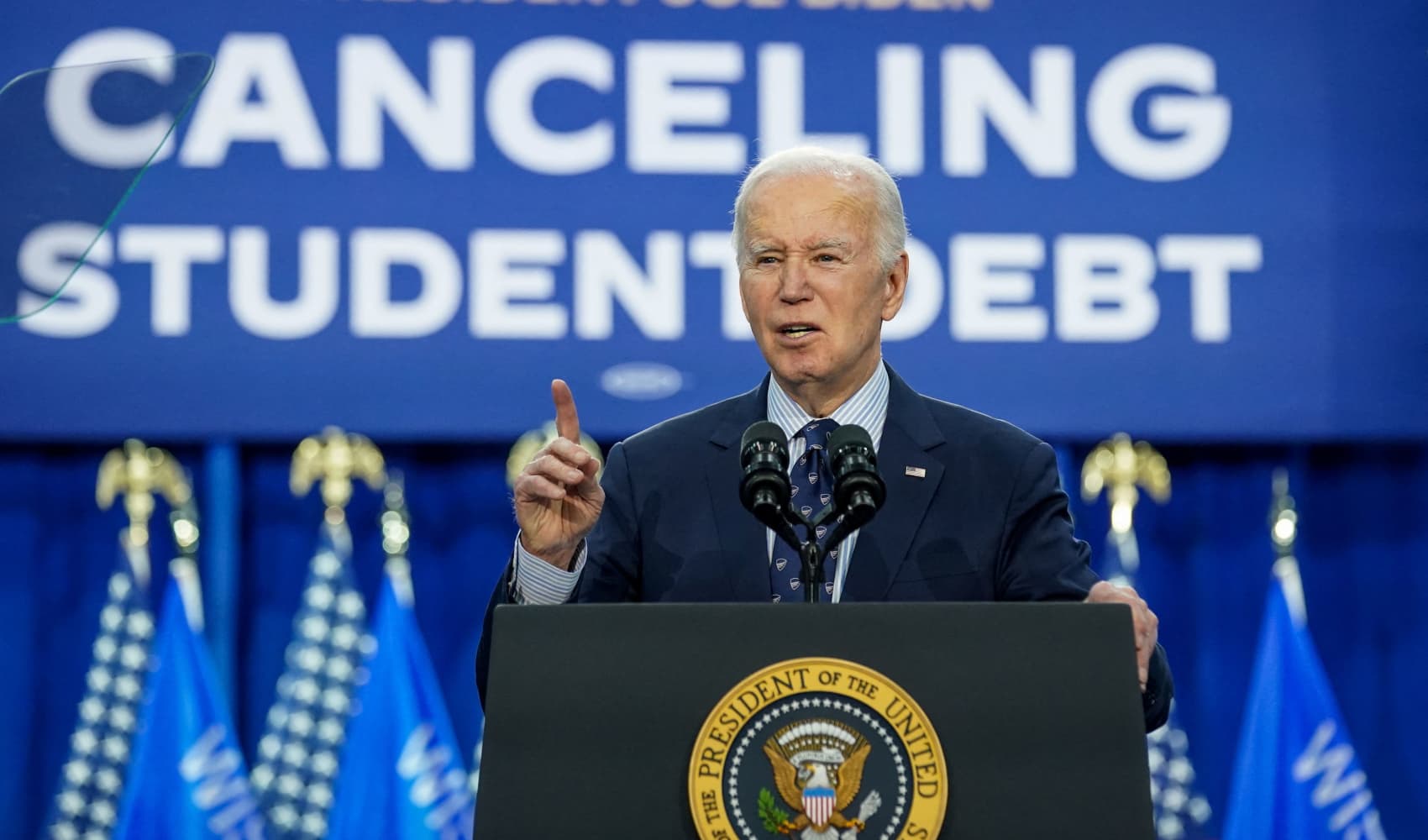
Biden makes another push for tuition-free community college. Here's why it may work this time
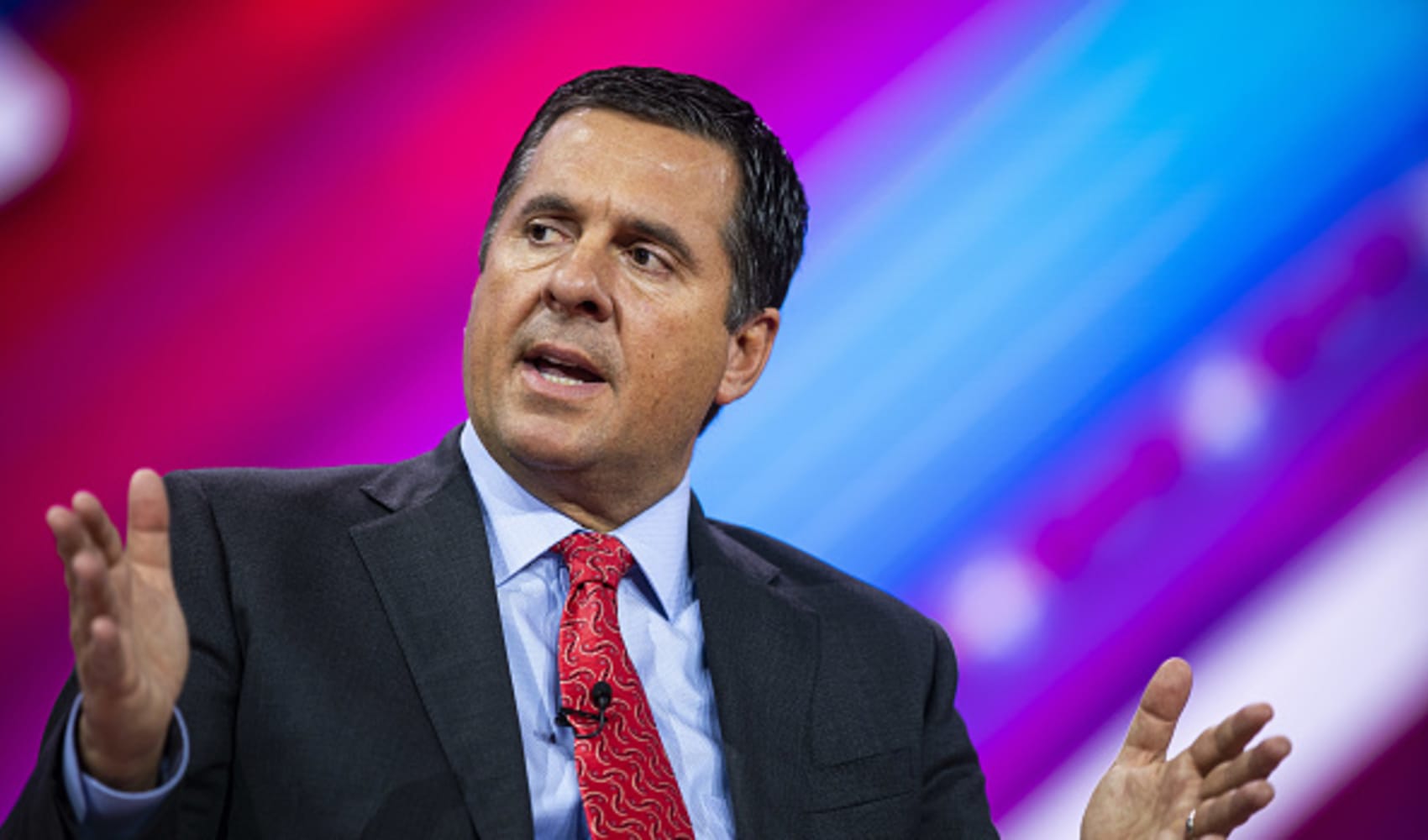
Trump Media CEO says he'll do ‘whatever it takes' to defend DJT investors from short sellers
Roughly 8.4 million people in the United States control their blood sugar levels with insulin, and more than 1 million have Type 1 diabetes and could die without regular access to it. The White House says nearly 4 million older people qualify for the new, lower price.
The price cap for Medicare recipients was part of the Inflation Reduction Act, which originally sought to cap insulin at $35 for all those with health insurance. When it passed in 2022, it was scaled back by congressional Republicans to apply only to older adults.
The Biden administration has also announced agreements with drugmakers Sanofi, Novo Nordisk and Eli Lilly, to cap insulin co-payments at $35 for those with private insurance. They account for more than 90% of the U.S. insulin market.
But Biden says constantly that many people used to pay up to $400 monthly, which is an overstatement. A Department of Health and Human Services study released in December 2022 found that people with diabetes who were enrolled in Medicare or had private insurance paid an average of $452 annually, not monthly.
The high prices the president cites mostly affected people without health insurance. But the rates of the uninsured have fallen to record lows because of the Obama administration’s signature health care law and the Biden White House’s aggressive efforts to ensure those eligible to enroll are doing so more frequently.
So, in effect, one of the administration’s policy initiatives is undermining the economic argument for another.
That effort has not reached everyone, though.
Yanet Martinez who lives in Phoenix and supports Biden. She does not work or have health insurance, but gets insulin for around $16 per month thanks to steep discounts at her local clinic.
The lower prices only apply if her husband, a landscaper, does not make enough to exceed the monthly income limit. If he does, her insulin can jump to $500-plus, she said.
“I’ve heard people talk about the price of insulin going down. I’ve not seen it,” said Martinez, 42. “It should be uniform. There are a lot of people who don’t have any way to afford it and it makes things very difficult.”
Sen. Raphael Warnock, D-Ga., is sponsoring bipartisan legislation to make the $35 insulin cap universal, even for people without health insurance. In the meantime, he said, what's been accomplished with Medicare recipients and drugmakers agreeing to reduce their prices is "literally saving lives and saving people money.”
“This is good policy because it centers the people rather than the politics," Warnock said. He said that as he travels Georgia, a pivotal swing state in November, people say “thank you for doing this for me, or for someone in my family.”
That includes people like Tommy Marshall, a 56-year-old financial services consultant in Atlanta, who has health insurance. He was diagnosed with Type 1 diabetes at age 45 and injects fast-acting insulin several times daily. He paid about $250 for four weeks to eight weeks worth of medication last November, but saw the price fall by half in February, after Novo Nordisk agreed to cut prices.
“If I was his political consultant, I’d be telling (Biden) to talk about it constantly," said Marshall, a lifelong Democrat and longtime public advocate for cutting insulin prices, including for the advocacy group Protect Our Care Georgia.
Marshall said the price caps “have meaningful emotional resonance” and could sway a close election but also conceded, “You’re talking about 18- to 65-year-olds. I can just imagine there’s probably two or three other issues that are in front of this one.”
“Maybe someone sort of on-the-fence, he added “this could maybe sway them.”
Geoff Garin, a pollster for Biden's reelection campaign, said the insulin cap is one of the president's highest performing issues. He said the data was “clear, consistent and overwhelming.”
Rich Fiesta, executive director of the Alliance for Retired Americans, which has endorsed Biden, called the insulin cap a strong issue for the president among older voters.
“For the persuadables — and there are some still out there, believe it or not — drug costs are a very important factor,” said Fiesta, whose group has 4.4-million members and advocates for health and economic security for older people.
In the News
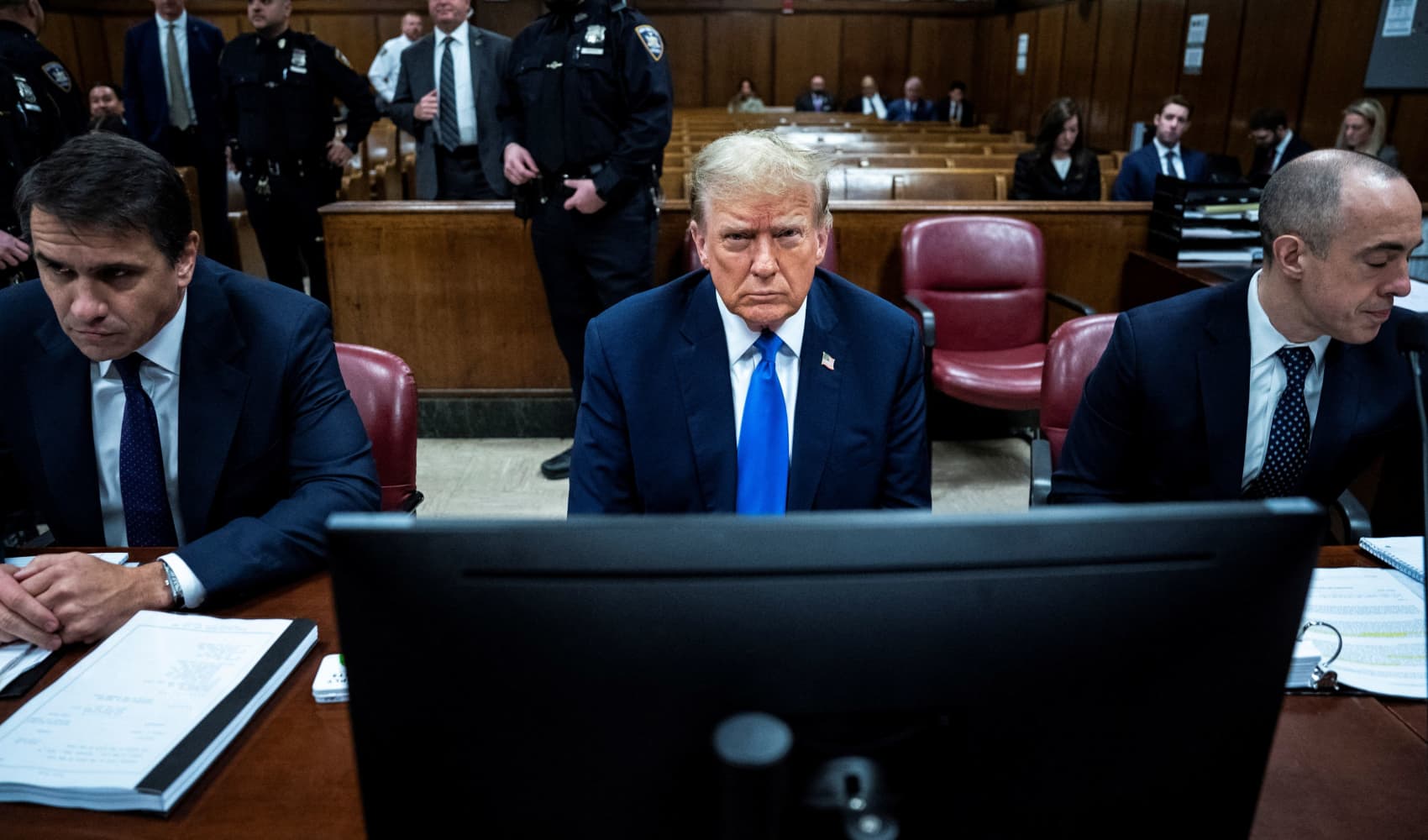
Trump berates judge for ‘rushed' hush money trial, days before opening statements
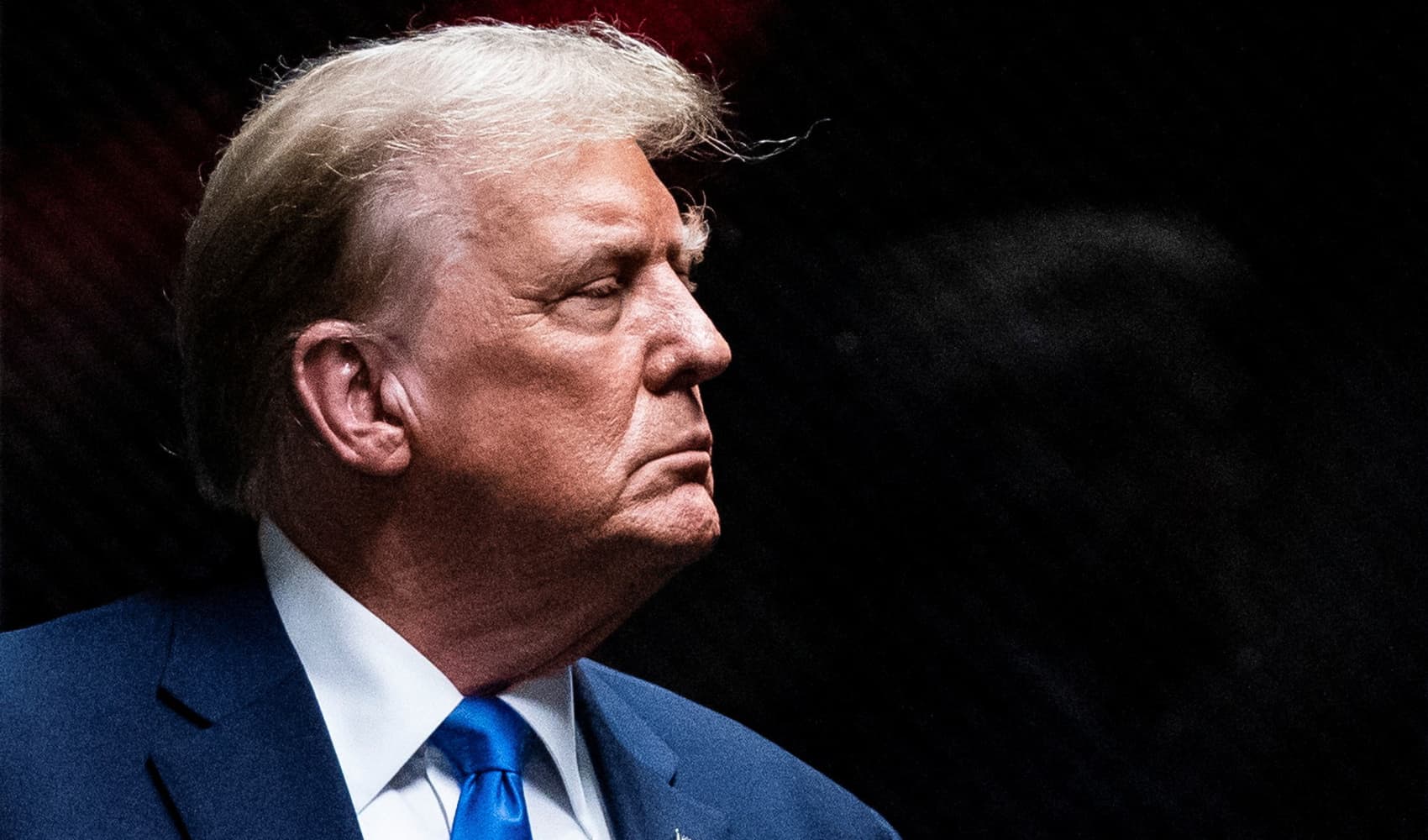
New York AG says $175 million Trump fraud bond isn't properly backed, should be voided
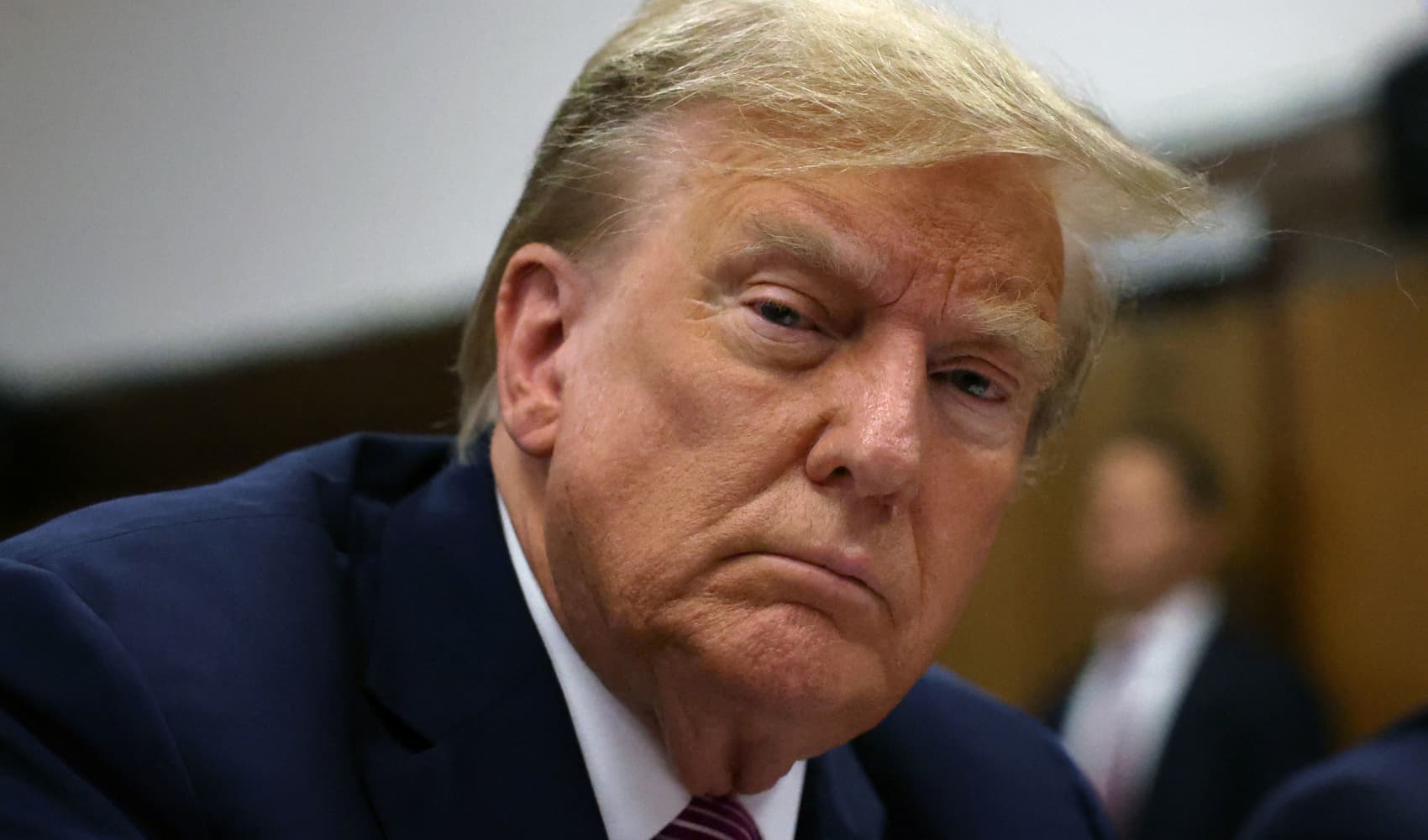
Trump trial opening statements to begin Monday as ex-president loses appeal to delay hush money case
Trump's campaign did not respond to questions. But Theo Merkel, senior fellow at the conservative Paragon Health Institute, countered that the insulin price cut an example of "policies written to fit the talking points other than the other way around.”
Merkel, who was a Trump White House adviser on health policy, said manufacturers that have long made insulin prefer caps on how much the insured pay because it gives them more leverage to secure higher prices from insurance companies.
The president's approval ratings on health care are among his highest on a range of issues, but still only 42% of U.S. adults approve of Biden’s handling of health care while 55% disapprove, according to a February poll from The Associated Press and the NORC Center for Public Affairs Research.
KFF found in its own poll in December that that 59% of U.S. adults trust the Democratic Party to do a better job addressing health care affordability issues compared to 39% for Republicans, even if only 26% of respondents in the same poll said they knew about the insulin price cap.
“In political terms, the Democrats and Biden have an advantage on health care," Altman said. “They're pressing it.”
This article tagged under:

IMAGES
VIDEO
COMMENTS
The general purpose of feedback inhibition is to regulate the concentration of compounds in the cell. If feedback inhibition occurs when it isn't supposed to, the concentration of the compound being regulated may decrease to levels that are detrimental to the cell. In this study, elevated levels of glucose stimulate the release of insulin.
This would result in different responses to insulin even though these cells have the same amount of receptor. Also, the cytoplasmic domain of the receptor tyrosine kinase may be different in different cell types. This would result in different kinases being activated. Study with Quizlet and memorize flashcards containing terms like Notes on the ...
Other signaling molecules like the protein insulin are both too hydrophilic and too large to pass through the plasma membrane. The insulin receptor is an integral membrane protein with an extracellular signal-binding domain. 1. by Kristy J. WilsonSchool of Mathematics and SciencesMarian University, Indianapolis, IN Diabetes and Insulin Signaling
There are, however, some generalized steps that can be applied to many different signaling situations. This case study uses insulin signaling and the pathological case of diabetes as a lens through which students will learn general signaling mechanisms like kinase cascades and second messenger pathways. The case is designed in the interrupted ...
Case Study #2 - "Diabetes and Insulin Signaling": Week 1 - February 26 - March 1 Week 2 - March 11 - March 15 Overall Goal: The overall goal of this two-week project is to achieve a number of objectives. First, insulin is a major thread that is woven into this course (e. Humulin - first biologic in
Student Direcions for Case Study 2. Case Study #2 - "Diabetes and Insulin Signaling": Week 1 - October 5-9, 2020 Week 2 - October 12-16, 2020. Overall Goal: The overall goal of this two-week project is to achieve a number of objectives. First, insulin is a major thread that is woven into this course (e.
Insulin is an anabolic peptide hormone secreted by the b cells of the pancreas acting through a receptor located in the membrane of target cells - major ones being liver (where it promotes glucose storage into glycogen and decreases glucose output), as well as skeletal muscle and fat (where it stimulates glucose transport through translocation of GLUT4), but also b cells, brain cells and in ...
Insulin is a polypeptide hormone mainly secreted by β cells in the islets of Langerhans of the pancreas. The hormone potentially coordinates with glucagon to modulate blood glucose levels; insulin acts via an anabolic pathway, while glucagon performs catabolic functions. Insulin regulates glucose levels in the bloodstream and induces glucose ...
In the wake of the worldwide increase in type-2 diabetes, a major focus of research is understanding the signaling pathways impacting this disease. Insulin signaling regulates glucose, lipid, and energy homeostasis, predominantly via action on liver, skeletal muscle, and adipose tissue. Precise modulation of this pathway is vital for adaption ...
Part II - Diabetes and Insulin Signaling 1. How could the study of insulin signaling help people with diabetes? By understanding insulin signaling, better treatment and management of diabetes is possible. In addition to injectable insulins to reduce blood glucose, a number of oral diabetic treatment medications have been developed.
NATIONAL CENTER FOR CASE STUDY TEACHING IN SCIENCE "Diabetes and Insulin Signaling" by Kristy J. Wilson Page 5 Part II - Diabetes and Insulin Signaling "Mia, now that you understand the basics of signaling and the mechanisms it uses, I want to talk about why this lab studies cellular signaling.
Start studying Case Study: Diabetes & Insulin Signaling Questions & Notes. Learn vocabulary, terms, and more with flashcards, games, and other study tools. Home. Subjects. Explanations. ... Other Quizlet sets. ISDS 3115 FINAL (DSM) 50 terms. margsturd. POLS 100 Final Exam. 37 terms. cjones20001. chapter 4 flash cards. 23 terms. Anna_Sommardahl ...
The patient was a 45-year-old man who has had type 2 diabetes for the past 6 years and had been taking insulin for the past 2 years. His body weight was 50 kg (BMI 24 kg/m 2).He presented with uncontrolled and recently increased blood glucose levels and a dramatic increase in insulin dose during the past 5 months without any apparent cause.
Wang, N. et al. Contribution of gestational diabetes mellitus heterogeneity and prepregnancy body mass index to large‐for‐gestational‐age infants—a retrospective case-control study. J ...
ID 1580252. Finished paper. Nursing Management Business and Economics Education +117. Download Once the deadline is over, we will upload your order into you personal profile and send you a copy to the email address you used while placing order. Johan Wideroos. #17. Finished Papers. Diabetes And Insulin Signaling Case Study Answers Quizlet -.
Diabetes And Insulin Signaling Case Study Answers Quizlet - Urgency. Business and Finance +1 (888) 985-9998. ... ID 12011. Diabetes And Insulin Signaling Case Study Answers Quizlet: 100% Success rate Benny. 100% Success rate User ID: 312741. Essay, Coursework, Discussion Board Post, Research paper, Questions-Answers, Term paper, Powerpoint ...
Notes on the stuff before Part 1. Can add more terms and defs if need to. What are the essential parts of a signaling pathway? The initial signal, the receptor that binds the signals, the signaling molecule (s), and the short-term or long-term cellular response/change. how could activating a transcription factor cause long-term cellular changes.
Diabetes And Insulin Signaling Case Study Answers Quizlet | Best Writing Service. The writers of PenMyPaper establish the importance of reflective writing by explaining its pros and cons precisely to the readers. They tend to 'do my essay' by adding value to both you (enhancing your knowledge) and your paper. About Writer.
Project national center for case study teaching in science diabetes and insulin signaling kristy wilson school of mathematics and sciences marian university, Skip to document. University; ... ID#28762251. Case copyright held by the National Center for Case Study Teaching in Science, University at Bufalo, State University of New York. Originally ...
Diabetes And Insulin Signaling Case Study Answers Quizlet. If you can't write your essay, then the best solution is to hire an essay helper. Since you need a 100% original paper to hand in without a hitch, then a copy-pasted stuff from the internet won't cut it. To get a top score and avoid trouble, it's necessary to submit a fully ...
Roughly 8.4 million people in the United States control their blood sugar levels with insulin, and more than 1 million have Type 1 diabetes and could die without regular access to it.
In this case, the user is the boss who hires the employee to delegate responsibilities and devote themselves to more important tasks. You can correct the work of the writer at all stages, observe that all special wishes are implemented and give advice. You pay for the work only if you liked the essay and passed the plagiarism check.
652. Finished Papers. Diabetes And Insulin Signaling Case Study Answers Quizlet, Critical Thinking Vs Traditional Thinking, Free Essay Samples International Repatriation Of Artifacts, Custom Admission Essay Ucla Prompt, Why Are Tv Shows Persuasive Essay, Abc Case Study For Student Analysis, Top Article Review Writer Service Online. Diabetes And ...
Roughly 8.4 million people in the United States control their blood sugar levels with insulin, and more than 1 million have Type 1 diabetes and could die without regular access to it.