Thank you for visiting nature.com. You are using a browser version with limited support for CSS. To obtain the best experience, we recommend you use a more up to date browser (or turn off compatibility mode in Internet Explorer). In the meantime, to ensure continued support, we are displaying the site without styles and JavaScript.
- View all journals
- Explore content
- About the journal
- Publish with us
- Sign up for alerts
- Published: 15 October 2018

Sustainable agriculture
Nature Sustainability volume 1 , page 531 ( 2018 ) Cite this article
6682 Accesses
5 Citations
2 Altmetric
Metrics details
- Agriculture
- Environmental impact
- Psychology and behaviour
- Sustainability
Achieving food security is possible, if we better understand the complexity of the agricultural system and re-design practices accordingly.
Early in September, the State of Food Security and Nutrition in the World report was released through a joint press conference at the FAO headquarters in Rome. The analysis ( The State of Food Security and Nutrition in the World FAO; 2018) is the outcome of a close collaboration between five United Nations agencies — the Food and Agriculture Organization, the International Fund for Agricultural Development, the United Nations Children’s Fund (UNICEF), the World Food Programme and the World Health Organization — and one of the key messages from the report is, perhaps not surprisingly, alarming: the world is not on track to meet the ‘Zero Hunger’ Sustainable Development Goal, SDG 2.
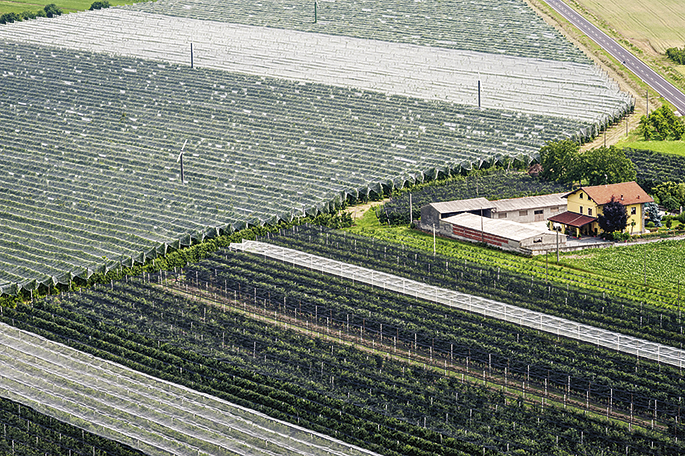
Alex Ramsay/Alamy Stock Photo
Drawing attention to the drivers of hunger and malnutrition, the report includes updated estimates of a number of indicators related to food security and its health implications, including the number of hungry people in the world, data on child stunting, adult obesity, and childhood obesity among others.
The report, which uses data from 2017, shows how hunger has increased worldwide for the third consecutive year, and childhood malnutrition has not improved or, even worse, in some cases has declined. Countries must take urgent action to meet SDG 2 by 2030.
This year’s edition of the annual report focuses on the need to build climate resilience for food security and nutrition. Acknowledging the dependency of our nutritional needs on the natural environment should indeed be a critical component of any food-policy strategy.
More specifically, the sustainability of the food system should be at the heart of the international food-security debate. Despite increasing hunger globally, the demand of food has been rising rapidly and has had a significant environmental cost: degradation of agricultural land, pollution of rivers and aquifers due to agro-chemicals, increased freshwater consumption, greenhouse-gas emissions from agriculture and land-use change, loss of agro-biodiversity and other negative consequences. All of these environmental impacts severely undermine our ability to continue to feed a growing population and ultimately will jeopardize the opportunity to meet SDG 2, unless more-sustainable food-production practices are embraced globally.
Enhanced agricultural productivity (intensification) has been a major response to the growing food demand, but intensification could be done better. In an Analysis by Pretty et al. published in our August issue, the authors underline how environmental considerations in agriculture intensification have been traditionally limited to minimizing negative impacts. Instead, with their analyses, they show that, for example, a move away from fertilizers to nitrogen-fixing legumes as part of rotations or intercropping could improve intensification without increasing environmental stress. Their point is that agriculture intensification can be sustainable if the system is adequately re-designed and if all players involved accept that no new designed system will succeed forever.
Increasing food production can impact conservation strategies — another issue likely to have long-term negative consequences for our ability to provide healthy levels of nutrition to all. Keesing and colleagues, in an Article in this issue, show however that a conflict between the two shouldn’t be the case. They analyse the potential trade-offs between management for wildlife and for livestock in an East African savannah, and find potential ecological and economic benefits from integrating the two.
However, even while considering effective strategies, it remains clear that human activities do affect biodiversity around the world, and that applies to agricultural practices as much as to other activities. In a Brief Communication in the August issue, Mehrabi et al. analyse the implications of the conservationists’ proposal to give back half the Earth’s surface to nature (the ‘Half-Earth’ project). Among other results, they find that, depending on the landscape conservation strategy, 23–25% of non-food calories and 3–29% of food calories from crops globally could be lost if the proposal were implemented. They do show that the trade-offs between agriculture and the Half-Earth proposal will be much lower if landscapes remain mosaics of shared land uses.
So, what are we left with? We can certainly improve agricultural practices as discussed earlier in this Editorial, and increase their sustainability. Will it be enough to achieve our societal goals? Perhaps we need to also look at our individual behaviour. We know that we need to manage our dependence on nature sustainably. And sustainable management hinges on deep understanding of human–nature relationships. Behavioural sciences can bring invaluable insights to our ways of mapping the complexity of such relationships. Going back to the sustainability of agricultural practices, the different ways in which individuals’ mindsets represent a system and the causal relationships among its components (mental models) can capture more or less complexity, as discussed in an Article by Levy and colleagues in our August issue. The authors show that, for example, mental models characterized by direct, unidirectional causation allow fast decision-making but might fail to anticipate consequences of actions in the presence of strong interdependences. Understanding these cognitive mechanisms has important implications for individual and collective decision-making about sustainable agriculture. And that is crucial to better orient food-security strategies. With the right set of changes in practices and interventions, informed by academic research and practice, there is still hope to achieve SDG 2 on time.
Rights and permissions
Reprints and permissions
About this article
Cite this article.
Sustainable agriculture. Nat Sustain 1 , 531 (2018). https://doi.org/10.1038/s41893-018-0163-4
Download citation
Published : 15 October 2018
Issue Date : October 2018
DOI : https://doi.org/10.1038/s41893-018-0163-4
Share this article
Anyone you share the following link with will be able to read this content:
Sorry, a shareable link is not currently available for this article.
Provided by the Springer Nature SharedIt content-sharing initiative
Quick links
- Explore articles by subject
- Guide to authors
- Editorial policies
Sign up for the Nature Briefing: Anthropocene newsletter — what matters in anthropocene research, free to your inbox weekly.

- Research Article
- Open access
- Published: 19 August 2020
Participatory research for sustainable agriculture: the case of the Italian agroecological rice network
- Elena Pagliarino ORCID: orcid.org/0000-0001-6140-3856 1 ,
- Francesca Orlando 2 ,
- Valentina Vaglia 2 ,
- Secondo Rolfo 1 &
- Stefano Bocchi 2
European Journal of Futures Research volume 8 , Article number: 7 ( 2020 ) Cite this article
17k Accesses
19 Citations
1 Altmetric
Metrics details
Since the Green Revolution, worldwide agriculture has been characterized by a typical top–down approach. The degree of autonomy, creativity, and responsibility of farmers has been limited by the continuous external inputs of chemicals, machinery, advice, subsidies and knowledge.
The issue of sustainability has brought complexity and uncertainty to this mainly linear process of innovation, steering agriculture toward alternative models. Agroecology represents an innovative paradigm of agriculture in which external inputs are minimized, and the assets of the farm are greatly valued. Agroecological production relies on the farmers’ direct management of resources and on their active engagement in the agricultural knowledge and innovation system.
This paper focuses on the experience of a group of farmers, scientists, public officials, and managers of private companies who are experimenting with agroecology in rice production in one of the most intensively farmed, profitable and environmentally sensitive areas of Italy. The partnership regularly comes together to discuss agricultural techniques and results, needs, and paths of innovation; in addition, it stimulates and takes part in research projects, following a participatory process based on co-learning and mutual responsibility. By using ethnographic methods such as direct observations and in-depth interviews, our work may contribute to understanding the role of participatory research in sustainable agriculture and what makes for good participation.
Introduction
The traditional model of innovation and its failings.
From the so-called Green Revolution, started in the 1950s, to the current period of innovations based on digital devices, worldwide agriculture has been characterized by a typical top–down transfer of technology. In this pervasive paradigm, technology is developed in the controlled environment of universities and research stations, passed on to agricultural advisors and then to farmers, who consume and apply it ([ 18 ]: 67). Technology is perceived as a commodity delivered to farmers, who have little control over its development and management [ 22 ]. The transferred technologies are uniform, standardized, and mass-produced to work almost everywhere. Standardization is applied not only to physical technologies, such as seeds, pesticides, and machinery, but also to procedures and their sequencing, with the aim of routinizing the activities of farmers, thus promoting predictable and manageable changes in rural areas ([ 18 ]: 71). Some feedback is provided by the extension agents, who turn the problems of the farmers into researchable questions, then answered by research scientists. Nevertheless, the innovation pipeline is mainly linear and one-way [ 82 ].
This system has improved the availability and quality of food per capita, ensuring food security in many areas of the world [ 72 ], and it has been a powerful tool for the diffusion of industrial agriculture [ 81 ].
While this traditional model is still practiced in many areas, its shortcomings have long been acknowledged. The reliance of farmers on suppliers of technologies, capital to buy such technologies and experts’ knowledge to be able to use them has grown, limiting their margins of autonomy and creativity in farming decisions. They have also lost control over essential resources due to the concentration of power in the mechanical, seed, chemical, processing, and distribution industries. With the introduction of advanced techniques, such as genetic engineering, nanotechnology, precision agriculture, sensors, satellites, and robotics, innovation has become increasingly sophisticated and its development even more disconnected from farmers.
Chambers, Pretty and other practitioners of the “farmer first” discourse [ 16 , 17 , 77 , 78 ] have highlighted the failure of this model in developing countries and resource-poor areas, which are more risk-prone and characterized by more complex and less controllable local conditions than the areas where the technologies and practices were actually developed.
The challenge of sustainability, posed first by the Report of the Club of Rome in 1972 and then by the Brundtland Report in 1987 and the Rio Declaration in 1992, started to be perceived as an issue only at the end of the last century [ 91 ], when it brought complexity to intensive agriculture, practiced in more developed countries. The issue of sustainability has brought to the fore the concepts of risk and uncertainty also in European agriculture. Risk and uncertainty are critical matters in agriculture and, therefore, their impact on both learning and practice needs to be taken into account. Dealing with environmental risks and developing innovations to address these risks require more inclusive ways of knowing and doing, as noted by Pimbert ([ 75 ]: 22), who stated that “more inclusive ways of knowing are required to bring together the partial and incomplete perspectives of different actors faced with uncertainty, diversity and change”. This is the reason why the participatory research approach has been incorporated into European agricultural research, increasingly oriented toward the challenge of sustainability, albeit lagging behind other sectors (for example, ecosystem management, which started soon after the Rio Declaration and Agenda 21, in 1992).
Criticism of the mechanistic process of innovation has extended to all farming systems, while a broad consensus has emerged on the links between conventional agriculture and its top–down innovation, on the one hand, and the environmental crisis, on the other hand.
The agroecological paradigm based on participation
Agroecology has been proposed as a radical alternative to the Green Revolution [ 1 , 2 , 38 , 87 , 94 ]. It represents an innovative paradigm of agriculture in which external inputs are minimized, and great value is attached to the internal resources of the farm and the territory. A systemic ecological approach is adopted in order to understand the relations between living organisms and their environment. This fosters the processes that move the agroecosystem closer to a natural, mature, relatively stable, and self-sustaining ecosystem, able to maintain productivity without external inputs [ 37 ].
Our work does not explore the issue of agroecology seen as a social movement but focuses exclusively on agroecology as a system of knowledge and innovation. In this meaning, agroecological production relies on the farmers’ direct management of resources and on their engagement in the governance of the agricultural knowledge and innovation system. Proponents of agroecology as an alternative development model argue that its potential can only be realized through participatory research and extension [ 16 , 83 , 84 , 94 , 96 ]. Cuéllar-Padilla and Calle-Collado [ 22 ] define agroecology as “the practice of science with people” and stress that participation is at the core of any single process. Agroecology implies the promotion of practices that (i) fit the local contexts in which they are implemented, (ii) foster the autonomy and skills of the communities involved (as is the case with the participatory research network discussed in our case study, whose learning and empowerment processes are presented in Section 3.2), (iii) profit from locally-produced resources, included local opinions regarding sustainability ( Ibidem ).
A young male farmer of our network explains: “It is a question of development model. So, if we choose a development model that favors indistinct, undifferentiated production—a commodity, as it is called—this leads to cost increases. The progressive increase in costs combines with stagnation in terms of value generated by the production. To deal with decreasing revenues, one must increase the surface area. This model breaks up the farming community because the land is a finite good. If ten farmers work this land today but the model forces me to expand, some farms will grow but some others will inevitably disappear. This is entrepreneurial desertification in farming. Conversely, the organic agriculture model restores the intrinsic value of what it produces because it qualifies it and, mind you, it is not a matter of profiting excessively, of setting prices that consumers can’t afford, the question is how to redistribute wealth along the production chain. Thanks to the organic system, I do this work and contribute to increasing the biodiversity of the local farming businesses.”
Agroecological research requires local-scale and action-oriented solutions to deal with technical and ecological aspects, as well as economic and sociocultural dimensions, adopting a holistic perspective on agricultural management. The research approach needs to integrate scientific and empirical knowledge throughout the process, achieving the co-production of new cross-cultural innovation [ 15 , 36 , 73 ].
A university professor of the network explains: “In traditional agronomic research, we are limited to comparing fertilizers and antiparasitic agents. We decontextualize, we only look at parcels, we compare in increasing doses, we add a witness, we add replications, we use well-documented and refined statistics, we publish, and then we entrust the best technique to the extension service. The best result obtained on the parcel must necessarily also be the best result on the farm. In case of failure, we put the blame on the farmer. This is the game. Impact is not assessed, indirect effects are not considered, especially on a territorial scale. But wasn’t agronomy born along with agriculture? Agronomy is life, creativity, the daily toil of those who work the land, it is not exclusively science. The real challenge lies in complexity. But all the actors have to be involved. It might seem like a longer path, but it is actually much shorter. It is the theory of interconnections, of evolution not based on competition but rather on cooperation.”
A male farmer says: “Farmers are researchers by nature, but with a great limitation: they don’t bother taking notes. They are not interested in writing, so they don’t bother publishing the discoveries coming from their ability to adapt during agronomy activities. In the network, instead, we had to do this. We had to take notes and then discuss them with the others, even the professors, on an equal footing.”
The European Commission has explicitly encouraged the transition to sustainable farming through interactive innovation and multi-actor approaches since 2012 [ 28 ], when the European Innovation Partnership for Agricultural Productivity and Sustainability (EIP-AGRI) and its Operational Groups were launched within the Common Agricultural Policy (CAP). Multi-actor projects and bottom-up thematic networks were also designed within the Horizon 2020 research and development (R&D) framework program. The common principle was to bring together innovation actors: farmers, advisers, researchers, businesses, NGOs, and others. The collaboration among them was supposed to make the best use of complementary types of knowledge, so as to achieve the co-creation and diffusion of solutions and opportunities that would be readily implementable in practice.
In Italy, the Ministry of Agricultural Policies [ 62 ] expressed its intention to support participatory and multi-actor projects in Action 10 of the National Strategic Plan for the Development of the Organic System, emphasising the importance of knowledge sharing, co-research and co-innovation through the involvement of various stakeholders, starting from the initial phases of research. In the call for R&D projects in organic agriculture at the end of 2018, these goals were actively pursued by requiring researchers who wished to receive financial support to include at least one farmer among their research partners and by rewarding those researchers who involved more than one farmer (Ministerial Decree no. 67374/2018).
Participatory networks have multiplied in recent years, activated as part of projects, on the basis of public co-financing. Their diffusion is strengthened by the supporting environment, that is, by the facilitation, coordination, and training processes implemented [ 34 ]. Yet, facilitating dialogue between researchers and farmers is still a priority, which will be pursued in European agricultural policy after 2020 [ 26 ].
Mansuri and Rao [ 55 ] warn that “induced” participation—that is, participation promoted through bureaucratically managed research and development interventions—requires a fundamentally different approach, one that is long-term, context sensitive, and committed to developing a culture of learning by doing. This is why it is particularly interesting to study the experience of a spontaneous, self-directed, and fairly informal, yet highly functional network that seems to be a unique case in the Italian agricultural sector.
What is true participation?
The term “participatory research” is used to refer to various approaches and methods, and it encompasses different types of participation. A systematic review of thirty-five experiences of participatory processes, with the involvement of farmers, concluded that farmers are too often considered a mere source of information to be used by researchers rather than active participants in the management and transformation of rural areas [ 57 ].
As for participatory methods, many authors stress the importance of research mechanisms and designs to bring together scientific and practical knowledge [ 22 , 35 , 50 , 56 , 65 , 99 ]. Successful participatory research, it is argued, can be achieved through a structured dialogue in which the dialectical process is encouraged by regular meetings, joint reflection, and the collective development of findings and conclusions. Nevertheless, the review by Menconi et al. [ 57 ] shows that there is no preferred scheme: every initiative has its own methods and practices and is tailor-made on the researchers’ preferences, resources, context, and project. However, simplicity of approach seems to be the best quality of any participatory activity ( Ibidem ).
As for the attitude and behavior of researchers regarding participation, the literature indicates a widespread lack of awareness, interest, time, incentives, and recognition by the current research system (e.g., [ 13 , 25 , 70 ]). Agricultural scientists have been put under growing pressure to undertake participatory research, but they do not have sufficient practice, skills, and competencies ( Ibidem ). Several authors have suggested blending various forms and intensities of stakeholders’ participation with formal agricultural research (e.g., [ 52 ]), “uniting science and participation” [ 76 ], into “compromised participation” [ 12 ], making things even more difficult in terms of designing, implementing, and monitoring participatory research.
Finally, in addition to the discussion around what participation is, some authors have questioned its very value, raising the issues of inclusion, power, and governance of participation [ 20 , 43 , 44 , 55 , 63 ].
Despite continuous attention paid to the topic, there is no consensus as to what participation means, how widespread it is, whether it is a sufficient goal in sustainable agriculture, and the extent to which it is actually inclusive.
Here, the experience of an Italian network for participatory research in agroecological rice production is presented with the aim to contribute to such ongoing debate. This paper focuses on the role of participatory research in the transition to sustainable agriculture, trying to shed light on if and why it is needed and what makes for good participation.
Study context: the difficult conversion to organic farming of the rice district in Northern Italy
Italy is the leading European producer of rice [ 31 ]. The crop is grown mainly in Northern Italy, mostly in the regions of Piedmont and Lombardy, where a rich, well-organized, and interconnected district comprises farms, processing and distribution businesses, research centers and extension services, and suppliers of chemicals, seeds, and machinery [ 14 ].
The cultivation is typically intensive monoculture, without crop rotation and with heavy chemical inputs, such as fertilizers and pesticides. The impact of rice growing on the environment tends to be considered very high, especially in terms of quality of soil and surface and ground water, with risks to human health posed by drinking contaminated water [ 45 ]. The transition to organic rice farming is perceived as a solution to ensure environmental protection, economic sustainability of the farms, consumer safety, and as a measure to mitigate climate change [ 41 , 80 ].
In Italy, organic farming is regarded as the most advanced and efficient way to develop an agroecological approach [ 68 ], and the discipline of agroecology finds concrete application in organic production, regardless of whether it is certified and remunerated on the market [ 98 ]. Hence, in the remainder of this study, the concepts of agroecology and organic farming will be used interchangeably.
The principles and approaches that should be adopted to manage organic farming systems are shown in European Commission (EC) Regulation 848/2018 (art. 6 and Annex II) [ 29 ]: limiting the use of non-renewable resources and external inputs, prohibiting the use of any product for weeding purposes, also of natural origin, and minimizing the use of organic fertilizers and pesticides, through measures to enhance soil life and its natural fertility (i.e., nourishing plants primarily through the soil ecosystem) and to prevent damage by pests and weeds, choosing appropriate resistant genotypes and crop rotation, and mechanical or physical methods. Therefore, the principles and approaches underlying organic agriculture are in line with the agroecological view of farming systems, although agroecology involves a wider approach, not limited to agronomic aspects, that overcomes any labels and certification systems. Agroecology aims not only to realize low-input farming systems, based on the exploitation of natural processes, but it also focuses on social–economical aspects, such as those related to human value, knowledge sharing, and equality in power distribution among the actors of the food supply chain. It is also true that, besides their principles, the regulations for organic agriculture allow the use of external products (EC Regulation 889/2008 [ 27 ]), which should be useful during the first period of transition to achieve a balance within the agroecosystem. However, in the real life of farms, this is often interpreted in a misleading way, and organic farming could follow the “input substitution approach” by replacing inputs permitted in conventional farming with others permitted in organic farming, which are not always very eco-friendly [ 51 , 60 , 61 ], without changing the underlying crop management approach.
Nevertheless, in our case study, organic agriculture is the basis upon which agroecological systems are generated. The organic rice farmers involved in the network are also agroecological farmers. They follow agroecological principles in relation to both (i) agronomic aspects (i.e., soil fertilization based on leguminous species and crop rotation, plant protection based on resistant genotypes, and the management of field flooding, innovative strategies for weeding without herbicides, as explained in [ 69 ]) and (ii) social aspects (i.e., group experience of knowledge sharing and mutual learning).
With the elimination of chemicals, the production of rice must be pursued through a complex process of varieties selection, crop rotation, and agronomic techniques to enhance soil and water resources and control weeds and pathogens, while also respecting the specificities of the territory. This work requires sophisticated know-how, experience, and skills that the Italian rice growers have long lost because they have been completely dependent on technology suppliers. The traditional research and advisory system is committed to ecological intensification but, due to the lack of specific funding dedicated to organic production, it has carried out few experiments on organic rice farming, mainly at the research station level [ 85 ]. The high costs of the innovative technologies developed, (e.g., mulch films and transplanting techniques, and the extreme variability of cropping systems)—depending on pedo-climatic conditions, field characteristics, and the business organization of farms—have prevented the dissemination of innovations beyond few farms. The spread of organic methods has taken place rather slowly, and organic rice production has remained niche, pursued only by a handful of pioneer farmers who, in the absence of prior knowledge, test innovative practices with a self-help and trial-and-error approach, as in Padel [ 71 ]. Organic rice cultivation was first adopted by farmers whose approach was seen as an “alternative” by the local agricultural community, i.e., biodynamic, macrobiotic, radical farmers motivated by strong environmental commitment, especially women. These farmers were initially treated with skepticism by their colleagues (as reported by [ 69 ]), sometimes even with suspicion and derision. However, their innovations were then taken up by a few pioneer farmers whose opinions are influential within the rice community, so that skepticism has now decreased, but it has not disappeared completely. This information derives from personal experiences reported by the farmers of the network. A female farmer of the network, for instance, explains that: “When the locals saw me do this work [Authors’ Note: manual work to avoid the use of herbicides], under the sun, with mosquitos all around… they thought me odd, they said: ‘that one has no brain’. That was another problem I had to deal with, being seen as a bit of an outsider. (…) It was very difficult. I struggled for many years. (…) I was heavily criticized because they saw that my business was earning much less than conventional farms—at the time, conventional farms were making good money—but I didn’t want to maximize profit, I wanted to maximize my personal expectations...”
In this context of difficult transition to organic farming, the multi-actor agroecological network analyzed here is carrying out participatory research and innovation to enhance organic methods. Exploring the values, motivations, processes, and relations of this Italian agroecological rice network is useful to understand if and how experiences of participatory research can change the trajectory of development in areas of intensive agriculture.
Our research explored the role and mechanisms of a participatory research network for the conversion to organic agriculture. We identified the following research goals:
To investigate learning processes and enabling environments;
To identify limits and opportunities of participatory research networks.
The questions that guided this study include:
Why did the farmers, researchers, and other actors join the participatory research network?
What and how do they learn within the participatory network?
Which are the limits and opportunities for the future of the network?
Methodology
This article draws on fieldwork investigating the partnership created by a group of farmers, scientists, government officials, and business managers in Northern Italy, between Lombardy and Piedmont, to research agroecological rice farming.
We integrate case study research and grounded theory, as in Andrade [ 4 ], choosing an interpretive approach [ 33 , 42 , 79 , 90 ]. We use qualitative methods, such as in-depth interviews and participant observations, constantly acknowledging the pedagogical model provided by Tracy [ 92 ] for quality issues. Twenty in-depth interviews were conducted, from January to November 2018, with the members of the network, using a biographical approach [ 66 , 89 ]. The interviews started by asking the respondents to tell their stories. They were invited to reflect on the origin and evolution of their professional experience, the moments of change and the time when they joined the network. They were also asked to say why they decided to participate in the network and to evaluate the consequences on their work and their expectations for the future. Spontaneous discussion, listening, and empathy were privileged throughout the process. The interviews were noted down, audio and video recorded with the interviewees’ permission, and later transcribed.
The functioning of the network and the relations among its members were directly observed during the partnership’s meetings, from September 2017 to December 2018. It was also possible to be involved in the informal exchange of messages among the participants via social networks and email.
Midgley [ 59 ] says that supporting evidence is often based on single case studies of intervention, and Meyer [ 54 ] points out the need to consider what is unique in each intervention. Our case study describes a small network of 28 people featuring farmers, researchers, and other actors. Other European networks have the same small number of participants, around thirty [ 40 ]. Therefore, the number of in-depth interviews (20), covering 70% of the network participants and integrated with the results of the observations made directly by the researchers during the network meetings over 16 months, appears reasonable and justifiable.
Objectives, methodologies, results, drivers of change, values, and visions were analyzed using grounded theory to develop an understanding of the processes of participation, assumption of responsibility, learning, and innovation. Grounded theory, in its latest evolution (e.g., [ 19 , 21 ]), is an interpretive method used to systematically analyze texts, such as interview transcripts and observation notes, in order to build theory concepts. This is done by reading the texts with specific questions in mind, extracting themes, and coding passages with keywords and quotes.
The narrative approach is used extensively in participatory social science, i.e., education, psychology, youth and childhood studies, geography, and land management science (for example, [ 86 ]). We found few applications in rural studies. In Phillips and Dickie [ 74 ], the narrative approach has been adopted to explore the rural future associated with climate change. Boxelaar et al. [ 10 ] explored how narrative approach can facilitate change in land management, demonstrating that this approach highlighted some of the ambiguities that existed within the project, but it did very little to change the course of the project. Kajamaa [ 47 ] shows that the narrative approach is appropriate to enrich participatory research when used in a complementary way to other ethnographic methods, such as in our case.
With the aim to explore which elements of the participatory research network support the transition to organic farming, the material was organized to fit into these categories:
Objectives, structure and functioning of the network;
Processes in the network;
Values shared;
Relations, power, and inclusion.
Results and discussion
The riso bio vero network.
The Riso Bio Vero (RBV) network brings together several organic rice farmers from the heart of the Italian rice district (provinces of Pavia, Vercelli, and Novara), as well as from outside this area. Scientists, public officials, and the managers of a company distributing organic products have also joined the network. The agricultural component of the group is not very representative of Italian farmers. According to the latest census of agriculture [ 46 ], in Italy, 30.7% of farmers are women, 2.5% are under 40, 6.2% are graduates, and only 0.8% have a degree in agriculture. In Europe [ 30 ], the first three figures are respectively: 28%, 11%, and 7.5%. In the RBV network, instead, women, young people, and graduates are well represented (respectively, 7 out of 17, 3 out of 17, and all) (Table 1 ).
The most recent data on the structure of European agriculture [ 30 ] suggest that, on average, 28% of farms across the EU are managed by women, with considerable differences among countries. In Lithuania and Latvia, nearly half of all the farms are managed by women; by contrast, in Finland, Malta, Germany, Denmark, and the Netherlands, the proportion of female farm managers does not exceed 10%. Many studies demonstrate that participatory and agroecological approaches can be gender-sensitive, i.e., able to address the issues of gender inequality and inclusion (see for example, [ 39 , 67 ]).
Only 11% of all farm holdings in the European Union (EU) are run by farmers under 40 (6.9% by farmers younger than 35 and just 4.9% by women under the age of 35) [ 30 ], and persuading more young people to begin farming is a major challenge [ 5 ]. The EU is encouraging young people to take up farming with start-up grants, income support, and benefits, such as additional training ( Ibidem ). Flament and Macias [ 32 ] highlight that a growing number of urban youths, often with a university degree, are deciding to become farmers. Described as “new peasants”, many of them choose agroecology as an alternative way to enter the food system, promoting both social and environmental sustainability. The idea of young farmers being “innovative” and turning away from traditionally intensive industrial farming models was already promoted by de Rooij in 2004 [ 23 ].
On average, only 7.5% of the current generation of European farmers have received full agricultural training, and 73.5% only have practical agricultural skills, coming from professional experience. Among farm managers, educational attainment is lower among women than men (5% versus 10% for full agricultural training and 79% versus 68% for only practical training) [ 30 ].
The RBV network was established in 2016 thanks to the coming together of a group of people who, despite knowing one another, until then had only occasionally collaborated. The intensification of their relations was linked to the opportunity, offered by the University of Milan, to organize the second international conference on Organic Rice Production (ORP 2) in Milan, on the occasion of EXPO 2015, the Universal Exposition hosted by Italy and focusing on food and agriculture. The conference was very successful; teamwork was stimulating; and the goal of continuing to work more steadily together was reinforced. The people who took part in the organization of the conference felt that they had a common vision of their work and that together they could defend and enhance their activities, even against the harsh attacks suffered by the sector. At the end of 2014, in fact, a television reportage ( Report on the national TV channel Rai3) had revealed the phenomenon of “falsi bio” (false organic producers), triggering a crisis that affected the entire rice industry, both organic and conventional, and still persists. Attacks on the image of organic rice farming played a crucial role in the decision to establish the group called “Riso Bio Vero” (True Organic Rice) to affirm the integrity of a portion of organic rice growers and their firm condemnation of fake organic producers.
A young farmer of the network explains the “false bio” phenomenon in Italy by saying:
“We are 100% organic, which is a very important choice to give the business credibility. In 2014, I was among those who fought the hardest against the issue of fake organic rice. When I started the conversion, I saw that some of my competitors basically produced in the traditional way, but then all their papers were in order to obtain the certification. This is damaging to honest organic producers, consumers, as well as to conventional producers, who choose to follow the rules and don’t give in to the golden opportunity of making easy money. Unfair competition swallows up other businesses. Both conventional and organic farmers are wiped out by those who do not comply with the rules. In 2014, together with other farmers, I decided to expose this unacceptable situation. We did it, for example, by collaborating with Report (there were many other initiatives, but Report achieved the greatest visibility). We were involved in writing the episode of the program about this issue, which became a sort of turning point in Italy’s organic rice production and, to an extent, in the organic production of other sectors too. Before that, there were thousands of hectares of organic rice cultivation that were actually farmed in the conventional way. There was no crop rotation, the embankments had no vegetation—and I have never seen land remaining bare without undergoing treatment. Since Report , the history of organic farming has changed. From then on, there has been much more attention from the institutions, from politics, born of our denunciation, of our raising awareness and rebelling, of our will to redeem the sector, especially on the part of young farmers who can’t tolerate living in such a… how can I put this… such an unfair world.”
The group’s original core included ten organic rice farmers (four from Lombardy, five from Piedmont and one from Tuscany), a professor from the University of Milan, an official from the Lombardy Region, and a representative of a company distributing organic products. Afterwards, a retired official of the Piedmont Region and a professor from the University of Pavia also joined. Both academics made available to the network their research groups, made up of technicians and young researchers.
Thanks to the participation of the University of Milan in the Riso-Biosystems national project (2017-2019), two scientists from two different public research institutions joined the network too. Furthermore, the research activity became a specific work package of the project. Although it would be very interesting to analyze the relationships between the RBV network and the rest of the partnership and the level of integration achieved, such a topic is not the subject of this study.
Subsequently, some organic rice growers became members of the network either permanently (two farmers from Piedmont) or occasionally (farmers from Veneto).
The group was founded with the aim to demonstrate that organic rice can be produced in a serious way, without circumventing the limits imposed by European regulations, which forbid the use of chemicals. The group of pioneer farmers have come together to promote their common interests, i.e., demonstrating the methods and best practices at the basis of professional organic rice production. They are all officially certified organic farmers. However, their views go beyond any labels, because they believe in the agroecological approach, which regards the farm as a living system that interacts with the environment and the socio-political structure of the territory. For these reasons, they do not consider organic farming a mere sustainable alternative to conventional farming and aim to avoid products that are permitted by organic farming regulations but not environmentally friendly. They have also focused on exposing the strategies of fake organic rice producers, which circumvent the limits imposed by the European regulations forbidding the use of chemicals. Indeed, the rice sector is particular prone to fraud since, differently from other productions, organic and conventional farms share the same irrigation system, based on a network of watercourses and channels that cross the valley of the river Po. Therefore, the auditing authorities cannot deem traces of banned chemicals in rice plants to be objective proof of forbidden treatments, since it is impossible to exclude accidental contamination through the sharing of irrigation water. Furthermore, the lack of chemical residues on the rice grain, despite repeated spraying of the plant, which is a good point for consumers, prevents the distinction between the production obtained with the organic method and that obtained with the conventional method, making organic cultivation susceptible to fraud.
Around this goal, the group began to collaborate by sharing previous knowledge and experiences. The partnership gathered latent discontent toward conventional rice cultivation and bitter frustration toward false organic farmers, channeling them into a participatory research system that would allow experimentation and innovation in agroecological rice cultivation.
Network’s role, activities, and structure
Participation in the group allows its members to share know-how and improve individual techniques, quickly adopting and adapting innovations successfully tested by others and, above all, starting a new research process “from below”. The exchange of individual experiences is very important for the site specificity of organic practices. Due to extreme variability in microclimate and soil conditions, as well as in farmers’ resources, capacities and organization, a good technique for one farm may not be feasible or suitable for another. Testing different techniques at the same time within a single context, as seen in parcel experimentation both at the farm and research station level, does not provide useful results in organic farming [ 8 , 48 , 88 ]. Vice versa, the application of the same technique to many different farms allows the growers to produce new insights and learn from one another. The first approach assumes a certain level of uniformity of cropping conditions across different farms. It transfers the results obtained from experimental trials, implying convergence of innovation through a standardized pattern of techniques, valid for different places and different times (the “funnel” scheme). Unfortunately, organic fields are unpredictably diverse, due to the reduction of external inputs that minimize possible sources of variability. Farmer-led trials reveal the constraints and benefits of different techniques by applying them to a wide range of field conditions and farm contexts and then selecting and adapting those that best respond to the specific characteristics of each farm (“folding fan” scheme). Bell and Bellon [ 6 ] explain the difference between the two approaches in terms of universalization versus generalization. The active involvement of the farmers in the research process makes it possible to experiment and adapt the same techniques to different farms, to achieve the quick and efficient generalization of best practices. Because of the extreme variability of environmental conditions among organic farms, even those where the same species are grown, the rapid dissemination of innovative results would not be feasible if the farmers were not involved—that is, if it were not supported by those who spend most of their time in the fields, carefully observing nature and its interactions with their own work, supervising the experiments and verifying their results year after year.
“Results come from individual experience, but experience comes from the exchanges among the farmers, who experiment with different techniques, each on their own land, each with their entrepreneurial approach. The mixing, discussion and reflection with the researchers and officials brings about improvements in the sector. Everyone has given and received much—this is the true strength of a network. We have become a network because we have done a lot of sharing, guided by mutual trust.” (Female farmer)
The activity of the network has allowed its members to improve existing agronomic techniques, increase and stabilize yields, and make actual discoveries, such as those regarding the allelopathic function of some species used as cover crop.
The research process is complemented by mutual assistance in the choice of machinery and suppliers, as well as in the management of the business, marketing strategies, information on regulations, and funding opportunities.
At first, discussion and collaboration among the members of the network concentrated on agronomic practices, the performance and constraints of little known agro-techniques, and issues of business administration and marketing. Then, the focus widened to include questions not strictly related to farming, e.g., measures to improve the transparency and integrity of the supply chain (critical issues and opportunities regarding both the improvement of the traditional organic certification system and alternative participative certification systems), practices to enhance plant biodiversity in the paddy fields, etc.
The governance of the network is very simple. A rice grower acts as leader of the farmer members, while a research fellow from the University of Milan serves as a bridge to the academic component and animates the entire network by taking care of overall communication. The group meets periodically, about once a month, preferably at the home of the farmers’ leader. The meetings last a whole day and include a shared lunch, for which everyone brings something that they have cooked. Regular attendance is supported by sharing meals and by common participation in other activities (e.g., training visits, trade fairs). The fact that all the participants invest a great deal of time in the network meetings and activities is not seen as a limit, but as a strength of the network.
The agenda of the meetings is set and shared by email. The researchers and farmers’ leader facilitate the discussion, which flows quite spontaneously, and use a projector to present data, results and videos, but no particular participatory method or approach is deliberately used. Sometimes, visits to one or more farms follow the discussion and help to verify the progress of the experiments undertaken directly in the field.
Outreach initiatives are carried out together with the research activity, including scientific publications authored by all the members of the network, seminars and conferences (i.e., ORP3 in Brazil in 2018 and a national conference open to all the actors of the supply chain, including the media, in Milan in 2019). The network is also preparing a manual for the identification of weeds in the paddy fields, a summary document on yields in organic rice cultivation and self-checking guidelines for the certification system.
Research process
The research process is managed through four cyclically repeating phases:
A phase of discussion concerning the issues detected in daily practice and possible experiments to investigate them.
A phase of experimentation conducted by the rice growers on their own farms but monitored by the farmers’ leader and the research fellow, who periodically visit the farmers and assist them with their technical needs, both directly in the fields and from a distance via social networks and email. At times, neighboring farmers also take part in the visits, to see the fields and give suggestions.
A collective phase of gathering, sharing, analyzing, and interpreting the results.
A phase of adoption of innovations at the farm level and identification of further critical issues.
Without knowing it, the growers are using the cycle learning process proposed by Kolb in his theory on experiential learning [ 49 ], in which concrete experience, reflective observation, abstract conceptualization, and active experimentation follow one another. Such an approach does not involve specific planning or the use of facilitating tools; rather, it centers around a reflexive, flexible, and iterative process. The action–reflection cycle helps establish a body of knowledge that is constructed and refined by the participants and represents a synthesis of the different skills brought to the partnership. A good example of this process is the research activity on plant biodiversity. During a conference, a farmer came into contact with some academics from the University of Pavia who were talking about a typical indigenous species found in the paddy fields ( Marsilea quadrifolia L.), which had been declared endangered due to massive herbicide use [ 11 ]. The farmer recognized the plant from having seen it in her fields and invited the incredulous scientists to visit her farm. The discovery triggered a research project, carried out on the land of all the farmers in the network and in the university lab, to verify the relationship between agronomic practices and plant biodiversity and enhance the ecological function of the paddy fields. It also offered the opportunity to design the brand “Marsilea rice”, to be used on the market to strengthen the identity of the group in opposition to false organic farmers. This example clearly shows how flexible the network is in its activities and scope, effectively combining a wide range of disciplines.
The members of the network are all at the same level and participate in the research and innovation process without a hierarchical approach. The academics provide their knowledge and stimulate the adoption of scientific procedures, but they are open to new forms of learning from cross-cultural exchange. They emphasize that their involvement in the network is driven by genuine interest in participatory research, curiosity about its functioning and fun and excitement in experimenting alternative forms of doing research. They admit that this research approach is not successful in terms of publications.
“Now I want to test this new approach, understand if it works, where it doesn’t work, why it works, with the clear and critical thinking of a researcher, without taking for granted that it will be a successful process. For instance, in terms of publications, it isn’t, but it is undoubtedly more interesting, fun, and exciting.” (Professor, male)
The scientists have backgrounds in agronomy, natural sciences, agricultural economy, and rural sociology, but they lack specific skills in participatory methodology. They share a commitment to participatory research that prioritizes respect, trust, and openness to experience, and their attitude is fundamental to ensure good relationships with the farmers and the other actors in the network. The researchers take the farmers’ skills very seriously to prioritize research aims and develop and validate agronomic practices. This trust is perceived by the farmers and reciprocated. Indeed, regular and direct contact between the researchers and the farmers allows them to strengthen the feeling of mutual trust that they have built.
The fact that a company distributing organic products has been present since the establishment of the network has meant that many of the farmers involved have signed a supply contract with this company. The agreement requires compliance with a set of strict cultivation guidelines deemed to be even more stringent than that required by the European organic certification regulations.
A female farmer explains: “It is an unbelievably strict contract. When you sign it, you accept being under constant monitoring, with two checks, one during the growth phase, when a rice sample is taken and analyzed, and another before the harvest—two multi-residual analyses—and then constant technical inspections. There is also a sort of protocol involving green manure or harrowing, so using cover crops or rotary tillers, but no support whatsoever.”
According to the producers, this seriousness is a guarantee for their image and is well remunerated by their buyers. So far, this economic relationship among many members of the network has not been considered an obstacle to the group’s research and innovation goals.
The network’s research activities have been funded through public and private tenders (e.g., bank foundations), and some members have supported them with their own funds. Although this is an example of bottom–up research funding, the extemporaneous and unorganized nature of such support prevents any assessment of this aspect.
Furthermore, the members have not yet taken into consideration issues of research ethics, such as confidentiality, property of innovations, and conflicts of interest.
Shared values
When the members of the network describe the values that they share, they mention a wide variety of topics, such as environmental commitment, responsible business ethics, economic rationality, aesthetics, and enhanced satisfaction in doing their job. Some common principles recur in the narratives collected through the interviews:
The members of the group are engaged in organic rice cultivation because they pursue not only economic profit, but also the protection of the environment in which they work and live, for themselves and for others.
“The radical decision of going organic, which I made a few years ago, was motivated, above all, by the situation of the market, which no longer offered any guarantee of profit or sustainability from any point of view. In my opinion, organic farming went instead in the direction of sustainability and business growth oriented toward the future. It means meeting the needs of aware consumers, producing a series of positive externalities besides the mere production of foodstuff. To me, being an organic rice producer today means being a business that yields a better type of food in many regards, provides a healthy environment, and is attentive to resources, which are not my private resources but common goods for the whole community, such as water and soil. Making this choice provides positive answers to all of these issues. This is what doing organic farming means.” (Young male farmer)
They believe that farmers must take responsibility for the environmental impact of agriculture.
They honor this commitment with courage.
They include ethics among the most important values of their activity.
“Climate change has forced us to face our responsibilities. Science is not neutral; it is not aseptic. Passion, ethics, values, ideals, and vision must be part of research. In organic farming, this is a viable path. It is not just a utopia; it is technically feasible too.” (Professor, male)
They believe that in organic farming, they can express their creativity, professionalism, and values, which were frustrated in conventional agriculture.
“Doing organic farming means doing varied and creative work. This is what organic farming requires. The seasons change every year and there is no fixed date for sowing, no fixed protocol, it changes from land to land. So, you need a lot of focus and a creative mind. Agriculture of this sort relies on everyone’s collaboration, intelligence and creativity. And everyone is important.” (Female farmer)
“The biggest difference between conventional farmers and organic farmers is that organic farmers feel peace of mind, they know that they’re doing the right thing. This is the underlying reason, they know that they are working at their best, that their cultivation methods are superior in quality, without compromises, and that there is no one to tell them what they should do, to give them chemicals. They know that they are working healthy fields, not sick fields constantly in need of chemicals for this and that.” (Female farmer)
Basing their work largely on their own abilities and resources, they feel more responsible, autonomous and free of constraints than when they used conventional methods and were highly dependent on external inputs.
“I decided to work the land with my own hands because I have always liked nature. As a child, I went to the countryside and I spent entire days observing the colors, the light, the shapes of nature. Being able to do a job that would bring me back to a place that was natural to me was the right choice. Obviously, it is not all bucolic and effortless. You are faced with all the problems of a much more difficult type of agriculture that puts you in direct correlation with nature, makes you use your brain. No technician comes along to tell you what to do. There are no technicians in organic rice farming. It’s all up to us. So, this also makes you more perceptive.” (Female farmer)
They believe that organic farming is a means of reducing costs and earning the right income for a decent life. When they practiced conventional cropping, most of their revenues were used to pay consultants and suppliers, and the margins to live with dignity were limited.
“I hope I’ll have a proud future, not a meagre one, not only in economic terms, but also from the point of view of the dignity of my work, which has something to give to everyone. I want to keep doing this with my head held high and I want those who will come after me to be able to do the same, with the same pride, the same determination, the same will to do it well.” (Young male farmer)
They find satisfaction working in contact with the land and aesthetic pleasure in the observation of nature: They believe that organic farming is the only way to preserve the beauty of nature and live in harmony with it.
“This is the land I got from my ancestors, my father and grandfather. I am proud to own it and I have always felt the responsibility of owning this land. The choice of going organic developed over many years, out of the awareness that we belong to nature and, as nature’s children, we are called upon to practice farming that respects nature, that loves it.” (Female farmer)
Their mission is to prove that organic rice cultivation can be carried out seriously and transparently.
They express their opinions and values with a very high level of emotional engagement. “Years ago, if I had had to imagine what my future business and my profession would be like, I would have never imagined, never even dreamed, that I could reach such a high level of satisfaction, creation of common work, collaboration with other farmers, with universities, with the Ministry. Not in my wildest dreams. I am so very happy.” (Female farmer)
In the network, they have created a physical, epistemic, and emotional space in which they meet and engage in shared knowledge production, free of power relations.
A young male farmer says: “During our meetings, it happens that at the start I have an opinion and, by the end, I have changed my mind completely. For someone like me, that is pretty strange. It’s not easy for me to admit that my ideas were not so good after all. This is what happens in our group. The discussions and sharing all together, each with their own opinions, allow us to come up with new, better ideas. This is possible since all points of view are equally important and no one is judged because of what they say.”
A female farmer adds: “We didn’t keep anything to ourselves, if one of us found out how to do something, they would tell the others: look, this is how you can do it. (…) I don’t necessarily say the right things. Someone else might see things differently and have the right intuition for that situation. Then, when all’s said and done, I will also agree that what that person said was right…”
Speaking about the professor who is a member of the network, another female farmer says: “He was very smart, he said: I have nothing to teach you from a technical point of view. It is you who should teach me. You know all the methods. We got on well with him, because he’s an intelligent person, he gets things right away. That’s how this participated research came about. He had twelve serious businesses to collaborate with.”
In such a space, practices and emotions are both valued and legitimated. Many of the members of the group state that they have become friends and that this has allowed them to overcome the sense of loneliness widespread among organic rice farmers, which continues to be one of the main motivations for participating in the activities of the group.
A female farmer says: “We’ve also become friends, because we have met very often, we have shared many things. We spend whole days together, so we socialize, we share our problems, the nice moments, our emotions too, like the storks on the electricity poles, the frogs hopping all around, some strange bird we saw for the first time, the selfies… (…) In my opinion, this is another step in participatory research. It counts too. It has been a big help because we don’t feel lonely… Otherwise, you know, they tell you you’re odd, you’re a fool, why should you bother when you can just spray something, since no one checks anyway… so you start feeling isolated, very much so. I think it is greatly appreciated and it is the right way forward.”
Emotions emerge as an important factor in the innovative learning process of the network, as described in Lund and Chemi [ 53 ] and Bellocchi et al. [ 7 ].
The fact that agronomic science and agricultural practice are very close has fostered their mutual understanding. They speak a common language, but what has truly brought them together is the sharing of a common mission, vision, and responsibility.
The peculiarity of the RBV network is that it is made up of people that have different degrees of authority and knowledge, and yet come together. Power differences (which inevitably exist between farmers, government officials, academics, etc.) are overcome and, although the more charismatic people act as leaders, the network is not hierarchical, since each member has put a collective goal (i.e., the research objectives) before their professional aims (i.e., profit, publications, etc.). This entails more relaxed interactions, as the spirit of collaboration seems to reduce the dynamics of power normally expressed in a competitive environment.
A young female researcher says: “I used to work in another university and I was very frustrated. The way of doing research was oriented toward competition and I didn’t like that, but I saw no alternative. That was how the system worked and I was a newcomer, I counted for nothing. Then, one day, I was at a congress, sitting next to the professor who was my thesis advisor. A colleague from our group was presenting some results, which came largely from my field work. I had worked so hard for my PhD. And this colleague was showing an article, bearing the names of all the people in the workgroup, except mine. I looked again, I thought I had to be wrong. I turned toward my professor and he said: ‘See how nasty we can be?’. I wanted to cry. But that moment made me understand that I had to change. I came here and I started working on this project, together with the farmers. I might never have a university career, but this work gives me satisfaction. I spend time in the fields with the farmers and I learn a great deal from them. We have published in international journals and we have put the names of all the farmers involved, specifically to acknowledge their contribution.”
Future of the network
The network defines itself as open and inclusive, but it has not established rules for the admission of new members, and applications to join made by other producers are assessed very carefully by the member farmers. The key requirement is to adhere to the principles of seriousness that characterize the network and, until now, this has been assessed through direct knowledge of the rice growers and their fields. During the process of inclusion of new farmers, the importance of relationships based on trust means that applicants are accepted only if they are considered “true organic”, beyond any official certification.
The network also features some public officials belonging to the institutions tasked with shaping policies for the transformation of rural areas, but so far, no initiative has been launched to stimulate a formal dialogue with these institutions.
The farmers are very directly involved in the network, appreciate the research activity and equal relationship with the researchers, and intend to formalize it in the near future. For their part, the researchers find this kind of work promising and engaging. The environmental outcomes of supporting a group of pioneering farmers involved in the difficult conversion to organic production justify the commitment of public personnel (researchers and officials), at least for now. In the future, the role of both researchers and officials will need to be redefined to avoid criticism for supporting a private group. The scaling-up of the research focus from mainly agronomic interests to the pursuit of sustainable development goals may also eventually motivate public participation. A workshop to understand if and how to incorporate the Sustainable Development Goals of Agenda 2030 [ 93 ] into the network has been conducted, but it has not led to any concrete assumption of responsibility.
Conclusions
The RBV network is a group of diverse actors from the organic rice sector participating in collective, self-planned, and self-developed research. Farmers, scientists, extension agents, government officials, and business managers are co-learning and co-producing knowledge and innovation. This public–private partnership is a voluntary, multi-year relationship that addresses the needs of the organic rice farmers, as well as those of the territory and the community, i.e., environmental issues and integrity of the supply chain.
An effective process of scientific and local knowledge sharing is taking place within the network. Cooperation is based on mutual trust and a common concern, i.e., how to shift from high-input cropping to organic farming, with the ultimate goal of protecting the environment and human well-being. The members’ active participation is mainly due to the fact that the activities carried out originate from real needs and concrete research questions.
The network follows a loosely structured agenda that allows for the continuous inclusion of new matters related to organic rice farming. In contrast to traditional research projects, which are planned in advance and leave little room for changes in goals, activities and methods, the spontaneous nature of this group generates high variability in the issues addressed, constantly reorienting its approach toward the emerging research questions.
This is a self-building group, formed around existing social relations, but inclusive and flexible: the joining of new actors (i.e., additional farmers, researchers skilled in specific topics, supply chain operators, etc.) is actively pursued through dissemination activities.
The participants show a very high degree of commitment and responsibility. The most evident sign of this is the considerable amount of time dedicated to research, both on the farms and in the regular meetings. All the members of the network are equally involved in the process of (i) defining the research questions and the activities to answer such questions, (ii) managing the research activities and the network’s organization, (iii) finding the resources needed for the research, inside and outside the network, and (iv) interpreting and evaluating the results. Such engagement is what makes them responsible, which is further confirmed by their strong motivation to disseminate the research results among other stakeholders outside the network.
Their involvement in the research process is transformative for the participants, who clearly admit that, by joining the network, they have changed their practices but also their ideas and beliefs. Such learning can create further transformations both in the sector and in the territory. Thanks to their intense communication work, the project findings are shared with other farmers and stakeholders and the network’s perspectives are brought to the attention of the institutions tasked with decisions on the transformation of rural areas. It will be interesting to follow the evolution of this network, so as to understand if it will essentially remain a group of friends engaged in collaborative research activities or if it will be able to develop into a model of innovation for the sector and an interlocutor for public decision-makers. In order to become an actor in the scientific and political debate, the network will probably need a more organized structure and include other relevant stakeholders, such as consumers, rural dwellers, and environmental NGOs.
Home and Rump [ 40 ] analyze 17 European Learning and Innovation Networks in Sustainable Agriculture (LINSAs) as part of the EU transdisciplinary research project SOLINSA. LINSAs are defined as networks of producers, consumers, experts, NGOs, SMEs, local administrations, researchers, and/or extensionists who are mutually engaged in pursuing common goals for sustainable agriculture and rural development, cooperating, sharing resources, and co-producing new knowledge by creating the right conditions for communication. Our case fits this definition perfectly. Home and Rump ( Ibidem ) recognize a wide variety of network typologies: from local scale to national or transnational; from small, simple homogenous networks to large, complex and diverse networks with multiple actors and “networks of networks”; from incremental to radical innovation; from top–down to bottom-up origin; and with several action fields, including non-food oriented, food production oriented and consumer oriented. Their study shows that LINSAs may emerge from small groups of farmers or may be inspired by individuals; they may develop as the formalization of an existing diffuse network or grow through a progressive process of co-opting local groups. Their size can vary from small (about 30 members), as in our case study, to about 100,000 farmers and 2,500 facilitators. Compared with the case studies presented by the two authors, our network has the following key characteristics:
Trans-regional scale (several regions of northern Italy);
Small dimension and simple structure;
Heterogeneous participation in terms of gender and age, but more homogeneous participation in terms of experiences and values (e.g., all the members are oriented toward the production of organic rice) and categories involved (consumers and NGOs are not present);
Commitment to both radical innovations (transition from conventional to organic rice) and incremental innovations;
Spontaneous, bottom–up origin;
Various action fields, including food production oriented, non-food oriented (environmental impact) and consumer oriented;
Low degree of formality;
Loose network with closed boundaries (participation in the network is voluntary, but the inclusion of new members appears to be contingent on sharing the same values, i.e., conventional farmers not willing to change are not accepted).
Participatory network experiences, especially for organic production, can be improved by considering the results of our analysis. In particular, in line with evidence from other studies [ 34 ], the importance of a supporting environment that facilitates and coordinates the learning processes is confirmed. What our case study highlights is that this environment can also be hardly structured or formalized. Indeed, it appears that the informal nature of the network is one of the key factors in its success.
As in Mukute and Lotz-Sisitka [ 64 ], collective learning happens when a group of people with different experiences and perspectives work together on the same issues and seek to jointly develop new knowledge or tools to address problems. As in Benton and Craib [ 9 ], in the learning process there is an emancipatory intent that is committed to changing unsatisfactory and oppressive realities, such as the socioeconomic and ethical crisis in the rice sector that started in 2014.
As Von Münchhausen and Häring [ 95 ] conclude, farmer–university networks function effectively if all their participants are considered equal partners. The findings of our research confirm the results of Home and Rump [ 40 ] who analyzed 17 networks, concluding with the identification of common factors that contribute to successful collaboration. Among these is the need to identify and build a working relationship with key partners, based on mutual trust and commitment, to strike a balance between guidance and listening, interactions and freedom, and to pursue positive and critical reflection—a fragile equilibrium that is difficult and time consuming to establish.
As in Mendez et al. [ 58 ], mutual learning takes place thanks to reciprocated trust, commitment and responsibility by all actors. These processes are favored by shared values. As a professor in our network points out, “Science is not neutral; it is not aseptic. Passion, ethics, values, ideals, and vision must be part of research.”
Mutual understanding is fostered by the use of a common language, both technical and methodological. Although applied for the first time in the network, the participatory approach has been fully espoused by its members. Despite being no experts in participation techniques, the network members understand and approve the reasons for participation.
The farmers involved in the network are well educated, unlike most farmers, and this aspect may influence their ability to speak a common language, comprehended by both the researchers and the other farmers.
The conversion to organic is often seen as a matter of procedures codified by regulations for a given period of time. For farmers, however, as the case study shows, conversion does not restrict itself to these procedures, but entails transformations that transcend any legal period and definition and have to do with the learning process that occurs in the network.
Our study results contribute to the participatory research approach by showing that personal values and attitudes are crucial. These certainly originate in the professional and human paths of the people involved, but can be developed both in education and training courses and through coaching and tutoring initiatives by other farmers and researchers who have had similar positive experiences.
Agroecology is an alternative development model to the failure of the traditional top–down innovation approach. It is said to be a knowledge intensive—as opposed to input intensive—agricultural practice [ 3 , 24 ]. Agroecology is also defined as the integration of scientific disciplines, agricultural practices, and social movements [ 97 ]. Hence, it requires an interdisciplinary approach to knowledge and pluralism in the ways of knowing. Participatory research, that is a transdisciplinary process, can therefore be seen as the right approach for the transition to agroecology. However, participatory processes need skillful researchers and farmers who have the ability to implement them and are willing to engage in the collaboration themselves. If we look at the matter from a sectoral perspective, the development of human capital receives little attention in the CAP. As highlighted by several recent studies, reforms are needed in this respect. A key suggestion that can be drawn from our case study is that of investing in the development of human capital and in the education of farmers and researchers in an integrated and coordinated way, so that they can develop skills in both agroecology practice and participatory research, designing new curricula in technical schools and universities and promoting the exchange of experiences between networks. A strong push toward education in farming is needed. Initial training is of national competence and agricultural education systems vary widely throughout the EU. But better integration between school and academic education and lifelong training is planned for the future through the European Social Fund and the CAP’s second pillar on Rural Development [ 5 ]. The future of European Participatory Research Networks can benefit from this integration. At the same time, bringing together complementary types of knowledge in a transdisciplinary approach, they can support that integration in innovative ways.
Availability of data and materials
The data supporting the findings of this study (audio and video recordings of the interviews; direct observation notes) are not publicly available, as they contain information that may compromise the privacy of those participating in the research, but are available from the corresponding author on reasonable request.
Altieri MA (1989) Agroecology: a new research and development paradigm for world agriculture. Agric Ecosystems Environ 27(1):37–46
Google Scholar
Altieri MA (2002) Agroecology: the science of natural resource management for poor farmers in marginal environments. Agric Ecosystems Environ 93(1):1–24
Altieri M, Nicholls CI (2012) Agroecology scaling up for food sovereignty and resiliency. In: Lichtfouse E (ed) Sustainable Agriculture Reviews, vol 11, pp 1–29
Andrade AD (2009) Interpretive research aiming at theory building: adopting and adapting the case study design. Qual Rep 14(1):42–60
Augère-Granier, M.L. 2017. Agricultural education and lifelong training in the EU. European Parliamentary Research Service [WWW] https://www.europarl.europa.eu/RegData/etudes/BRIE/2017/608788/EPRS_BRI (2017)608788_EN.pdf (visited on 02/06/2020).
Bell MM, Bellon S (2018) Generalization without universalization: towards an agroecology theory. Agroecol Sustainable Food Syst 42(6):605–611
Bellocchi A, Quigley C, Otrel-Cass K (2017) Exploring emotions, aesthetics and wellbeing in science education research. Springer Cultural Studies of Science Education 13
Bengtsson J, Ahnström J, Weibull AC (2005) The effects of organic agriculture on biodiversity and abundance: a meta-analysis. Journal of applied ecology 42(2):261–269
Benton T, Craib I (2001) Critical realism and the social sciences. In Benton, T. and Craib, I. (eds.) Philosophy of science. The Philosophical Foundations of Social Thought, Palgrave Macmillan, Basingstoke, pp 119–139
Boxelaar L, Paine M, Beilin R (2007) Change management and complexity: the case for narrative action research. The Journal of Agricultural Education and Extension 13(3):163–176
Bruni I, Gentili R, De Mattia F, Cortis P, Rossi G, Labra M (2013) A multi-level analysis to evaluate the extinction risk of and conservation strategy for the aquatic fern Marsilea quadrifolia L. in Europe. Aquatic botany 111:35–42
Buhler W, Morse S, Arthur E, Bolton S, Mann J (2002) Science, agriculture, and research: a compromised participation? Earthscan, London
Caister K, Green M, Worth S (2011) Learning how to be participatory: an emergent research agenda. Action Res 10(1):22–39
Caraveli H (2000) A comparative analysis on intensification and extensification in Mediterranean agriculture: dilemmas for LFAs policy. J Rural Stud 16(2):231–242
Carolan MS (2006) Sustainable agriculture, science and the co-production of ‘expert’ knowledge: the value of interactional expertise. Local Environment 11(4):421–431
Chambers R, Pacey A, Thrupp LA (eds) (1989) Farmer first: farmer innovation and agricultural research. Intermediate Technology Publications, London
Chambers R (1994) The origins and practice of participatory rural appraisal. World Development 22(7):953–969
Chambers R (1997) Whose reality counts? Putting the first last. ITDG Publishing, London
Charmaz K (2006) Constructing grounded theory: a practical guide through qualitative analysis. Sage, London
Cooke B, Kothari U (eds) (2001) Participation: the new tyranny? Zed Books, London
Corbin J, Strauss A (2015) Basics of qualitative research: techniques and procedures for developing grounded theory. Sage Publications, Thousand Oaks
Cuéllar-Padilla M, Calle-Collado A (2011) Can we find solutions with people? Participatory action research with small organic producers in Andalusia. Journal of Rural Studies 27:372–383
De Rooij, S. 2004. Young farmers in Europe: opting for innovation. LEISA INDIA Magazine on Low External Input Sustainable Agriculture 6(2):24-26.
De Schutter O (2010) Report submitted by the Special Rapporteur on the right to food, United Nations General Assembly, Human Rights Council, Sixteenth session, United Nations: New York
Edwards-Jones G (2001) Should we engage in farmer-participatory research in the UK? Outlook on Agric 30(2):129–136
EIP-AGRI, 2020. Research needs from practice 2020. EIP-AGRI [WWW] https://ec.europa.eu/eip/agriculture/sites/agri-eip/files/eip-agri_report_research_needs_from_practice_2020_en.pdf (visited on 02/06/2020).
European Commission. 2008. Commission Regulation (EC) No 889/2008 of 5 September 2008 laying down detailed rules for the implementation of Council Regulation (EC) No 834/2007 on organic production and labelling of organic products with regard to organic production, labelling and control [WWW] https://eur-lex.europa.eu/legal-content/EN/TXT/PDF/?uri=CELEX:32008R0889&from=EN (visited on 03/06/2020).
European Commission. 2012. Communication from the Commission to the European Parliament and the Council on the European Innovation Partnership ‘Agricultural Productivity and Sustainability’ Brussels: European Commission [WWW] ec.europa.eu/eip/agriculture/sites/agri-eip/files/communication_on_eip_-_en.pdf (visited on 12/06/2018).
European Union. 2018. Regulation (EU) 2018/848 of the European Parliament and of the Council of 30 May 2018 on organic production and labelling of organic products and repealing Council Regulation (EC) No 834/2007 [WWW] https://eur-lex.europa.eu/legal-content/EN/TXT/PDF/?uri=CELEX:32018R0848&from=EN (visited on 02/06/2020).
Eurostat. 2016. Agriculture, forestry and fishery statistics [WWW] https://ec.europa.eu/eurostat/documents/3217494/7777899/KS-FK-16-001-EN-N.pdf/cae3c56f-53e2-404a-9e9e-fb5f57ab49e3 (visited on 03/06/2020).
FAO (2016) FAOSTAT Food and agriculture data. FAO, Rome [WWW] www.fao.org/faostat (visited on 29/04/2018)
Flament SO, Macias B (2015) New peasants moving back to rural areas. Farming Matter 31(2):12–21
Flyvbjerg B (2006) Five misunderstandings about case-study research. Qual Inq 12(2):219–245
Fotheringham, J., Hetherington, A., Kobilsky, A., Rohmer, B., Chever, T., Renault, C., Romieu, V., Carillo, J., Giambenedetti, G., Vukovic, M., Collison, M., and Kuehnemund, M. 2016. Evaluation study of the implementation of the European Innovation Partnership for Agricultural Productivity and Sustainability. Final Report. European Commission: Brussels [WWW] https://op.europa.eu/en/publication-detail/-/publication/3f035a53-e9dc-11e6-ad7c-01aa75ed71a1 (visited on 02/06/2020).
Funtowicz S, Ravetz J (1993) Science for the post-normal age. Futures 25(7):739–755
Gabathuler E, Bachmann F, Kläy A (2011) Reshaping rural extension. In: Learning for sustainability (LforS) – an integrative and learning-based advisory approach for rural extension with small-scale farmers. Margraf Publishers GmbH, Weikersheim
Gliessman SR (1995) Sustainable agriculture: an agroecological perspective. Adv Plant Pathol 11:45–57
Gliessman SR (2008) Agroecology: ecological processes in sustainable agriculture. Ann Arbor Press, Chelsea
Gomez LF, Ríos-Osorio LA, Eschenhagen-Durán ML (2016) Key concepts of agroecology science. A systematic review. Trop Subtrop Agroecosystems 19:109–117
Home R, Rump N (2015) Evaluation of a multi-case participatory action research project: the case of SOLINSA. The Journal of Agricultural Education and Extension 21(1):73–89
Gomiero T, Pimental D, Paoletti MG (2011) Environmental impact of different agricultural management practices: conventional vs. organic agriculture. Crit Rev Plant Sci 30(1-2):95–124
Guba EG, Lincoln YS (1994) Competing paradigms in qualitative research. In: Denzin NK, Lincoln YS (eds) Handbook of qualitative research. Sage Publications, Thousand Oaks, pp 105–117
Guijt I, Shah MK (eds) (1998) The myth of community: gender issues in participatory development. Practical Action Publishing, England
Hickey S, Mohan G (2004) Participation - from tyranny to transformation. Zed Books, London
ISPRA (2018) Rapporto nazionale pesticidi nelle acque − dati 2015-2016. Edizione 2018. ISPRA, Roma
ISTAT. 2010. 6° Censimento generale dell’agricoltura. ISTAT, Roma [WWW] http://censimentoagricoltura.istat.it (visited on 29/04/2018).
Kajamaa A (2012) Enriching action research with the narrative approach and activity theory: analyzing the consequences of an intervention in a public sector hospital in Finland. Educational Action Research 20(1):75–93
Kravchenko AN, Snapp SS, Robertson GP (2017) Field-scale experiments reveal persistent yield gaps in low-input and organic cropping systems. Proceedings of the National Academy of Sciences 114(5):926–931
Kolb DA (1984) Experiential learning: experience as the source of learning and development. Prentice Hall, Englewood Cliffs
Lawrence DN, Christodoulou N, Whish J (2007) Designing better on-farm research in Australia using a participatory workshop process. Field Crops Res 104:157–164
Levidow L, Pimbert M, Vanloqueren G (2014) Agroecological research: conforming — or transforming the dominant agro-food regime? Agroecol Sustainable Food Syst 38(10):1127–1155
Lilja N, Bellon M (2008) Some common questions about participatory research: a review of the literature. Development in Practice 18(4–5):479–488
Lund B, Chemi T (eds) (2015) Dealing with emotions: a pedagogical challenge to innovative learning. Sense Publishers, Rotterdam
Meyer J (2000) Evaluating action research. Age Ageing 29(2):8–10
Mansuri G, Rao V (2013) Localizing development: Does Participation Work? The World Bank, Washington
Martin A, Sherington J (1997) Participatory research methods – implementation, effectiveness and institutional context. Agricultural Systems 55(2):195–216
Menconi ME, Grohmann D, Mancinelli C (2017) European farmers and participatory rural appraisal: a systematic literature review on experiences to optimize rural development. Land Use Policy 60:1–11
Mendez VE, Caswell M, Gliessman SR, Cohen R (2017) Integrating agroecology and participatory action research (PAR): lessons from Central America. Sustainability 9:705
Midgley G (2011) Theoretical pluralism in systemic action research. Systemic Practice and Action Research 24(1):1–15
Mier M, Cacho TG, Giraldo OF, Aldasoro M, Morales H, Ferguson BG, Rosset P, Khadse A, Campos C (2018) Bringing agroecology to scale: key drivers and emblematic cases. Agroecol Sustainable Food Syst 42(6):637–665
Migliorini P, Gkisakis V, Gonzalves V, Raigón MD, Bàrberi P (2018) Agroecology in Mediterranean Europe: genesis, state and perspectives. Sustainability 10(8):2724
Mipaaf (Ministero delle politiche agricole alimentari e forestali). 2016. Piano strategico nazionale per lo sviluppo del sistema biologico [WWW] https://www.politicheagricole.it/flex/cm/pages/ServeBLOB.php/L/IT/IDPagina/10014 (visited on 12/06/2018).
Mosse D (2004) Cultivating development: an ethnography of aid policy and practice. Pluto Press, London
Mukute M, Lotz-Sisitka H (2012) Working with cultural-historical activity theory and critical realism to investigate and expand farmer learning in Southern Africa. Mind, Culture, and Activity 19:342–367
Nabasa J, Rutwara G, Walker F, Were C (1995) Participatory rural appraisal: principles and practicalities. Natural Resources Institute, Chatam
Olagnero M (2005) Vite nel tempo. La ricerca biografica in sociologia. Carocci, Roma
Oliver B (2016) “The earth gives us so much”: agroecology and rural women's leadership in Uruguay. Cult Agric Food Environ 38(1):38–47
Organic Action Network Italia. 2017. Carta del biologico di Bergamo. Il modello biologico per una produzione agricola e un consumo sostenibili. Organic Action Network Italia [WWW] http://www.anabio.it/uploads/article/cartadelbiologicodibergamo-92d8dfefbd.pdf (visited on 29/04/2018).
Orlando F, Alali S, Vaglia V, Pagliarino E, Bacenetti J, Bocchi S (2020) Participatory approach for developing knowledge on organic rice farming: management strategies and productive performance. Agric Syst 178:102739
Ortolani L, Bocci R, Bàrberi P, Howlett S, Chable V (2017) Changes in knowledge management strategies can support emerging innovative actors in organic agriculture: the case of participatory plant breeding in Europe. Org Farming 3(1):20–33
Padel S (2001) Conversion to organic farming: a typical example of the diffusion of an innovation? Sociologia Ruralis 41(1):40–61
Patel R (2012) The long green revolution. J Peasant Stud 40(1):1–63
Pence RA, Grieshop JI (2001) Mapping the road for voluntary change: partnerships in agricultural extension. Agric Hum Values 18(2):209–217
Phillips M, Dickie J (2014) Narratives of transition/non-transition towards low carbon futures within English rural communities. J Rural Stud 34:79–95
Pimbert M (2009) Towards food sovereignty: reclaiming autonomous food systems. International Institute of Environment and Development, London
Pound B, Snapp S, McDougall C, Braun A (eds) (2003) Managing natural resources for sustainable livelihoods: uniting science and participation. Earthscan Publications, London
Pretty NJ (1995) Participatory learning for sustainable agriculture. World Development 23(8):1247–1263
Pretty J (2002) Agri-culture: reconnecting people, land and nature. Earthscan Publications, London
Ragin CC, Becker HS (eds) (1992) What is a case? Exploring the foundations of social inquiry. Cambridge University Press, Cambridge
Reganold JP, Wachter JM (2016) Organic agriculture in the twenty-first century. Nature Plants 2(2):15221
Röling N (1988) Extension science: information systems in agricultural development. Cambridge University Press, New York
Röling N, Engel P (1990) The development of the concept of agricultural knowledge information systems (AKIS): implications for extension. In: Rivera WM, Gustafson DJ (eds) Agricultural extension: Worldwide institutional evolution and forces for challenge. Elsevier, Amsterdam
Röling N, Jiggins J (1998) The ecological knowledge system. In: Röling N, Wagemakers MAE (eds) Facilitating sustainable agriculture: Participatory learning and adaptive management in times of environmental uncertainty. Cambridge University Press, Cambridge
Röling N, Wagemakers MAE (eds) (1998) Facilitating sustainable agriculture: participatory learning and adaptive management in times of environmental uncertainty. Cambridge University Press, Cambridge
Romani M, Beltarre G, Tabacchi M (2007) Organic rice farming. Regione Lombardia, Milan
Savin-Baden M, Van Niekerk L (2007) Narrative inquiry: theory and practice. J Geogr High Educ 31(3):459–472
Sevilla-Guzmán E, Woodgate G (1997) Sustainable rural development: from industrial agriculture to agroecology. In: Redclift M, Woodgate G (eds) The international handbook of environmental sociology. Edward Elgar, Cheltenham
Shennan C, Krupnik TJ, Baird G, Cohen H, Forbush K, Lovell RJ, Olimpi EM (2017) Organic and conventional agriculture: a useful framing? Annual Review of Environment and Resources 42:317–346
Siciliano E (1998) Approccio biografico. In: Melucci A (ed) Verso una sociologia riflessiva. Il Mulino, Bologna
Stake RE (1995) The art of case study research. Sage Publications, Thousand Oaks
Tilman D, Cassman KG, Matson PA, Naylor R, Polasky S (2002) Agricultural sustainability and intensive production practices. Nature 418:671–677
Tracy SJ (2010) Qualitative quality: eight “big-tent” criteria for excellent qualitative research. Qualitative Inquiry 16(10):837–851
United Nations. 2015. Transforming our world: the 2030 agenda for sustainable development [WWW] https://sustainabledevelopment.un.org/content/documents/21252030%20Agenda%20for%20Sustainable%20Development%20web.pdf (visited on 5/4/2018).
Uphoff N (ed) (2002) Agroecological innovations: increasing food production with participatory development. Earthscan Publications, London
Von Münchhausen S, Häring AM (2012) Lifelong learning for farmers: enhancing competitiveness, knowledge transfer and innovation in the eastern German state of Brandenburg. Stud Agric Econ 114:86–92
Warner KD (2008) Agroecology as participatory science emerging alternatives to technology transfer extension practice. Sci Technol Hum Val 33(6):754–777
Wezel A, Bellon S, Dore T, Francis C, Vallod D, David C (2009) Agroecology as a science, a movement and a practice. A review. Agronomy Sustainable Dev 29:503–515
Wezel A, Goette J, Lagneaux E, Passuello G, Reisman E, Rodier C, Turpin G (2018) Agroecology in Europe: research, education, collective action networks, and alternative food systems. Sustainability 10:1214
Zuber-Skerrit O (2001) Action learning and action research: paradigm, praxis and programs. In: Sankara S, Dick B, Passfield R (eds) Effective Change Management through Action Research and Action Learning: Concepts, Perspectives. Processes and Applications. Southern Cross University Press, Lismore, pp 1–20
Download references
Acknowledgements
The authors acknowledge with gratitude the active involvement of the Riso Bio Vero network members and their willingness to tell their stories and share their thoughts. The interpretations in this article remain the authors’ own.
This study was carried out as part of the Riso-Biosystems three-year project (2017-2019), funded by the Italian Ministry of Agriculture, Food and Forestry Policies to study and promote organic rice. The funding body does not have any role in the design of the study, in the collection, analysis, and interpretation of the data and in the writing of the manuscript.
Author information
Authors and affiliations.
Research Institute on Sustainable Economic Growth (Cnr-Ircres), Italian National Research Council, Moncalieri (Turin), Italy
Elena Pagliarino & Secondo Rolfo
Department of Environmental Science and Policy, University of Milan, Milan, Italy
Francesca Orlando, Valentina Vaglia & Stefano Bocchi
You can also search for this author in PubMed Google Scholar
Contributions
All authors contributed to the conception and design of the work; EP and FO devoted themselves to the acquisition, analysis, and interpretation of data; EP have drafted the work and all authors substantively revised it. The authors read and approved the final manuscript.
Corresponding author
Correspondence to Elena Pagliarino .
Ethics declarations
Ethics approval and consent to participate.
This study was conducted in compliance with the CNR Code of Conduct of 10/20/2017, whose observance is supervised by the Institute Director, and in compliance with the University of Milan’s Code of Ethics and for Research Integrity, issued by Rectoral Decree 224/2019 of 18/01/2019.
Consent for publication
Not applicable.
Competing interests
The authors declare that they have no competing interests.
Additional information
Publisher’s note.
Springer Nature remains neutral with regard to jurisdictional claims in published maps and institutional affiliations.
Rights and permissions
Open Access This article is licensed under a Creative Commons Attribution 4.0 International License, which permits use, sharing, adaptation, distribution and reproduction in any medium or format, as long as you give appropriate credit to the original author(s) and the source, provide a link to the Creative Commons licence, and indicate if changes were made. The images or other third party material in this article are included in the article's Creative Commons licence, unless indicated otherwise in a credit line to the material. If material is not included in the article's Creative Commons licence and your intended use is not permitted by statutory regulation or exceeds the permitted use, you will need to obtain permission directly from the copyright holder. To view a copy of this licence, visit http://creativecommons.org/licenses/by/4.0/ .
Reprints and permissions
About this article
Cite this article.
Pagliarino, E., Orlando, F., Vaglia, V. et al. Participatory research for sustainable agriculture: the case of the Italian agroecological rice network. Eur J Futures Res 8 , 7 (2020). https://doi.org/10.1186/s40309-020-00166-9
Download citation
Received : 09 October 2019
Accepted : 03 August 2020
Published : 19 August 2020
DOI : https://doi.org/10.1186/s40309-020-00166-9
Share this article
Anyone you share the following link with will be able to read this content:
Sorry, a shareable link is not currently available for this article.
Provided by the Springer Nature SharedIt content-sharing initiative
- Rice farming
- Agroecology
- Participatory research
Information
- Author Services
Initiatives
You are accessing a machine-readable page. In order to be human-readable, please install an RSS reader.
All articles published by MDPI are made immediately available worldwide under an open access license. No special permission is required to reuse all or part of the article published by MDPI, including figures and tables. For articles published under an open access Creative Common CC BY license, any part of the article may be reused without permission provided that the original article is clearly cited. For more information, please refer to https://www.mdpi.com/openaccess .
Feature papers represent the most advanced research with significant potential for high impact in the field. A Feature Paper should be a substantial original Article that involves several techniques or approaches, provides an outlook for future research directions and describes possible research applications.
Feature papers are submitted upon individual invitation or recommendation by the scientific editors and must receive positive feedback from the reviewers.
Editor’s Choice articles are based on recommendations by the scientific editors of MDPI journals from around the world. Editors select a small number of articles recently published in the journal that they believe will be particularly interesting to readers, or important in the respective research area. The aim is to provide a snapshot of some of the most exciting work published in the various research areas of the journal.
Original Submission Date Received: .
- Active Journals
- Find a Journal
- Proceedings Series
- For Authors
- For Reviewers
- For Editors
- For Librarians
- For Publishers
- For Societies
- For Conference Organizers
- Open Access Policy
- Institutional Open Access Program
- Special Issues Guidelines
- Editorial Process
- Research and Publication Ethics
- Article Processing Charges
- Testimonials
- Preprints.org
- SciProfiles
- Encyclopedia
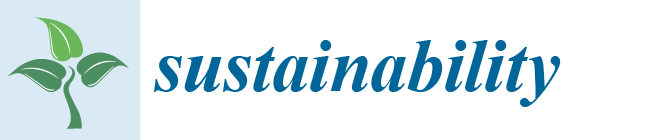
Journal Menu
- Sustainability Home
- Aims & Scope
Editorial Board
- Reviewer Board
Topical Advisory Panel
- Instructions for Authors
Special Issues
- Sections & Collections
- Article Processing Charge
- Indexing & Archiving
- Editor’s Choice Articles
- Most Cited & Viewed
- Journal Statistics
- Journal History
- Journal Awards
- Society Collaborations
- Conferences
- Editorial Office
Journal Browser
- arrow_forward_ios Forthcoming issue arrow_forward_ios Current issue
- Vol. 16 (2024)
- Vol. 15 (2023)
- Vol. 14 (2022)
- Vol. 13 (2021)
- Vol. 12 (2020)
- Vol. 11 (2019)
- Vol. 10 (2018)
- Vol. 9 (2017)
- Vol. 8 (2016)
- Vol. 7 (2015)
- Vol. 6 (2014)
- Vol. 5 (2013)
- Vol. 4 (2012)
- Vol. 3 (2011)
- Vol. 2 (2010)
- Vol. 1 (2009)
Find support for a specific problem in the support section of our website.
Please let us know what you think of our products and services.
Visit our dedicated information section to learn more about MDPI.
Sustainable Agriculture
A section of Sustainability (ISSN 2071-1050).
Section Information
The global population is set to increase to over 10 billion by 2050 and, with economic development, the average wealth of individuals in developing nations is set to rise—this brings with it a set of challenges for agriculture and the food supply chain. To date, the increase in the supply of food to meet global demand has been one of the great success stories of applied research. However, this success has come at the expense of nature; biodiversity is declining at an accelerating pace, rivers, lakes and aquifers are polluted, soils are polluted or being eroded, forests are being converted to grow crops to supply food and energy and greenhouse gas emissions from agriculture nearly equal those from transport. Things cannot continue as they are; future food security will need to focus on the sustainable supply of food, incorporating innovation in technology, supply chains and social science/economics. Nature can, and does, provide ecosystem services to agriculture and these services need to be incorporated into the management and conservation of biodiversity.
This section will offer findings that address the challenge of feeding the global population whilst protecting the planet. Manuscripts describing new research, new syntheses and new theories are welcome.
Following special issues within this section are currently open for submissions:
- The Sustainability of Agricultural Soils (Deadline: 30 June 2024 )
- Sustainability in Agricultural Economics and Agri-Food Management (Deadline: 5 July 2024 )
- Soil Improvement and Crop Productivity Increase in Sustainable Agriculture: Mechanism and Technology (Deadline: 5 July 2024 )
- Biodiversity Indicators for Ecological and Sustainable Conservation in Forests (Deadline: 9 July 2024 )
- Innovations in Agricultural and Rural Development in a Changing World (Deadline: 10 July 2024 )
- Production of Solid Biofuels from Agricultural Waste (Deadline: 20 July 2024 )
- Waste Utilization in Agriculture and Sustainable Development (Second Edition) (Deadline: 26 July 2024 )
- Sustainable Agriculture and Food Security (Deadline: 31 July 2024 )
- Advances in Sustainable Agricultural Crop Production (Deadline: 31 July 2024 )
- Sustainable Crop Productivity under Climate Change: Resilience, Nutritional Quality and Implications for Future Management (Deadline: 31 August 2024 )
- Sustainable Agricultural Development Economics and Policy 2nd Edition (Deadline: 31 August 2024 )
- Plant Biology and Ecophysiology for the Environment and Sustainability (Deadline: 31 August 2024 )
- Food Safety, Food Quality, Food Packaging and Sustainable Food Supply Chains (Deadline: 2 September 2024 )
- Biotechnology on Sustainable Agriculture (Deadline: 4 September 2024 )
- Weed Management in Sustainable Crop Production Management Systems (Deadline: 17 September 2024 )
- Planning and Sustainable Management of Irrigation in Agricultural Operations (Deadline: 26 September 2024 )
- Climate Change and Sustainable Food Production (Deadline: 27 September 2024 )
- Advancing Ruminant Nutrition for Sustainable Agriculture: Current Challenges and Future Perspectives (Deadline: 30 September 2024 )
- Innovative Technologies and Strategies for Enhancing Food Safety, Nutrition, and Sustainability (Deadline: 30 September 2024 )
- Agricultural Economic Transformation and Sustainable Development (Deadline: 30 September 2024 )
- Climate Change Adaptation and Mitigation—Organic Farming Systems (Deadline: 30 September 2024 )
- Feed Resources and Nutrition Strategies for Sustainable Livestock Production (Deadline: 30 September 2024 )
- Sustainable Agriculture and Food Systems in Southeast Asia and China (Deadline: 1 October 2024 )
- Sustainability of Agriculture: The Impact of Climate Change on Crops (Deadline: 2 October 2024 )
- Biocontrol for Sustainable Crop and Livestock Production, Volume II (Deadline: 10 October 2024 )
- Advanced Research on Soil Pollution and Sustainable Agriculture (Deadline: 15 October 2024 )
- Controlled Environment Agriculture for Sustainable Farming (Deadline: 20 October 2024 )
- Sustainable Agroecosystem: Interactions between Plants and Microorganisms (Deadline: 25 October 2024 )
- Environmental Management and Sustainable Agriculture: Soil, Water and Crops (Deadline: 26 October 2024 )
- Sustainable and Innovative Agriculture in Issues of Energy Production and Food Safety (Deadline: 31 October 2024 )
- Contaminants in Irrigation Water Impacting the Safety of Fresh Produce (Deadline: 31 October 2024 )
- Towards Sustainable Agriculture: Pollution Prevention and Control Technologies (Deadline: 1 November 2024 )
- Sustainable Agriculture: Plant Physiology, Nutrition and Crop Production (Deadline: 6 November 2024 )
- Water–Fertilizer Utilization and Sustainable Agriculture (Deadline: 22 November 2024 )
- Application of Biotechnology in Sustainable Agriculture (Deadline: 24 November 2024 )
- Sustainable Precision Agriculture: Latest Advances and Prospects (Deadline: 30 November 2024 )
- Farmland Soil Pollution Control and Ecological Restoration (Deadline: 30 November 2024 )
- Sustainable Crop Production and Plant Science: Theories, Methods, Modeling and Applications (Deadline: 30 November 2024 )
- Sustainable Development of Intelligent Agriculture (Deadline: 30 November 2024 )
- Advanced Research on Agriculture and Food Systems Landscape towards Sustainability (Deadline: 30 November 2024 )
- Sustainable Crop Production and Agricultural Practices (Deadline: 30 November 2024 )
- Weeds Management in Sustainable Agriculture System (Deadline: 30 November 2024 )
- From the Field to the Factory: The Dynamics of Sustainable Urban Agriculture (Deadline: 1 December 2024 )
- Agricultural Engineering for Sustainable Production and Circular Economy (Deadline: 20 December 2024 )
- Adaptive Response and Mechanism of Crops to Abiotic Stresses—2nd Edition (Deadline: 30 December 2024 )
- Soil Fertility and Plant Nutrition for Sustainable Cropping Systems (Deadline: 31 December 2024 )
- Sustainable Agriculture Development: Challenges and Oppotunities (Deadline: 31 December 2024 )
- Mitigating Greenhouse Gas Emissions from Livestock for Sustainable Agriculture (Deadline: 31 December 2024 )
- Sustainable Agricultural Engineering: Agroecology Sustainable Development and Agriculture Environmental Impact Assessment and Measurement (Deadline: 31 December 2024 )
- Interactions of Land Use, Economic Growth, and Greenhouse Gas Emissions in Sustainable Agriculture (Deadline: 31 December 2024 )
- Sustainability Assessment of Agricultural Cropping Systems (Deadline: 31 December 2024 )
- Agriculture, Land and Farm Management (Deadline: 31 December 2024 )
- Soil Heavy Metals Threaten Agricultural Sustainability: Translocation Processes and Remediation Strategies (Deadline: 31 December 2024 )
- Advances in Measurement, Instrument, and Sensing Methods for Sustainable Agriculture (Deadline: 31 December 2024 )
- Land Management and Sustainable Agricultural Production: 2nd Edition (Deadline: 31 December 2024 )
- Sustainable Agriculture and Restoration of Agroecosystem Affected by Climate Change (Deadline: 22 January 2025 )
- Sustainable Development of Agricultural Systems (Deadline: 27 January 2025 )
- Sustainable Agriculture and Biological Science (Deadline: 29 January 2025 )
- Oasis Agricultural Ecology and Sustainable Development and Utilization of Oasis Resources—2nd Edition (Deadline: 31 January 2025 )
- Green Microbiology: Advancing Sustainability through Microbial Innovations (Deadline: 31 January 2025 )
- Sustainable Agriculture: Cultivation and Breeding of Crops (Deadline: 5 March 2025 )
- Maple Syrup and Sugar Crops: Market and Climate Challenges and Potential Pathways for Sustainable Development (Deadline: 9 March 2025 )
- Environmental and Economic Sustainability in Agri-Food System (Deadline: 31 March 2025 )
- Advanced Agricultural Economy: Challenges and Opportunities (Deadline: 31 March 2025 )
- Sustainable Agriculture with Innovative Technology and Equipment: Towards a Low-Carbon Era (Deadline: 31 March 2025 )
- Sustainable Oasis Ecosystems and Saline–Alkali Land Improvement (Deadline: 18 April 2025 )
- Climate Change Impacts on Ecological Agriculture Sustainability (Deadline: 20 April 2025 )
- Climate Change and Sustainable Agricultural System (Deadline: 30 April 2025 )
- Sustainability of the Agricultural System and Agro-Ecological Environment (Deadline: 30 April 2025 )
- Effects of Soil and Water Conservation on Sustainable Agriculture (Deadline: 30 April 2025 )
- Biomass Energy Technologies and Sustainable Agricultural Waste Utilization (Deadline: 30 April 2025 )
- Sustainable Thermal Comfort: Heat Pump Solutions for Energy-Efficient Buildings (Deadline: 30 April 2025 )
- Soil, Plant and Human Health in Sustainable Environment (Deadline: 20 May 2025 )
- Sustainability Issues in Plant Growth and Development: Environmentally Friendly Alternatives for Pest Management (Deadline: 31 May 2025 )
- Sustainable Poultry Farming Practices: Innovations and Challenges (Deadline: 31 May 2025 )
- Exploring the Innovative Approaches and Advanced Practices to Sustainable Agriculture and Animal Husbandry (Deadline: 31 May 2025 )
- Harnessing Biological and Abiotic Solutions for Enhanced Productivity and Phytochemical Profile of Plants (Deadline: 25 June 2025 )
Topical Collections
Following topical collections within this section are currently open for submissions:
- Contemporary Challenges to the Sustainable Development of Agriculture and Rural Areas
- Policies for Sustainable Agricultural Productivity and Efficiency
- Rural Policy, Governance and Sustainable Rural Development
- Sustainability in Agricultural Systems and Ecosystem Services
- Sustainable Agriculture and Food Supply Chains in Changing Climate
- Sustainable Insect Farming: Feed the Future
- Sustainable Livestock Production and Management
Papers Published
Further information, mdpi initiatives, follow mdpi.
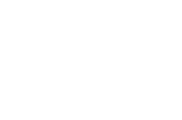
Subscribe to receive issue release notifications and newsletters from MDPI journals
Click through the PLOS taxonomy to find articles in your field.
For more information about PLOS Subject Areas, click here .
Loading metrics
Open Access
Peer-reviewed
Research Article
Artificial intelligence solutions enabling sustainable agriculture: A bibliometric analysis
Roles Conceptualization, Formal analysis, Investigation, Software, Visualization, Writing – original draft
Affiliation Institute of Agricultural and Food Economics, Hungarian University of Agriculture and Life Sciences, Gödöllő, Hungary

Roles Conceptualization, Formal analysis, Methodology, Resources, Software, Validation, Visualization, Writing – review & editing
* E-mail: [email protected]
Roles Data curation, Funding acquisition, Methodology, Project administration, Resources, Supervision, Validation, Visualization, Writing – review & editing
Affiliations Institute of Agricultural and Food Economics, Hungarian University of Agriculture and Life Sciences, Gödöllő, Hungary, North-West University, Vanderbijlpark, South Africa
- Priya Rani Bhagat,
- Farheen Naz,
- Robert Magda
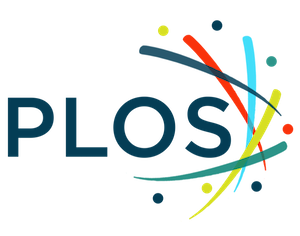
- Published: June 9, 2022
- https://doi.org/10.1371/journal.pone.0268989
- Reader Comments
There is a dearth of literature that provides a bibliometric analysis concerning the role of Artificial Intelligence (AI) in sustainable agriculture therefore this study attempts to fill this research gap and provides evidence from the studies conducted between 2000–2021 in this field of research. The study is a systematic bibliographic analysis of the 465 previous articles and reviews done between 2000–2021 in relation to the utilization of AI in sustainable methods of agriculture. The results of the study have been visualized and presented using the VOSviewer and Biblioshiny visualizer software. The results obtained post analysis indicate that, the amount of academic works published in the field of AI’s role in enabling sustainable agriculture increased significantly from 2018. Therefore, there is conclusive evidence that the growth trajectory shows a significant climb upwards. Geographically analysed, the country collaboration network highlights that most number of studies in the realm of this study originate from China, USA, India, Iran, France. The co-author network analysis results represent that there are multi-disciplinary collaborations and interactions between prominent authors from United States of America, China, United Kingdom and Germany. The final framework provided from this bibliometric study will help future researchers identify the key areas of interest in research of AI and sustainable agriculture and narrow down on the countries where prominent academic work is published to explore co-authorship opportunities.
Citation: Bhagat PR, Naz F, Magda R (2022) Artificial intelligence solutions enabling sustainable agriculture: A bibliometric analysis. PLoS ONE 17(6): e0268989. https://doi.org/10.1371/journal.pone.0268989
Editor: Ardashir Mohammadzadeh, University of Bonab, ISLAMIC REPUBLIC OF IRAN
Received: November 18, 2021; Accepted: April 29, 2022; Published: June 9, 2022
Copyright: © 2022 Bhagat et al. This is an open access article distributed under the terms of the Creative Commons Attribution License , which permits unrestricted use, distribution, and reproduction in any medium, provided the original author and source are credited.
Data Availability: The data for bibliometric analysis was extracted from SCOPUS database using relevant keywords provided within the study.
Funding: The author(s) received no specific funding for this work.
Competing interests: The authors have declared that no competing interests exist.
1. Introduction
As the world tries to battle through the novel challenges and obstacles caused by the Covid-19 pandemic and every sector faced detrimental impact of the pandemic at different stages of a business process. The agriculture sector is one of these sectors which faced several disruptions including labor supply for production, reduced transportation of agriculture produce, and risks associated with agriculture markets. Therefore, artificial intelligence (AI) systems and solutions will find contemporary applications in the field of agriculture and sustainable farming practices. Evidently, the pandemic is not a temporary disruption of normal but a milestone that will change the course of modern-history towards a sustainability driven society [ 1 , 2 ]. Sustainability is a multidimensional discipline that has been the popular research interest of scholars for the past few decades [ 3 , 4 ]. Because of its multidisciplinary attribute sustainability encompasses a wide variety of subjects ranging from climate, environment, green economy, food safety, sustainable agriculture, clean technologies etc. In a 2018 research article, Ávila et al. highlighted that in the field of education in sustainability, expansive research in every contributing domain is necessary to embark on the trail to global development [ 5 ].
The concept of “sustainable agriculture” has received increased attention in recent years with the rising technological improvements. In a 2020 keyword citation burst analysis on ecological modernization approach by Rocchi et al., “sustainable agriculture” consistently shows very high citation burst in the past and current literature [ 6 ]. Due to its high popularity among researchers the more recently published bibliometrics studies conduct in the realm of sustainable agriculture explore the interactions between sustainable agri-food systems with the economy, society, and policy making [ 7 ], agriculture systems modernization approach [ 6 ], big data in sustainable agriculture [ 8 ]. In terms of Artificial Intelligence in agriculture, recent articles provide bibliometric analysis of the crossover between remote sensing technologies and agriculture [ 9 ], advanced information and communication technology in agriculture [ 10 ], global trends in precision agriculture technology [ 11 ].
In the scope of this review, sustainable agriculture is defined as, the agricultural practices that ensure fulfillment of present day and future food and nutrition requirements of the society, while maximizing the net advantage towards the ecosystem, society, and earth when all its implication on costs and benefits are monitored [ 12 ]. Artificial intelligence (AI) was first coined by John McCarthy in 1956 and then many different definitions arise over the years but in the scope of this study, it is defined in the rational approach as a system that automates intelligent behaviour or acquires intelligence over time using computational programming and gives rational outputs to perform specific tasks without much human intervention [ 13 ].
The impact of the covid-19 pandemic on the agriculture sector is detrimental. There were various studies conducted in the past that observed the disruptions in the agriculture sector and provided sustainable solutions by employing AI technologies in creating sustainable agriculture. However, there is a dearth of studies that provided a state-of-the-art review of AI technologies application in sustainable agriculture. Therefore, this study was conducted to identify the current stage of knowledge concerning AI and sustainable agriculture and provided bibliometric and network analysis in this field. Also, this bibliometric research is motivated to bring together the highlights of the progress made in the application of AI in sustainable agriculture practices to inspire future applications of AI in this field. Hence, it is imperative for future researchers to reflect upon the past decade for academic literature in the field to be able to innovate according to the demands of the current pandemic situation [ 2 ]. This study attempts to compile, analyze, and identify the properties of the scholarly articles and reviews indexed in the Scopus database specifically for the keywords- artificial intelligence, Sustainable agriculture made available between the duration of 2000 to 2021. This study involves both quantifying and bringing out qualitative inferences from the size and features of previously published scholarly works from one or more source databases such as Scopus indexed database. The qualitative questions we intend to explore in the scope of this study are as follows:
- What is the number of scientific articles and reviews published between the year 2000–2021 in the scope of AI and sustainable agriculture?
- What is the growth trajectory in the number of works published in the area of AI and Sustainable agriculture?
- What is the geographic distribution of the research work produced around the world in the field of study?
- Which are the prominent journals and who are the eminent authors involved in the research of AI and sustainable agriculture?
Following this introduction, this article is structured in the following manner: in section 2 the materials and methods used are elaborated. Further, in section 2.1 the bibliometric analysis approach is explained, followed by section 3 which provides an overview and results, and finally, the article closes with sections 4, 5, and 6 providing a discussion about future research options, implications in the present and conclusion respectively.
2. Research methodology
The approach taken to conduct this study is a comprehensive bibliometric analysis of the previously published works of literature that incorporate the usage of AI in the field of sustainable agriculture between the duration of 2000 and 2021. A total of 637 articles were extracted from the Scopus database as a CVS file, out of which only 465 relevant journal articles and review papers were considered for maintaining the veracity of the resulting conclusions. Scopus as a source index, is highly regarded amongst academicians and researchers for searching legitimate scientific articles as it facilitates searching and extraction of specific keywords from the titles, citations, abstracts or keywords from the publications listed in the database [ 14 ].
Bibliometric analysis has been regarded as a reliable method to perform quantitative and empirical study and compilation of previously published works of literature in any field [ 15 ]. Pritchard in 1969 first vaguely defined bibliometrics as analyzing books, written documents, article or media communication by application of statistical and mathematical tools [ 16 ]. Broadus then in 1987 defined bibliometrics as “the quantitative study of physical published units, or of bibliographic units, or of the surrogates for either [ 17 ]". In the scope of this study it encompasses analysis methods such as citation network analysis, geographic network analysis, prominent countries and authors ranked and prominent word cloud. These tools provide a visual representation of the development of literature in the specified field over the course of time and highlight the impactful trending areas of research in the duration selected [ 18 ].
Visualization of the networks established from the large number of articles extracted is a key step in the bibliometric process which was done using multidimensional scaling there are various software tools available in the market like R package, iGraph package, VOSviewer and Biblioshiny [ 19 ]. Due to constant innovation and increased accessibility of advanced web-based and electronic bibliographic and referencing applications, the outputs from bibliometric analysis have greatly improved in quality [ 20 ]. Within the scope of this study, VOSviewer has been used to generate and visually represent the network of authors and countries as the VOSviewer platform provides the most suitable options for easily displaying the bibliometric maps and is easily understandable by any type of audience. Biblioshiny is used in the study to generate the prominent keyword cloud. It is an extension of the Bibliometrix R package web-interface used to visualize the clusters in the database.
2.1 Research method: Bibliometric study
The procedure followed in this study was standard comprehensive bibliometric analysis which starts from keyword search in the Scopus search syntax as shown in the first step of Fig 1 . The keywords indicate that articles and publications containing “Artificial Intelligence” or “Machine Learning” or “Robotics” etc. AND “Sustainable Agriculture” in their titles, keywords, or abstracts. The search was run, and the total number of articles obtained within the timespan of 2000 to 2021 and before any type of filtration was 637. The output was then refined for any redundancies. Thereafter, only journal articles, review papers, selecting only English papers, and within year 2000–2021 were considered for the study, bringing the total number of articles to 465.
- PPT PowerPoint slide
- PNG larger image
- TIFF original image
https://doi.org/10.1371/journal.pone.0268989.g001
This total consists of about 85.8% journal articles and 14.2% review papers. Further, about 46.6% of the publications were made during or after the onset of the Covid-19 pandemic. This set of indexes was then exported to Excel, VOSviewer, and Biblioshiny to obtain the network analysis, assessment of the growth trajectory, geographic distribution, identification of prominent authors, and keyword assessment. The ranking of country-wise publication of articles and citations were determined using Microsoft Excel features.
3. Results and overview
The Table 1 describes the important properties of the data set used for the study which helps in determining the overall scholarly or academic impact that has been made in the field of AI utilized in sustainable agriculture in the duration of 2000 to 2021. The total size of the literary works extracted for the study is 465. One of the most notable properties of this data set is that out of a total of 2005 authors who publish research in this field only 15 of them have produced single author documents. When compared to bibliometric analysis in other popular fields of academic study, these statistics have lower numbers which indicate that there is a need for more academic research and publication to provide knowledge and encourage the use of AI widely.
https://doi.org/10.1371/journal.pone.0268989.t001
3.1 Year-wise article statistics
Further, Fig 2 is used to visually depict the growth trajectory and shift of research interest in the use of AI in sustainable agriculture. The academic literature in this discipline had steady but moderate growth from the year 2000 to 2015. After which evidently, there was a greater impact Industry 4.0 had on sustainable agriculture practices and hence we can see the trend gradually increasing since 2017 and we see an approximately 255.7% increase in Scopus indexed articles and review published from 2019 to 2020 and mid 2021. This growth confirms a surge in scholarly inclination towards addressing the uses of AI in sustainable agriculture practices.
https://doi.org/10.1371/journal.pone.0268989.g002
3.2 Country wise article statistics
In terms of the geographic distribution of the literary works, as per Table 2 about 54.8% of the publications are produced from China ranking it at number 1 out of top 20. The next countries leading knowledge production in use of AI in sustainable agriculture are USA, India, Iran, and France. The rest of the list also comprises of highly developed Anglo-American-European nations such as, Italy, UK, Germany, Spain, Australia, Netherlands, Turkey, Canada, Switzerland and Portugal, and other developing Asian economies such as Malaysia, Indonesia and Pakistan.
https://doi.org/10.1371/journal.pone.0268989.t002
3.3 Country wise citation statistics
The country wise citation ranking in Table 3 , shows USA and China received a maximum number of citations 1938 and 1141, respectively. Then, the following countries with a considerable number of received citations are UK, Netherlands, and Germany. Whereas, Algeria shows the highest average article citation at 68.
https://doi.org/10.1371/journal.pone.0268989.t003
3.4 Ranking of key journals in the selected discipline
It is important for promising scholars to identify the role of prominent journals which are facilitating knowledge dissemination in the discipline of the use of AI in sustainable agriculture so that they can approach the industry leaders and foster further innovation and research potentials. In our analysis, we identified the top 20 journals that span into a wide variety of research disciplines ( Table 4 ). To highlight a few, Sustainable practices (Sustainability), agricultural economy (Agronomy, Agronomy For Sustainable Development), technology in agriculture (Computers and Electronics in Agriculture-81 papers), environmental research (Science of the Total Environment, Journal of Cleaner Production, Agricultural Water Management), emerging technologies (Remote Sensing, Applied Sciences), Multidisciplinary (IEEE Access), environmental and sustainability studies (Land Use Policy), Environmental Monitoring and Assessment (Agriculture Ecosystems and Environment, Ecological Indicators) and the list includes furthermore areas. This indicates that there is a growing inclination towards cross-disciplinary exploration in attaining AI systems that can help make agriculture more sustainable in the future.
https://doi.org/10.1371/journal.pone.0268989.t004
3.5 Ranking of key authors in the selected discipline
Using biblioshiny package in R-software, we have generated Table 5 , which indicated the most prominent authors publishing in the domain of AI utilization in sustainable agriculture and farming. Based on the filtered database the papers, articles, and reviews are produced by 2005 authors in total. The top published authors come from China, USA, India, Iran and France based on their affiliate universities. The low number of articles even from the top authors suggest that this field of study is still in its emerging stages and increasing authorship and co-authorship has the potential of drawing further interest in this discipline.
https://doi.org/10.1371/journal.pone.0268989.t005
3.6 Ranking of key institutions in the selected discipline
The top ten affiliations by the number of articles produced are shown in Table 6 . It is essential to determine which distinguished organizations and universities are leading the research scenario in the field of AI utilization in sustainable agriculture and related disciplines. According to the output from the analysis, Wageningen University, Netherlands has produced 13 scientific articles in the scope of the topic under study. The following China Agricultural University, and Northwest A&F University are from China, and each have published 12 and 10 scientific articles, respectively.
https://doi.org/10.1371/journal.pone.0268989.t006
3.7 Keyword analysis
The result of the keyword analysis using Biblioshiny shows precisely the various technologies within the scope of artificial intelligence which are being used in sustainable agriculture. These technologies are–machine learning, agricultural robots, neural networks, artificial neural networks, remote sensing, precision agriculture and support vectors machines. In addition to it, Fig 3 provided the major keywords and its occurrence in the selected articles. It is evident apart from the keywords of artificial intelligence and agriculture which are under study, the most projected key words are agricultural robots, decision making, machine learning, remote sensing, artificial neural systems, logic algorithms and other related technologies.
https://doi.org/10.1371/journal.pone.0268989.g003
The projected set of keywords also helps in determining the various thematic areas in which the majority of the articles belong. The popular thematic areas determined are automation of agricultural production using AI, machine learning in the field of food production, modernization of farming methods using technology and weather forecasting solutions for sustainable agriculture.
3.8 Network analysis
The Fig 4 , is a VOSviewer generated network illustration of the most prominent published author collaborations for AI and Sustainable agriculture scientific articles that are indexed in Scopus from 2000–2021. The eight different color clusters represent the different domains and the interaction and collaboration of authors from different domains to produce multi-disciplinary scientific articles. The size of each circle denoted for each author represents the amount of academic literature, citations they have produced in the field of AI and sustainable agriculture respectively.
https://doi.org/10.1371/journal.pone.0268989.g004
Based on the originating country of publication and using VOSviewer software the geographic network distribution is obtained in the form of Fig 5 . The network also reinforces the findings from the bibliometric analysis of the top 20 countries that produce scientific studies in the domain of AI and sustainable agriculture. The different colored clusters represent the various disciplines of study and their interaction with other disciplines in the scope of the study. Further, the circles representing China, the United States, India, the UK, and Germany confirm the earlier findings. On further exploration of Fig 5 , it is observed that the clusters created between the United States of America, European Union member states and United Kingdom are more dense and diverse as compared to the interaction with and amongst Asian countries.
https://doi.org/10.1371/journal.pone.0268989.g005
4. Discussion
AI enhancements have made different sectors of production highly effective in doing so by providing sustainable solutions. Initially, the applications of AI technologies were intended to increase productivity but the trend had begun to gradually shift towards researching for sustainability and green technologies that would automate and reduce resource consumption in the production process. Jha et al. in their review of automation used in agriculture, highlighted that the younger farmers and producers are more inclined towards investing in automation technologies than the older generations [ 21 ]. Over utilization of pesticides and agrochemicals has led previously fertile lands to turn barren, this can be avoided by implementing Artificial Neural Network methods as suggested in the results obtained by Elahi et al. in their 2019 test on rice crops [ 22 ]. Studies have also emerged in utilization of data intelligence automation tools like extreme machine learning in precision farming decision support system to determine the accurate yield of crops of small holder farms of produce such as coffee [ 23 , 24 ].
The aim of this article is to compile and reach various inferences from the bibliometric analysis of the literature published in the duration of 2000–2021. The research is innovative and a novel study trying to explore the AI techniques utilised specifically in sustainable agriculture practices which have not been done before. The resulting research framework is intended to provide a basis for future research in an efficient and organised manner.
4.1 AI in sustainable agriculture
The use of AI in sustainable agriculture has the potential to transform aspects of farming such as image sensing for yield mapping, yield prediction, skilled and unskilled workforce, increasing yield and decision-support for farmers and producers [ 25 ]. Based on a 2019 article by Alreshidi, AI is widely being implemented in the following ways to make agriculture more sustainable, these are- climate monitoring, automatically climate-controlled greenhouses, crop quality monitoring, livestock management, predictive analysis and comprehensive farm management systems are a few [ 26 ]. The AI products that are highly in demand from customers of sustainable agriculture backgrounds are chatbots for help with information on farming practices, digital plant health diagnosis applications, remote sensing instruments and irrigation management solutions [ 27 ].
Klyushin & Tymoshenko, (2021) proposed an optimization approach for drip irrigation system optimization to attain sustainable agriculture by using AI methods [ 28 ]. On the other hand, Zhang et al. (2021b) emphasized on informatics and material science to find sustainable solutions in sustainable agriculture by using nanotechnology and AI [ 29 ]. Aggarwal & Singh (2021) asserted on technology assistance in precision farming and discussed the implications of AI and internet of things (IoT) in agriculture to assess water requirements, humidity, need for fertilizers etc [ 30 ]. Spanaki et al. (2021) addressed the issues concerning food security and proposed an AI technique as a solution by adopting a design science methodology [ 31 ]. Mohr & Kühl (2021) investigated the barriers in AI acceptance in agriculture and applied technology acceptance model [ 32 ]. Nevertheless, Mahto et al. (2021) used artificial neural network (ANN) to forecast prices of agriculture commodities and compared of performance of their model with ARIMA model for sustainable agriculture [ 33 ].
4.2 Machine learning in sustainable agriculture
Machine learning was defined in the 2018 book “Foundations of Machine Learning” as computational processes that utilize historic data and past experiences to modify, improve, repair and predict future performance accurately [ 34 ]. Machine learning in sustainable agriculture latest utilization is in optimizing supply chains [ 35 ], in-field monitoring [ 36 ], soil temperature prediction [ 37 ] and sustainable soil management [ 38 ]. The different types of machine learning technologies that can be implemented to foster sustainable production are decision trees, neural networks, polynomial predictive methods and K-nearest neighbors [ 39 ]. Traditional methods of soil suitability assessments for sustainable agriculture can prove to be expensive and time taking especially in remote areas where data about the properties of the soil is unavailable there machine learning technologies are gaining popularity for large scale land suitability assessments [ 40 ].
Qin et al. in 2018 successfully explored the predictive abilities of machine learning on estimating the economic optimum nitrogen rate for corn crops using data from 47 test conducted throughout the American corn belt in USA and found that more robust data was required to make accurate estimations using machine learning based models [ 41 ]. Liakos et al. in 2018 conducted a review of machine learning in agri-tech and found that the artificial and deep neural network method of machine learning was a popular choice across all categories of agriculture processes but specifically in the categories of livestock management, water management and soil management [ 42 ]. A 2019 comparative study between four different machine learning techniques by Ju et al., concluded that while conducting estimations on corn and soybean yields, Convolutional Neural Network or CNN was the most accurate [ 43 ].
4.3 Robotics in sustainable agriculture
Robotics in agriculture is mainly utilized to speed up repetitive and mundane tasks in the production process like spraying, mowing, seeding, harvesting, weed control, picking and finally in sorting products and packaging. Automation provided by robotics in combination with cloud computing, block-chain and big data has also found utility in supply chain of fresh produce. In the realm of making farming sustainable, field robots are used in precision farming by targeted weed control functions replacing treatment of crops and soil with harmful and excessive chemical sprays [ 44 ]. The concept of Agriculture 5.0 has been gaining momentum as a term used to define the incorporation of artificial intelligence and robotics in data-driven farming systems [ 45 ].
Sarri et al. in 2020 reported the results of SMASH project at its design stages of AgroBot with four modules combined to physically control weeds and protection of crops [ 46 ]. Robotic solutions are not only popular in the research community but also amongst the members of the industry who want to invest and implement these sustainable systems [ 47 ]. With the widespread utility, there is an emergence in new research interest in safe human-robot interactions in agricultural settings. Benos et al., express that due to automation and programmed robots, human safety is a concern and that efforts must be taken to make robots extra sensitive to perceiving human proximity and ensure risk-free work environment [ 48 ].
The article by Linaza et al., from 2021 summarises the recent research projects in European Union on the use of robotics and highlights that the use of robots can not only make it precise but also solve the labour shortages caused rise in the average age of farmers [ 49 ]. Furthermore, Mondejar et al., described that usage of robotics in agriculture can solve major food shortage issues and help achieve United Nations sustainable development goals without depleting non-renewable resources rapidly [ 50 , 51 ]. However, to bring the robotic technologies to the commercial level some barriers that must be overcome are improved speed and accuracy. There is a scarcity of research funding when compared to investment interests in industrial manufacturing and military equipment that’s why the body of study is small and the process from development to implementation is slow and on small scale [ 52 – 54 ].
4.4 Resulting observations made in the use of AI techniques in sustainable agriculture
There are a number of other AI solutions that can be implemented in ensuring sustainability in food production like predictive analytics, decision support systems, genomics tracing, artificial neural networks, fuzzy logic, neuro-fuzzy logic, Bayesian Network, and remote sensing. Studies have suggested that advanced bio-sensing technologies in sustainable agriculture will facilitate early diagnosis of diseases and plant pathogens even in asymptomatic plants hence reducing the loss of crops and production [ 55 ]. Unmanned Arial Vehicles or drones integrated with advanced machine learning help in continuous weed management enabling selection decisions and reducing herbicide diffusion in the environment [ 56 ]. Also, Rasmussen et al., in 2021 studied the effect of engaging unmanned arial vehicles which receive data from satellite imagery to perform weed mapping of a particular variety of weed and the result showed that it provides higher resolution images and makes an individual variety of weed to be detected in the crops [ 57 ].
AI and genomics research can enhance defect detection and improve production gains per unit of time with genetic historical data for making accurate predictions [ 58 – 60 ]. Artificial neural networks and fuzzy logic on the other hand are systems that can be continuously trained in making prediction models for sustainable agriculture [ 21 ]. A recent 2021 stimulation study by Pazouki, devised a system for surface irrigation using fuzzy expert systems and meta-heuristic optimization algorithms which enhanced the regular system and improved its practicality, performance and reduced the requirement for manual labour [ 61 ]. Hence proving the wide application of the system in irrigation and water management for sustainable agriculture.
On the other hand, the application of fuzzy logic was used in another study by Soylu and Çarman in 2021 where they developed an automatic fuzzy-based slip control system for tractors on the field by continuously recording how many times slippage occurred during the process of tilling, which proved to reduce slippage by 42% and reduced fuel consumption by 44% [ 62 ]. A few limitations of the artificial intelligence-based systems were highlighted in the recent 2021 study by Meroni et al., in which they stated that the difference between the accuracy of machine learning enabled systems and regular bench-marking systems were not significantly dispersed and the performance of machine learning systems reduced if the data was small [ 63 ].
Table 7 briefly discussed some recent studies conducted in the selected field of research. Based on previous literature and identified techniques used in providing solutions for sustainable agriculture, a research framework is developed in this study ( Fig 6 ).
https://doi.org/10.1371/journal.pone.0268989.g006
https://doi.org/10.1371/journal.pone.0268989.t007
Based on the results and further propositions from the above stated recent studies, we have attempted to propose a framework for optimizing future research in the utilization of AI technology in sustainable agriculture. We propose that, from Fig 6 depicted below, researchers can create novel topics and thematic areas utilizing the classification of operations performed in agriculture at various stages. Then choose relevant and correlatable AI technologies as in Fig 6 , that have been identified based on existing academic literature and research its application on the distinct processes identified in attaining sustainable agriculture practices.
The suggested framework can also provide a foundation to attract new researchers to the direction of researching this avenue as it is observed that the size of existing scholarly research is relatively very small between 2000–2021. There is an ethical need to motivate further scholastic interest in the development of modern technology usage in the food supply chain to ensure food safety for future generations owing to rapid depletion of resources and global environmental and climate changes.
5. Future research
The potential for future research is vast in the scope of sustainability studies involving advanced technologies and sustainable agriculture as these fields interact frequently in the market’s demand for newer innovations. In terms of bibliometric exploration of previous literary works, further scrutiny could be performed by using sophisticated R bibliometrix programming for functions such as co-occurrence analysis, co-citation analysis, cluster and stream analysis etc. The size of the dataset used for the studies can also be increased to include more technologies such as block chain, Internet of Things, cloud computing, and supply-chain solutions, which are extensively utilized in the agriculture industry. Further studies could also combine scholarly articles and reviews from more than one index source such as Scopus and Web of Science together for extracting a larger dataset. But this kind of dataset will require further steps to clean the dataset from duplications and redundant information.
6. Implications
The implications of the results from this study can be of significant impact as it provides the targeted scholars, readers and researchers with a comprehensive compilation of the body of research conducted previously in the area of sustainable agriculture and AI. The results imply that the interest in the exploration of diverse applications of AI in sustainable agriculture is steadily rising. Distinguished authors from multidisciplinary backgrounds and expertise are inclined towards combining it with other thematic domains around the world.
It also indicates that there is potential to publish more literary and research articles as the body of literature are not as large compared to other bibliometric studies. This article also brings the attention of the readers to this untapped opportunity, and they can get motivated to fill this research gap. The proposed research framework in Fig 6 , also has the potential to further provide a streamlined approach to building pertinent scholarly research topics in this particular thematic area.
7. Conclusion
In our analysis, we classified the keywords and used them to extract 674 relevant articles and reviews which gave us the following results: there is a rising academic interest in the field of AI usage in sustainable agriculture with a drastic improvement from 2019 to 2020. China, the USA and Australia are leaders in producing top works of literature and authors in the domain. These results were obtained by performing data cleansing and classifying the data set for network analysis using functions of Microsoft Excel, VOSviewer, and Biblioshiny. By analysing the results it can be determined that there is huge potential for the application of AI to attain sustainability, especially in predicting the yield, crop protection, climate control, crop genetic control, and produce supply-chain, wherein the prominent researchers and institutions need to collaborate further and form more networks to bring radical progress in the field.
As we proceed to the future, focusing on sustainability will dictate all aspects of life on earth. The first and foremost challenge with climate change will be ensuring food safety and availability for all. Although the green revolution and industrial revolution in the past had exponentially improved food production capacities, these approaches also exerted intolerable pressure on the agricultural lands, natural resources, and the ecosystem, the implications of which are unsustainable and irreversible. The 4th industrial revolution with its hi-tech capabilities promised sustainable alternatives to traditional agriculture practices which can potentially reduce or slow down the depletion of the earth’s resources. As the Covid-19 pandemic shocked and paused the usual shenanigans of every sector for a while, it is a good time to review the body of work in AI and sustainable agriculture before making our way forward.
Supporting information
https://doi.org/10.1371/journal.pone.0268989.s001
- View Article
- Google Scholar
- 3. Cataldo R., Grassia M.G., Lauro C.N., Marino M., Voytsekhovska V. A Bibliometric Study of the Global Research Activity in Sustainability and Its Dimensions. In Studies in Classification , Data Analysis , and Knowledge Organization . Proceedings of the International Conference on Data Science and Social Research II, Milan, Italy, February 4–5, 2019, Mariani P., Zenga M. (eds) Springer, Cham. 91–102.
- PubMed/NCBI
- 13. Russell S., & Norvig P. Artificial Intelligence A Modern Approach . New Jersey: 1995,Prentice Hall.
- 34. Mohri M., Rostamizadeh A., & Talwalkar A. Foundations of Machine Learning . Cambridge, Massachusetts, USA 2018. The MIT Press.
- 43. Ju, S., Lim, H., & Heo, J. Machine Learning Approaches for Crop Yield Prediction with Modis and Weather Data. 40th Asian Conference on Remote Sensing: Progress of Remote Sensing Technology for Smart Future, ACRS 2019, Daejeon: Asian Association on Remote Sensing,1–4.
- 46. Sarri, D., Lombardo, S., Lisci, R., De Pascale, V., & Vieri, M. AgroBot Smash a Robotic Platform for the Sustainable Precision Agriculture. In Innovative Biosystems Engineering for Sustainable Agriculture , Forestry and Food Production . Proceedings of the International Mid- Term Conference 2019 of the Italian Association of Agricultural Engineering (AIIA), Matera, Italy, September 12–13, 2019; Coppola A., Carlo Di Renzo G. Eds.,793–801.
- 47. Cavallone, P., Botta, A., Carbonari, L., Viscont, C., & Quaglia, G. The Agri.q Mobile Robot: Preliminary Experimental Tests. In Advances in Italian Mechanism Science . Proceedings of the 3rd International Conference of IFToMM, Naples, Italy, September 9–11, 2020; Niola V., Gasparetto A. Eds., 524–532.
- Sustainable Agriculture Research
- Announcements
SAR, Vol. 13, No. 2, November 2024: Call for Papers
Posted on Apr 4, 2024 Sustainable Agriculture Research (sar) is calling for papers for the Vol. 13, No. 2, November 2024 issue (Deadline: October 1, 2024). You are cordially invited to submit manuscripts... Read More
SAR Indexed in ERA
Posted on Jul 5, 2019 Sustainable Agriculture Research (SAR) has been indexed in ERA, Excellence in Research for Australia, Australia’s national research evaluation framework. ERA identifies and promotes excellence across the full spectrum of research activity in Australia’s h Read More

Policy Change of Free Print Journals
Posted on Jan 25, 2018 As you are aware, printing and delivery of journals results in causing a significant amount of detrimental impact to the environment. Being a responsible publisher and being considerate for the envi.. Read More
Current: Vol. 13, No. 1 (2024)
- Large Scale Nationwide Screening of Bioavailable Tetracyclines and Arsenic Using Whole-cell Bioreporter from Pangasius and Tilapia Aquaculture System in Bangladesh
- Emranul Ahsan
- Mohammad Abdur Razzak
- Seikh Razibul Islam
- Ajmala Akter
- Lokman Ali

- Impact of Biochar Applications on Tropical Soils under Different Land-use Regimes
- Khasifah Muhamad
- Uchenna Ogbonnaya
- John Quinton
- Kirk Semple
- Farmers’ Perception of Indigenous Seasonal Forecast Indicators in North Central Burkina Faso
- Pamalba Narcise Kabore
- Aboubacar-Oumar Zon
- Dasmane Bambara
- Souleymane Koussoubé
- Amade Ouedraogo
- Utilization of Black Soldier Fly (Hermetia illucens) Larvae as a Potential Substitute for Fish Meal in the Production of Nile Tilapia (Oreochromis niloticus L.)
- Jonathan Munguti
- Francis Wekesa
- Isaac Osuga
- Mercy Kariuki
- Rodrigue Yossa
- Daniel Mungai
- Domitila Kyule
- Jacob Abwao
- Mary Opiyo
- Kevin Obiero
- Nicholas Outa
- Erick Ogello
- Jacob Iteba
- James G. Kirimi
- Anne Maundu
- David Liti
- Chrysantus M. Tanga
- Passive Solar Greenhouse-A Sustainable Option for Propagating Sweet Potato for Colder Climatic Regions
- Sajjad Ali Rao
- Poonam Singh
- Production Systems and Management Practices of Chicken Populations in Zambia
- Sylvia Jana Harrison
- Mayoba Barbara Moono
- Idowu Kolawole Odubote
- Determinants of Smallholder Farmers’ About Choices of Sustainable Land Management Practices in West Wollega Zone, Oromia Region, Ethiopia
- Addisu Raga
- Fekadu Beyene
- Jema Haji
- Chanyalew Siyum
- Merging Interest for Sustainability Agenda: Is There a Link between Sustainable Agriculture Practices and Farm Efficiency?
- Mahendra Reddy
- Between Professionalism and Amateurism in the Use of the Agricultural Training Videos: Lessons Learnt from Experimental Auctions in Benin
- Gerard C. Zoundji
- Esperance Zossou
- Dossou S. Wilfilas Awanvoeke
- Simplice D. Vodouhe
- Reviewer Acknowledgements for Sustainable Agriculture Research, Vol. 13, No. 1
- Joan Lee
What is e-Version First TM
e-Version First is a feature offered through our journal platform. It allows PDF version of manuscripts that have been peer reviewed and accepted, to be hosted online prior to their inclusion in a final printed journal. Readers can freely access or cite the article. We aim to publish accepted manuscripts in e-Version First in two week's time after the final draft completed.
Each paper published in Journal of Agricultural Science is assigned a DOI®number , which appears beneath the author's affiliation in the published paper. Click HERE to know what is DOI (Digital Object Identifier)? Click HERE to retrieve Digital Object Identifiers (DOIs) for journal articles, books, and chapters.
Competing Interests Statement
All authors of Sustainable Agriculture Research are requested to disclose any actual or potential conflict of interest including any financial, professional, personal or other relationships with other people or organizations, along with the paper. Download MS-Word
Paper Selection and Publication Process
a). Upon receipt of paper submission, the Editor sends an E-mail of confirmation to the corresponding author within 1-3 working days. If you fail to receive this confirmation, your submission/e-mail may be missed. Please contact the Editor in time for that.
b). Peer review. We use double-blind system for peer-review; both reviewers and authors’ identities remain anonymous. The paper will be peer-reviewed by three experts; two reviewers from outside and one editor from the journal typically involve in reviewing a submission. The review process may take 4-10 weeks .
c). Notification of the result of review by E-mail.
d). The authors revise paper and pay the article processing charge (formatting and hosting).
e). After publication, the authors are requested to kindly complete an application form if they need free print copies.
f). E-journal in PDF is available on the journal’s webpage, free of charge for download.
The publisher and journal have a policy of “Zero Tolerance on the Plagiarism”. We check the plagiarism issue through two methods: reviewer check and plagiarism prevention tool (ithenticate.com).
All submissions will be checked by iThenticate before being sent to reviewers.
-----------------------------------------------------------------------------------------------------------------------------
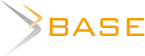
- ISSN(Print): 1927-050X
- ISSN(Online): 1927-0518
- Started: 2012
- Frequency: semiannual
Journal Metrics
WJCI (2021): 0.340
Impact Factor 2021 (by WJCI): 0.434
- CAB Abstracts
- CNKI Scholar
- Directory of Research Journals Indexing
- Electronic Journals Library
- Excellence in Research for Australia (ERA)
- Google Scholar
- JournalTOCs
- PKP Open Archives Harvester
- Qualis/CAPES
- SHERPA/RoMEO
- Standard Periodical Directory
- WJCI Report
- Joan Lee Editorial Assistant
- [email protected]
- Journal Home
- Editorial Team
- Order Hard Copies

An official website of the United States government
The .gov means it’s official. Federal government websites often end in .gov or .mil. Before sharing sensitive information, make sure you’re on a federal government site.
The site is secure. The https:// ensures that you are connecting to the official website and that any information you provide is encrypted and transmitted securely.
- Publications
- Account settings
Preview improvements coming to the PMC website in October 2024. Learn More or Try it out now .
- Advanced Search
- Journal List
- Front Microbiol
Nanotechnology in Sustainable Agriculture: Recent Developments, Challenges, and Perspectives
1 Amity Institute of Microbial Technology, Amity University, Noida, India
Atanu Bhattacharyya
2 Department of Entomology, University of Agricultural Sciences, Gandhi Krishi Vigyan Kendra, Bengaluru, India
Quang D. Nguyen
3 Research Centre of Bioengineering and Process Engineering, Faculty of Food Science, Szent István University, Budapest, Hungary
Nanotechnology monitors a leading agricultural controlling process, especially by its miniature dimension. Additionally, many potential benefits such as enhancement of food quality and safety, reduction of agricultural inputs, enrichment of absorbing nanoscale nutrients from the soil, etc. allow the application of nanotechnology to be resonant encumbrance. Agriculture, food, and natural resources are a part of those challenges like sustainability, susceptibility, human health, and healthy life. The ambition of nanomaterials in agriculture is to reduce the amount of spread chemicals, minimize nutrient losses in fertilization and increased yield through pest and nutrient management. Nanotechnology has the prospective to improve the agriculture and food industry with novel nanotools for the controlling of rapid disease diagnostic, enhancing the capacity of plants to absorb nutrients among others. The significant interests of using nanotechnology in agriculture includes specific applications like nanofertilizers and nanopesticides to trail products and nutrients levels to increase the productivity without decontamination of soils, waters, and protection against several insect pest and microbial diseases. Nanotechnology may act as sensors for monitoring soil quality of agricultural field and thus it maintain the health of agricultural plants. This review covers the current challenges of sustainability, food security and climate change that are exploring by the researchers in the area of nanotechnology in the improvement of agriculture.
Introduction
Agriculture is always most important and stable sector because it produces and provides raw materials for food and feed industries. The limit of natural resources (production land, water, soil, etc.) and the growth of population in the world claim the agricultural development to be economically further, viable, environmentally and efficiently. This alteration will be the vital for achieving many factors in the recent year ( Johnston and Mellor, 1961 ; Yunlong and Smit, 1994 ; Mukhopadhyay, 2014 ). Agricultural nutrient balances are differed noticeably with economic growth, and especially from this surmise, the development of the soil fertility is very much significant in developing countries ( Campbell et al., 2014 ).
The development of agriculture is compulsory phenomena for the purge of poverty and hunger which must be getting rid of from the present situation. Therefore, we should have to take one bold step for agriculture development. In this world mainstream of peoples are below poverty level which are being scatted in the rural area where agriculture enlargement has not so being effective.
Nowadays, the most vital obsession is to create flanked by, agriculture poverty and nutritional process getting food. Therefore, new technology should have to adopt that decidedly focuses on getting better agricultural production ( Yunlong and Smit, 1994 ). Recently, food and nutritional security are fully embedded in the novel knowledge. The agriculture development also depends on the social inclusion, health, climate changes, energy, ecosystem processes, natural resources, good supremacy, etc., must also be documented in specific target oriented goals. Therefore, sustainable agricultural strengthening the practical opportunity to get rid of poverty and hunger of the people. The agriculture on the road to recovery, thus the environmental performance is required and at the same time participation of food chain ecosystems are required in relation to agricultural food production ( Thornhill et al., 2016 ).
No doubt that the sustainable growth of agriculture totally depends on the new and innovative techniques like nanotechnology. Naturally, it haunts us to know what is this important technology? If we like to go in the year 1959 Feynman’s lecture on “Plenty of room at the bottom,” from this very day, the nanoprocess is in underway ( Feynman, 1996 ). Later on Professor Norio Tanaguchi (1974) proposed the actual term of nanotechnology ( Bulovic et al., 2004 ). Afterward, nanotechnology develops more vivid way, as because, more recent instruments develops to consider or isolate nanomaterials in accurate way ( Bonnell and Huey, 2001 ; Gibney, 2015 ). Additionally, the number of publications related to the term of “nano” was also grown exponentially. Figure Figure1 1 demonstrates the number of documents on scopus.com (accessed date: March 15, 2017) with the search term of “Nano and (Food and Agriculture).” In 2016, about 14,000 documents with nanotechnology in food or agriculture were listed meaning high activities of this field. Also about 2707 patents matched this criteria are found in world patent database 1 . The world market size of nanotechnology in 2002 was about US$ 110.6 billion and predicted to grow to US$ 891.1 billion in 2015 according to analysis of Helmut Kaiser Consultancy 2 . The developments of nanotechnology in materials and electronics have higher dynamics than other applications ( Figure Figure2A 2A ). Recently, food and agriculture also require high amount of nanomaterials especially in packaging. The NAFTA region shares the biggest slice from the market size ( Figure Figure2B 2B ), but Europe and Asia especially China, Japan, and India also come up very dynamically.

Number of documents on scopus.com with the search term “Nano and (food or agriculture). The hits were grouped annually. Accessed date: March 15, 2017.

World market of nanotechnology by applications (A) and regions (B) . Source of data: Helmut Kaiser Consultancy (hkc22.com), Accessed date: March 15, 2017.
It is well known that one billionth of a meter is one nanometer (nm). Why this nano will change its property? It is due to alteration in the atoms and develops a magnetic power. It can be projected that the smaller size of nanomaterial possesses larger surface area and exhibits more active. With this course of action nanotechnology is knocking the doors of perception. The magnetic property of polymer develops due to tellurium atoms; antimony-bismuth; and sulfur atoms. Moreover, it has been observed that when the atoms of do pant and atoms of europium interact together, and then the entire molecules carry out the magnetic property. Thus the alter property of nanomaterials is related with more reactive in the most sectors including in biological process ( Pokropivny et al., 2007 ; Prasad, 2014 ). This ultimate technology possesses several unique electronic association, plasmonic and optical properties which are related with the quantum confinement effects, the alteration of the electronic energy levels may appear due to the surface area in relation to volume ratio ( Sun, 2007 ; Aziz et al., 2015 ; Prasad et al., 2016 ). In the present century, there is a big demand for fast, reliable, and low-cost systems for the detection, monitoring, and diagnosis for biological host molecules in agricultural sectors ( Vidotti et al., 2011 ; Sagadevan and Periasamy, 2014 ). The application of chemically synthesize nanomaterials now a days considered as toxic in the nature, in attract of this, nanomaterials may synthesis from plant system and it considered as green nanotechnology ( Prasad, 2014 ). Green nanotechnology is a safe process, energy efficient, reduces waste and lessens greenhouse gas emissions. Use of renewable materials in production of such products is beneficial, thus these processes have low influence on the environment ( Prasad et al., 2014 , 2016 ). Nanomaterials are eco-environmentally sustainable and significant advances have been made in the field of green nanotechnology. In the present decade, it is more shift toward the green nano in a faster rate for implementation its functions. Still it is not clear how the environmental sustainability of green nanotechnology will be achieved in future? These risks must be mitigated in advancing green nanotechnology solutions ( Kandasamy and Prema, 2015 ).
In modern agriculture, sustainable production and efficiency are unimaginable without the use of agrochemicals such as pesticides, fertilizers, etc. However, every agrochemical has some potential issues including contamination of water or residues on food products that threat the human being and environmental health, thus the precise management and control of inputs could allow to reduce these risks ( Kah, 2015 ). The development of the high-tech agricultural system with use of engineered smart nanotools could be excellent strategy to make a revolution in agricultural practices, and thus reduce and/or eliminate the influence of modern agriculture on the environment as well as to enhance both the quality and quantity of yields ( Sekhon, 2014 ; Liu and Lal, 2015 ).
The development of biosensors is also a good field for exploitation of many strengths of nanotechnology, thus nanotechnology is there and plays an essential role. Due to special properties of nanomaterials, on one hand, the sensitivity and performance of biosensors could be improved significantly in their applications ( Fraceto et al., 2016 ); on another hand, many new signal transduction technologies are let to be introduced in biosensors ( Sertova, 2015 ). Additionally, use of nanomaterials let to miniaturize many (bio)sensors to small and compact/smart devices such as nanosensors and other nanosystems that are very important in biochemical analysis ( Viswanathan and Radecki, 2008 ; Sertova, 2015 ; Fraceto et al., 2016 ). It also helps to detect the mycotoxins present in several foods and their functions are very rapid ( Sertova, 2015 ).
Nanoparticles and Their Functions
Carbon nanotubes (cnts).
It is a new form of carbon, equivalent to two dimensional graphene sheet rolled into a tube. Two main types of nanotubes are single-walled nanotubes (SWNTs) and multi-walled nanotubes (MWNTs). Its tensile strength ∼200 GPa, thus ideal for reinforced composites and nanoelectro mechanical systems. Moreover, metallic or semiconducting and offers amazing possibilities to create electronic circuits, or even complete nanodevices. Structurally, the nanotube systems consist of graphitic layers seamlessly wrapped into cylinders. Recently, fluorescent nanoparticles (NPs) or quantum dots (QDs) have been developed for labeling the plant proteins ( Pyrzynska, 2011 ; Chahine et al., 2014 ).
No doubt that properties (mechanical, electronic, thermal, optical, elastic, etc.), and thus applicability of CNTs were determined by geometrical dimensions especially by diameter. Diameter of most SWNTs is about 1 nm and strongly correlated to synthesis techniques, mixing of σ and α bonds and electron orbital rehybridization. Exploitation of these properties of CNTs definitely will open new possibilities to develop many types of nanodevices which confers unique conductive, optical and thermal properties for applications in agri-field and in development of sustainable agricultural conditions ( Raliya et al., 2013 ). Agrochemicals or other substances can be targeted to hosts by delivery systems based on CNTs, thus led to reduce the amount of chemicals released into the environment as well as the damage to other plant tissues ( Raliya et al., 2013 ; Hajirostamlo et al., 2015 ).
Camilli et al. (2014) reported that the absorption of the toxic organic solvent dichlorobenzene from water increased about 3.5 times by some CNTs nano-sponges than CNT powder. Generally, the use of CNT nano-sponges containing sulfur and iron increases efficiency in soaking up water contaminants such as pesticides, fertilizers, oil, and pharmaceuticals. Unfortunately, under certain circumstances CNTs may cause vitality of human cells by penetrability and accumulability in the cytoplasm ( Porter et al., 2007 ).
Quantum Dots
Generally, semiconductor QDs are high quantum yield and molar extinction coefficients, broad absorption spectra with narrow, symmetric fluorescence spectra spanning the ultraviolet to near-infrared, large effective excitation, high resistance to photobleaching and exceptional resistance to photochemical degradation. Thus these are excellent fluorescence, quantum confinement of charge carrier’s materials and possess size tunable band energy ( Bulovic et al., 2004 ; Androvitsaneas et al., 2016 ). QDs have unique spectral properties compared with traditional organic dyes, thus recently, they have been applied as a new generation of fluorophores in bioimaging and biosensing ( Bakalova et al., 2004 ). QDs also function as photocatalysts for the light driven chemical conversion of water into hydrogen as a pathway to solar fuel ( Konstantatos and Sargent, 2009 ). QDs at low concentration revealed no detectable cytotoxicity for seed germination and seedling growth. Therefore, based on this transport approach, QDs can be utilized for live imaging in plant root systems to verify known physiological processes ( Hu et al., 2010 ; Das et al., 2015 ).
Multifunctional plasmonic materials which can couple sensing phenomenon well and size tunable energy regulation, can be coupled with MEMS, and induce specific field responses ( Bulovic et al., 2004 ). The gold nanorods significantly physiological changes occurred of watermelon plant and confirmed phytotoxicity toward plant particularly at high concentration ( Wan et al., 2014 ) and also ability to transport auxin growth regulator 2,4-D, which resulted in a significant influence on the regulation of tobacco cell culture growth ( Nima et al., 2014 ).
Micro- and Nanoencapsulation
Encapsulation is defined as process in which the given object is surrounded by a coating or embedded in homogeneous or heterogeneous matrix, thus this process result capsules with many useful properties ( Rodríguez et al., 2016 ). The benefits of encapsulation methods are for protection of substances/objects from adverse environments, for controlled release, and for precision targeting ( Ezhilarasi et al., 2012 ; Ozdemir and Kemerli, 2016 ).
Depending on size and shape of capsules different encapsulation technologies are mentioned, while the (macro) encapsulation/coating results capsules in macroscale, whereas the micro- and nanoencapsulation will give particles in micro- and nanoscale size ( Ozdemir and Kemerli, 2016 ). Nanocapsules are vesicular systems in which the substances are confined to a cavity consisting of an inner liquid core enclosed by a polymeric membrane ( Couvreur et al., 1995 ). Recently, micro and NPs are getting significant attention for delivery of drugs, for protection and increase in bioavailability of food components or nutraceuticals, for food fortification and for the self-healing of several materials, and also it possesses big prospective phenomenon in plant science ( Ozdemir and Kemerli, 2016 ). Some drugs such as peptides or anti-inflammatory compounds are successfully nanoencapsulated ( Puglisi et al., 1995 ; Hildebrand and Tack, 2000 ; Haolong et al., 2011 ). The development of nanoencapsulated methods for ligation of targeted tissues to NPs which will make possible to deliver several biologically active compounds to the target tissues ( Pohlmann et al., 2008 ). Furthermore, the development of this technology will build more possibility to create new drugs with precise therapeutic action on embattled tissues. Nanocapsules can potentially be used as MRI-guided nanorobots or nanobots ( Vartholomeos et al., 2011 ).
Nanoemulsions
Nanoemulsions are formed by very small emulsion nanoscale droplets (oil/water system) exhibiting sizes lower than ∼100 nm ( Gutiérrez et al., 2008 ; Anton and Vandamme, 2011 ). Although fundamentally significant differences between nanoemulsions and microemulsions could not be exists, but in fact, the physical properties of nanoemulsions can be quite different from those of microscale emulsions ( Mason et al., 2006 ; Gupta et al., 2016 ). Due to the size of droplets, the ratio of surface area to volume, Laplace pressure and elastic modulus of nanoemulsions are significantly larger than that of ordinary emulsions. Moreover unlike general emulsions, most of nanoemulsions appear optically transparent that, thus, technically have many advantages make such us incorporation into drinks. Unfortunately, the formulation of nanoemulsion needs very high energy, thus it requires some special devices that are able to generate extreme shear stress such as, high pressure homogenizator or ultrasonic generator ( Asua, 2002 ; Gupta et al., 2016 ). Tadros et al. (2004) reported “low-energy” method for formation of nanoemulsions and in this process, two liquid phases (one is a homogeneous liquid consisted of lipophilic phase and hydrophilic surfactant plus potentially a solvent, polymer or drug, and the other is an aqueous phase, even pure water) are bought into contact of this phase. Then the hydrophilic species contained in the oily phase is rapidly solubilized into the aqueous one, inducing the demixation of the oil in the form of nano-droplets, instantly stabilized by the amphiphiles ( Anton and Vandamme, 2009 ; Gupta et al., 2016 ). This method thus is seemed to be simplest and does not require any special devices with high energy.
Nanotechnology and Agricultural Sustainable Development
The nanotechnology can takes an important part in the productivity through control of nutrients ( Gruère, 2012 ; Mukhopadhyay, 2014 ) as well as it can also participate in the monitoring of water quality and pesticides for sustainable development of agriculture ( Prasad et al., 2014 ). Nanomaterials have such diverse assets and activities that it is impossible to deliver a general assessment of their health and environmental risks ( Prasad et al., 2014 ). Properties (other than size) of NPs have the influence on toxicity include chemical composition, shape, surface structure, surface charge, behavior, extent of particle aggregation (clumping) or disaggregation, etc. may associate with engineered NPs ( Ion et al., 2010 ). For this reason even nanomaterials of the same chemical composition that have different sizes or shapes can exhibit their different toxicity. The implication of the nanotechnology research in the agricultural sector is become to be necessary even key factor for the sustainable developments. In the agri-food areas pertinent applications of nanotubes, fullerenes, biosensors, controlled delivery systems, nanofiltration, etc. were observed ( Ion et al., 2010 ; Sabir et al., 2014 ). This technology was proved to be as good in resources management of agricultural field, drug delivery mechanisms in plants and helps to maintain the soils fertility. Moreover, it is being also evaluated steadily in the use of biomass and agricultural waste as well as in food processing and food packaging system as well as risk assessment ( Floros et al., 2010 ). Recently, nanosensors are widely applied in the agriculture due to their strengths and fast for environmental monitoring of contamination in the soils and in the water ( Ion et al., 2010 ). Several sensors based on nano-detection technology such as viz. biosensors, electrochemical sensors, optical sensors, and devices will be the main instruments for detecting the heavy metals in trace range ( Ion et al., 2010 ).
Nanomaterials not only directly catalyze degradation of waste and toxic materials but it also aids improve the efficiency of microorganisms in degradation of waste and toxic materials. Bioremediation uses living organisms to break down or remove toxins and harmful substances from agricultural soil and water. In particular, some other terms are also generally used such as bioremediation (beneficial microbes), phytoremediation (plants), and mycoremediation (fungi and mushrooms). Thus, with the bioremediation the heavy metals can be removed from soil and water environmentally and efficiently by microorganisms ( Dixit et al., 2015 ). Therefore, the agricultural bioremediation helps in sustainable remediation technologies to resolve and restore the natural situation of the soil. It is an interesting phenomena in considering the nano–nano interaction to remove the toxic component of the agricultural soil and make it sustainable ( Ion et al., 2010 ; Dixit et al., 2015 ).
Nanofertilizers
In the recent decade nanofertilizers are freely available in the market, but particularly the agricultural fertilizers are still not shaped by the major chemical companies ( Table Table1 1 ). Nanofertilizers may contain nano zinc, silica, iron and titanium dioxide, ZnCdSe/ZnS core shell QDs, InP/ZnS core shell QDs, Mn/ZnSe QDs, gold nanorods, core shell QDs, etc. as well as should endorse control release and improve the its quality. Studies of the uptake, biological fate and toxicity of several metal oxide NPs, viz. Al 2 O 3 , TiO 2 , CeO 2 , FeO, and ZnONPs were carried out intensively in the present decade for agricultural production ( Dimkpa, 2014 ; Zhang et al., 2016 ). The deficiency of zinc has been documented as one of the main problems in limiting agricultural productivity in the alkaline nature of soils ( Sadeghzadeh, 2013 ).
Some commercial product of nanofertilizers.
Commercial product | Content | Company |
---|---|---|
Nano-Gro | Plant growth regulator and immunity enhancer | Agro Nanotechnology Corp., FL, United States |
Nano Green | Extracts of corn, grain, soybeans, potatoes, coconut, and palm | Nano Green Sciences, Inc., India |
Nano-Ag Answer | Microorganism, sea kelp, and mineral electrolyte | Urth Agriculture, CA, United States |
Biozar Nano-Fertilizer | Combination of organic materials, micronutrients, and macromolecules | Fanavar Nano-Pazhoohesh Markazi Company, Iran |
Nano Max NPK Fertilizer | Multiple organic acids chelated with major nutrients, amino acids, organic carbon, organic micro nutrients/trace elements, vitamins, and probiotic | JU Agri Sciences Pvt. Ltd, Janakpuri, New Delhi, India |
Master Nano Chitosan Organic Fertilizer | Water soluble liquid chitosan, organic acid and salicylic acids, phenolic compounds | Pannaraj Intertrade, Thailand |
TAG NANO (NPK, PhoS, Zinc, Cal, etc.) fertilizers | Proteino-lacto-gluconate chelated with micronutrients, vitamins, probiotics, seaweed extracts, humic acid | Tropical Agrosystem India (P) Ltd, India |
Metal oxide NPs are radiolabeled by direct proton bombardment or enriched during synthesis with 18 O to generate 18 F ( Llop et al., 2014 ). Size, degree of aggregation and zeta potential of the metal oxide NPs are studied in the presence of proteins and cell media ( Llop et al., 2014 ; Marzbani et al., 2015 ). Moreover, NP uptake and intracellular fate are followed by ion beam microscopy, transmission electron microscopy, Raman chemical imaging spectroscopy, and confocal laser scanning microscopy ( Marzbani et al., 2015 ). In the future, sustainable bio-based economy that uses eco-efficient bio-processes and renewable bio-resource, will continue decrease and substitute the harmful materials in established applications, and thus it will play a major role (the key strategic challenge) in the development of the technologies desired to address to 21st century ( Prasad et al., 2014 ; Marzbani et al., 2015 ). Accumulation of knowledge in fields of ecology, biology, biodiversity, material science, biotechnology, and engineering opens possibilities to increase biomass productivity as well as to utilize biomass and organic wastes at a highly efficient.
In the present century, the smart agriculture is a way to achieve priority of short and long term development in the countenance of climate change and serves as a link to others ( Helar and Chavan, 2015 ). It seeks to support countries and other functional aspects in securing the necessary agricultural functions ( Kandasamy and Prema, 2015 ). In the past few years, researches related to the expansion of resources in a nanometric extent and their inherent properties are intensively conducted and focused. Practically, when the crystallite size of inorganic materials are reduced to nanoscale, two different phenomena can occur. In the first one (quantum size effect), radical changes of the physical–chemical properties of material are observed. In this case, the performance is totally dependent on the semiconductors-NPs. On the other hand, due to the huge ratio of surface area to volume, NPs exhibit very good transduction properties which are being more interesting for analytical purpose of agricultural products ( Kandasamy and Prema, 2015 ). Nanostructures materials exposed several advantages in logical sciences when used as transducers or as a part of the appreciation in a macro-sized sensing device. In this facts the gold NPs (AuNPs) has its intrinsic properties, and may use as transducers for several improvements of agricultural products. The AuNPs have well-known surface plasmon band that is visible around 520 nm. Moreover, AuNPs have high surface areas and distinctive physicochemical assets that can be easily tuned and thus making them ideal candidates for developing biosensing devices. Additionally, these NPs possess attracted attention in biological studies owing to their low toxicity, biocompatibility and unique optical properties. Biological tests measuring the presence or activity of selected analytics become quicker, more sensitive and flexible when nanoscale particles are put together ( Vidotti et al., 2011 ; Kandasamy and Prema, 2015 ). Thus, application of nanoscale particles results numerous advantages over traditional procedures.
Nanopesticides
The use of nanomaterials in plant protection and production of food is under-explored area in the future. It is well known that insect pests are the predominant ones in the agricultural fields and also in its products, thus NPs may have key role in the control of insect pests and host pathogens ( Khota et al., 2012 ; Table Table2 2 ). The recent development of a nanoencapsulated pesticide formulation has slow releasing properties with enhanced solubility, specificity, permeability and stability ( Bhattacharyya et al., 2016 ). These assets are mainly achieved through either protecting the encapsulated active ingredients from premature degradation or increasing their pest control efficacy for a longer period. Formulation of nanoencapsulated pesticides led to reduce the dosage of pesticides and human beings exposure to them which is environmentally friendly for crop protection ( Nuruzzaman et al., 2016 ). So, development of non-toxic and promising pesticide delivery systems for increasing global food production while reducing the negative environmental impacts to ecosystem ( de Oliveira et al., 2014 ; Kah and Hofmann, 2014 ; Bhattacharyya et al., 2016 ; Grillo et al., 2016 ).
A list of studies on nanopesticides/herbicides and its application.
Carrier system | Agent | Purpose | Method | Reference |
---|---|---|---|---|
Chitosan | Imazapic and Imazapyr | Cytotoxicity assays | Encapsulation | |
Silica | Piracetam, pentoxifylline, and pyridoxine | Perfused brain tissue | Suspension | |
Alginate | Imidacloprid | Cytotoxicity, sucking pest (leafhoppers) | Emulsion | |
Polyacetic acid-polyethylene glycol-polyacetic acid | Imidacloprid | Decrease the lethal concentration | Encapsulation | |
Carboxymethyl chitosan | Methomyl | Control release for longer time-period | Encapsulation | |
Chitosan/tripolyphosphate | Paraquat | Lower cyto- and genotoxicity | Encapsulation | |
Chitosan/tripolyphosphate Chitosan-saponin Chitosan-Cu | Chitosan, saponin, CuSO | Antifungal activity | Cross-linking | |
Xyloglucan/poloxamer | Tropicamide | Have significantly higher corneal permeation across excised goat cornea Less toxic and non-irritant | Encapsulation | |
Wheat gluten | Ethofumesate | Reduce its diffusivity | entrapment/extrusion | |
Alginate | Azadirachtin | Slower release | Encapsulation | |
Surfactants/oil/water | Glyphosate | Increase in bio-efficacy, alleviating the negative effect of pesticide formulations into environment | Emulsion | |
Alginate/chitosan | Paraquat | Increased period of action of the chemical on precise targets, while reducing problems of ecological toxicity | Pre-gelation of alginate then complexation between alginate and chitosan | |
Polyhydroxybutyrate-co-hydroxyvalerate | Atrazine | Decreased genotoxicity and increased biodegradability | Encapsulation | |
Organic-inorganic nanohybrid | 2,4-Dichlorophenoxyacetate | Controlrelease | Self-assembly |
Microencapsulation-like nanoencapsulation is used to develop the quality of products of desired chemicals delivery to the target biological process. Recently, few chemical companies openly promote nanoscale pesticides for sale as “microencapsulated pesticides.” Some products from Syngenta (Switzerland) such as Karate ZEON, Subdue MAXX, Ospray’s Chyella, Penncap-M, and microencapsulated pesticides from BASF may fighting fit for nanoscale ( Gouin, 2004 ). Syngenta also markets in the Australia some products such as the Primo MAXX, Banner MAXX, Subdue MAXX, etc. Despite they are known as microemulsions in the market, however, they are really nanoscale emulsions. It confirms very thin interface between the term of microemulsion and nanoemulsion. This technique is commonly used for formulations of organic NPs ( Gouin, 2004 ) containing active agrochemicals or substances of interest.
Ecotoxicological Implications of the Nanoparticles
The advancement of nanotechnologies has presented significant extents of manufactured NPs into the environment. In order to protect human health and plant from the prospective antagonistic effects of a wide range of nanomaterials, an increasing number of research have focused on the assessment of the toxicity of the NPs normally used in industry ( Yang and Watts, 2005 ; Rana and Kalaichelvan, 2013 ; Du et al., 2017 ; Tripathi et al., 2017a , b , c ). The toxicity of a metal depends upon several factors like solubility, binding specificity to a biological site, and so forth. Metal NPs exhibit antibacterial, anticandidal, and antifungal activities ( Aziz et al., 2016 ; Patra and Baek, 2017 ). Metal NPs exert cytotoxicity depending on the charge at membrane surface, of course, the efficiency of nanotoxic effects of NPs are definitely depending on structure of targeted cell-wall, thus the sensitive order should be mould > yeast > Gram-negative > Gram-positive. Nanotoxicity may be accredited to electrostatic interaction between NPs with membrane and their accumulation in cytoplasm ( Rana and Kalaichelvan, 2011 ; Aziz et al., 2015 , 2016 ).
Several NPs (TiO 2 , ZnO, SiO 2 , and Fullerenes) are photochemically active. When they are exposed to light, the excited electrons are generated that then form superoxide radicals in the presence of oxygen by direct electron transfer ( Hoffmann et al., 2007 ). Thus, this ecotoxicity is surprised in the act when organisms are simultaneously exposed to NPs and UV light (particularly UV light has higher energy than visible light). In this case, the cells respond to oxidative stress by increasing a number of protective enzymatic or genetic constitutions that can easily be measured ( Kovochich et al., 2005 ; Vannini et al., 2014 ), thus generation of reactive oxygen species (ROS) is oxidative stress parameter that can be exploited in determination of the context of toxicity and ecotoxicity. In vitro studies on the toxicity of NPs have confirmed the generation of ROS, for example, by TiO 2 and fullerenes ( Sayes et al., 2004 ), while on other hand, some authors revealed that NPs (fullerenes and silicon NPs) may protect against oxidative stress ( Daroczi et al., 2006 ; Tripathi et al., 2016b , 2017d ; Venkatachalam et al., 2017 ). Much more researches related to interactions between cells and NPs as well as mechanistic facets of NPs metabolism in organisms and specific cells are needed to clarify this dichotomy.
Ecotoxicological research would increasingly attention on the environmental consequence of the materials and complexity of natural systems. Extensive research would be necessary to determine delayed impacts of environmental exposure to NPs and to help determine possible adaptive mechanisms ( Cox et al., 2017 ; Singh et al., 2017 ). More research on bioaccumulation in the food chain and interaction of NPs with other pollutants in the environment. NPs in plants enter cellular system, translocate them shoot and accumulate in various aerial parts, the possibility of their cycling in the ecosystem increases through various trophic levels. After accumulation of NPs effect rate of transpiration, respiration, altering the process of photosynthesis, and interfere with translocation of food material ( Shweta et al., 2016 ; Tripathi et al., 2016b ; Du et al., 2017 ). The degree of toxicity is linked to this surface and to the surface properties of the NPs. The ecotoxicity of NPs is thus very important as it creates a direct link between the adverse effects of NPs and the organisms including microorganisms, plants, and other organisms including humans at various trophic levels ( Rana and Kalaichelvan, 2013 ; Tripathi et al., 2016a ).
Growth of Cultivated Plants and its Ecotoxicological Sustainability
The agriculture host plants take the main part in food chain. Recently, the plants do not only grow on agricultural lands, but they are also developed on aqueous medium too. Naturally, several NPs of iron oxide (magnetite), a magnetic form of iron ore can deposit in the plant host. It is interesting to propose that the iron (II, III) oxide NPs (Fe 3 O 4 -NPs) have the ability to accumulate in Lepidium sativum and Pisum sativum plants. Therefore, this type of observation clearly proposes that the roles to mention NPs are present in the natural ecosystem ( Bystrzejewska-Piotrowska et al., 2012 ; Abbas et al., 2016 ). Moreover, the uses of polymeric NPs in the agricultural field, especially loaded with insecticides of plant origin are unique and increasingly permeated ( Chakravarthy et al., 2012 ; Perlatti et al., 2013 ). No doubt that microorganism plays crucial role in maintaining soil health, ecosystem, and crop productivity ( Mishra and Kumar, 2009 ). Therefore, it is very essential to know the ecotoxicological aspects of the considered agricultural field. If nanomaterials containing agricultural plants are devoid of any toxic nanocomposite, then the unique possibility of more production of agricultural crops. Thus, the introduction of engineered (either chemical or green) NPs in the agricultural field should always be a routine check-up to sustain an eco-friendly in the agricultural field ( Figure Figure3 3 ).

Potential applications of nanotechnology representing the consequences of nanoparticles in sustainable agriculture.
Nanobiosensors
Many advantages of physical–chemical properties of nanoscale materials are also exploitable in field of biosensors development of biosensors. Sagadevan and Periasamy (2014) stated that the sensitivity and performance of biosensors can be improved by using nanomaterials through new signal transduction technologies. The tremendous advancement in the nanobiosensors are due to the great technological demand for rapid, sensitive and cost-effective nanobiosensor systems in vital areas of human activity such as health care, agriculture, genome analysis, food and drink, the process industries, environmental monitoring, defense, and security. At present, the nanotechnology-based biosensors are at the early stage of development ( Fogel and Limson, 2016 ). The improvement of tools and procedures used to fabricate, measure and image nanoscale objects, has led to the development of sensors. The nanomaterials such as metal (gold, silver, cobalt, etc.) NPs, CNT, magnetic NPs, and QDs have been actively investigated for their applications in biosensors which have become a new interdisciplinary frontier between biological detection and material science. Thus a biosensor is a device that combines a biological recognition element with physical or chemical principles. It integrates a biological one with an electronic component to yield a measurable signal component, and the biological recognition is through the transducer process and the signal processing through electronic achievement. The higher specificity and sensitivity of biosensor systems over the conventional methods are due to the presence of the bioreceptor (biological element) that is combined with a suitable transducer which produces a signal after interaction with the target molecule of interest. Recently, different natural and artificial bioreceptors are developed and applied such as enzymes, dendrimers, thin films, etc. Therefore, by biosensor, an analytical device, this converts a biological response into an electrical signal. It is concerned with these parts of biological elements like, an antibody, an enzyme, a protein, or a nucleic acid. The transducer and the associated electronics or signal processors that are primarily responsible for detection of the functions ( Rai et al., 2012 ). The micro cantilever-based DNA biosensor that uses AuNPs have been developed and used widely to detect low level DNA concentration during a hybridization reaction ( Brolo, 2012 ).
Nanotechnologies in Food Industry
Nanoscale biosensors can take part in pathogen detection and diagnosis. Nanotechnology has the ability to supply bioactive ingredients in foodstuffs to hosts while improvement of knowledge of food materials at the nanoscale ( Martirosyan and Schneider, 2014 ). It also helps in nanoscale filtration systems for improved texture modification of food. Nano biosensors interact with food, attractive surface and thus maintain the glaziers and colors of food, magnetic nanocomposite for tag sensors. Nanoprinted, intelligent packaging, controlled release ( Ghaani et al., 2016 ), nano-additives ( Khond and Kriplani, 2016 ), nanocoding of plastics and paper materials ( Bhushani and Anandharamakrishnan, 2014 ), for authentication and identification purposes ( Khond and Kriplani, 2016 ; Savina et al., 2016 ). In food quality tests, some important aspects should be covered such as sensing ability of label and package, in situ sensors, food quality monitoring (e.g., color, smell, taste, texture), control and nutraceuticals delivery, portable DNA/protein chips, etc. Most of the time, nanomaterials produced by bottom-up methods ( Siegrist et al., 2008 ).
Food Process
Recently nanotechnology is widely applied in food processing such as nanocarrier systems for delivery of nutrients and supplements, organic nano-sized additives for food, supplements, and animal feed. Many food products already contain NP naturally. Milk contains casein, a form of milk protein present at the nanoscale or meat is made up of protein filaments that are also be classified into nanomaterial group. The texture and properties of these products are determined by the organization and structures of proteins inside.
Recently, some nutrients mainly vitamins are encapsulated and delivered into the bloodstream through digestion system with very high efficiency. Some foods and drinks were fortified with these NPs without affecting the taste or appearance. NP emulsions are being used in ice cream and spreads of this nanoemulsion can improve the texture and uniformity of the ice cream ( Berekaa, 2015 ). A real example: KD Pharma BEXBACH GMBH (Germany) provides encapsulated Omega-3 fatty acids in two different forms—suspension and powder. The capsulation technology used the resulted particles in nano- and microscale.
Food Packaging and Labeling
In the food industry, maintaining some important factors such as quality, safe, freshness, taste, etc. whole supply chain requires producers to packaging and labeling their products. Development of smart packages that can provide useful information is still big challenge for researchers and producers. Recently, some packaging materials incorporated with “nanosensors” to detect the oxidation process in food have been produced and used in food industry. Working scheme is quite simple: when the oxidation occurs in the food package, NP-based sensors indicate the color change and information about the nature of the packed foods can be observed. This technology have been successfully applied in package of milk and meat ( Bumbudsanpharoke and Ko, 2015 ).
Due to nanostructure, NPs are good barriers for diffusion gases such as oxygen, carbon dioxide, thus it can be exploited in food packaging. Some drinks (beer, soda waters, etc.) naturally have to keep appreciate amount of carbon dioxide can be packaged in the bottles made with nanocomposites because of minimization of CO 2 lost, decrease in weight of packaging materials, increase in shelf life, etc. Other exploitation way is the incorporation of NPs in packaging and this technology will slow down some biochemical processes such as oxidation, degradation, etc. thus it help to extend the shelf-life of food products. In the food packaging industry, the most used materials are plastic polymers that can be incorporated or coated with nanomaterials for improved mechanical or functional properties ( Berekaa, 2015 ). Moreover, nanocoatings on food contact surfaces act as barrier or antimicrobial properties. Silver NPs have been successfully embedded in the plastic for making food storage bins, and this acts like disinfection of bins, thus minimizing harmful bacterial growth. Therefore, the nanotechnology is a forward looking process, it acts as an agricultural biosecurity ( Bumbudsanpharoke and Ko, 2015 ).
NPs in precise have revealed broad-spectrum antibacterial properties against both Gram-positive and Gram-negative bacteria. ZnO NPs were found to inhibit Staphylococcus aureus ( Liu et al., 2009 ) and AgNPs exhibit concentration-dependent antimicrobial activity against Escherichia coli, Aeromonas hydrophila , and Klebsiella pneumoniae ( Aziz et al., 2016 ). The antimicrobial mechanism of action of NPs is typically considered as of few prototypes such as oxidative stress and cell damage, metal ion release, or non-oxidative mechanisms ( Wang et al., 2017 ). These mechanisms can happen concurrently. Firm studies have suggested that Ag NPs quick neutralization of the surface electric charge of the bacterial membrane and change its permeability, ultimately leading to apoptosis. Moreover, the generation of ROS prevents the antioxidant defense system and causes physiochemical damage to the intrinsic cell membrane. According to current investigation, the major processes causal the antibacterial effects of NPs are as follows: disruption of the bacterial cell membrane; generation of ROS; penetration of the bacterial cell membrane by passive or facilitated diffusion and induction of intracellular antibacterial effects, including interfaces with DNA replication, and inhibition of protein synthesis ( Aziz et al., 2015 ; Wang et al., 2017 ).
Nanosensors help in food labeling and in combination with NP-based intelligent inks or reactive nanolayers may provide smart recognition of relevant food product. Printed labels in the food package that can indicate the following highlights: temperature, time, pathogens, freshness, humidity, etc. Nanobarcode particles with different patterns of gold which can form template and also with silver stripes are possible to synthesize itself. Lastly, we can suggest that the contaminant or nutrient sorption on NPs surfaces has attracted the attention of researchers for more studies on soil chemistry, showing that NPs have high sorption capacities for metal and anionic contaminants ( Li et al., 2016 ). It was found that the contaminant sequestration was accomplished mainly by surface complication. It is likely that the sorbet surface species can be encapsulated within interior surfaces of NPs. A phenomenon with significant consequences for contaminant dispersion or remediation processes can exhibit. Moreover, metallic species as Ni can be linked to natural short-ordered aluminosilicates, TiO 2 surfaces, humic acids, and aromatic compounds by MWCNTs and these association may be considered as very potent bioremediation in nano agricultural system ( Raliya et al., 2013 ; Hajirostamlo et al., 2015 ).
Future Perspectives
Sustainable agriculture must be taken as an ecosystem method, where abiotic–biotic-living beings live in accord with a co-ordinated stability of food chains and their related energy balances. New technologies, modernization, increased in use of nano-chemicals, specialization and government policies are adapted to maximize the production in agriculture. To overcome the situation, it is mandatory to establish the recent technology in the food industry. Therefore, the new and future technology is nanotechnology that possesses very unique property in food supply chain (from the field to table: crop production, use of agro-chemicals such as nanofertilizers, nanopesticides, nanoherbicides, etc., precision farming techniques, intelligent feed, enhancement of food texture and quality, and bioavailability/nutrient values, packaging and labeling, etc.) round the world agricultural sector. Some focused areas may need more attention in near future researches in the field of agricultural nanotechnology or nanofoods:
- simple • New environmental and safety delivery systems for carrying special food/feed compounds, plant nutrients, etc. These systems also can have pharmaceutical application potentials.
- simple • The (bio)sensors related nanotechnology have effective role in insect pest control and food products of agriculture. Consumers always can get actual information of the state of certain food product via intelligent food packaging corporated with nanosensors.
- simple • The properties of nanomaterials such as size, dose, exposure time, surface chemistry, structures, immune response, accumulation, retention time, etc., and other effects should be accessed carefully. New analytical methods are needed to develop to detect, validate and access the effects of each nanomaterials/nanofoods in whole ecosystems. Life-cycle analysis of nanomaterials/nanofoods should be done. Improvement of wide-ranging databank as well as international collaboration for policy, idea and regulation are needed for manipulation of this knowledge. Additionally, the authorities should provide clear guidelines and roadmaps for reducing risks of the use of nanotechnological products.
- simple • New communication channels and debates should be opened with participation of different sides such as consumers, researchers, authorities, industrial sectors, etc. to discuss impacts of this technology in human life, economy, and science.
This technology in the long term may provide innovative and economical development routes for human nutrition worldwide.
Agriculture which is the only provider of human’s food that should produce from transitional and final inputs with well-known technologies. Thus, it is necessary to take a modern knowledge in agriculture. In spite of being relative advantages in agriculture process, still developing countries are suffering from lack of high importance of food products. Despite a lot of information about individual nanomaterials are available, but toxicity level of many NPs is still indefinable, thus the application of these materials is limited due to the lack of knowledge of risk assessments and effects on human health. Development of comprehensive database and alarm system, as well as international cooperation for regulation and legislation are necessary for exploitation of this technology.
Author Contributions
RP, AB, and QN developed the idea and wrote the manuscript. All authors proofread and approved the final manuscript.
Conflict of Interest Statement
The authors declare that the research was conducted in the absence of any commercial or financial relationships that could be construed as a potential conflict of interest.
1 patentscope.wipo.int (accessed date: March 15, 2017).
2 hkc22.com
- Abbas S. S., Haneef M., Lohani M., Tabassum H., Khan A. F. (2016). Nanomaterials used as a plants growth enhancer: an update. Int. J. Pharm. Sci. Rev. Res. 5 17–23. [ Google Scholar ]
- Androvitsaneas P., Young A. B., Schneider C., Maier S., Kamp M., Höfling S., et al. (2016). Charged quantum dot micropillar system for deterministic light-matter interactions. Phys. Rev. B 93 : 241409 10.1103/physrevb.93.241409 [ CrossRef ] [ Google Scholar ]
- Anton N., Vandamme T. F. (2009). The universality of low-energy nano- emulsification. Int. J. Pharm. 377 142–147. 10.1016/j.ijpharm.2009.05.014 [ PubMed ] [ CrossRef ] [ Google Scholar ]
- Anton N., Vandamme T. F. (2011). Nano-emulsions and micro-emulsions: clarifications of the critical differences. Pharm. Res. 28 978–985. 10.1007/s11095-010-0309-1 [ PubMed ] [ CrossRef ] [ Google Scholar ]
- Asua J. M. (2002). Miniemulsion polymerization. Prog. Polym. Sci. 27 1283–1346. 10.1016/S0079-6700(02)00010-2 [ CrossRef ] [ Google Scholar ]
- Aziz N., Faraz M., Pandey R., Sakir M., Fatma T., Varma A., et al. (2015). Facile algae-derived route to biogenic silver nanoparticles: synthesis, antibacterial and photocatalytic properties. Langmuir 31 11605–11612. 10.1021/acs.langmuir.5b03081 [ PubMed ] [ CrossRef ] [ Google Scholar ]
- Aziz N., Pandey R., Barman I., Prasad R. (2016). Leveraging the attributes of Mucor hiemalis-derived silver nanoparticles for a synergistic broad-spectrum antimicrobial platform. Front. Microbiol. 7 : 1984 10.3389/fmicb.2016.01984 [ PMC free article ] [ PubMed ] [ CrossRef ] [ Google Scholar ]
- Bakalova R., Zhelev Z., Ohba H., Ishikawa M., Baba Y. (2004). Quantum dots as photosensitizers? Nat. Biotechnol. 22 1360–1361. 10.1038/nbt1104-1360 [ PubMed ] [ CrossRef ] [ Google Scholar ]
- Berekaa M. M. (2015). Nanotechnology in food industry; advances in food processing, packaging and food Safety. Int. J. Curr. Microbiol. App. Sci. 4 345–357. [ Google Scholar ]
- Bhattacharyya A., Duraisamy P., Govindarajan M., Buhroo A. A., Prasad R. (2016). “Nano-biofungicides: emerging trend in insect pest control,” in Advances and Applications through Fungal Nanobiotechnology ed. Prasad R. (Cham: Springer International Publishing; ) 307–319. 10.1007/978-3-319-42990-8_15 [ CrossRef ] [ Google Scholar ]
- Bhushani J. A., Anandharamakrishnan C. (2014). Electrospinning and electrospraying techniques: potential food based applications. Trends Food Sci. Technol. 38 21–33. 10.1016/j.tifs.2014.03.004 [ CrossRef ] [ Google Scholar ]
- Bonnell D. A., Huey B. D. (2001). “Basic principles of scanning probe microscopy,” in Scanning Probe Microscopy and Spectroscopy: Theory, Techniques, and Applications ed. Bonnell D. A. (New York, NY: Wiley-VCH; ). [ Google Scholar ]
- Brolo A. G. (2012). Plasmonics for future biosensors. Nat. Photonics 6 709–713. 10.1038/nphoton.2012.266 [ CrossRef ] [ Google Scholar ]
- Bulovic V., Mandell A., Perlman A. (2004). Molecular Memory Device. US 20050116256 A1 . [ Google Scholar ]
- Bumbudsanpharoke N., Ko S. (2015). Nano-food packaging: an overview of market, migration research, and safety regulations. J. Food Sci. 80 R910–R923. 10.1111/1750-3841.12861 [ PubMed ] [ CrossRef ] [ Google Scholar ]
- Bystrzejewska-Piotrowska G., Asztemborska M., Steborowski R., Polkowska-Motrenko H., Danko J., Ryniewicz B. (2012). Application of neutron activation for investigation of Fe3O4 nanoparticles accumulation by plants. Nukleonika 57 427–430. [ Google Scholar ]
- Camilli L., Pisani C., Gautron E., Scarselli M., Castrucci P., D’Orazio F., et al. (2014). A three-dimensional carbon nanotube network for water treatment. Nanotechnology 25 : 065701 10.1088/0957-4484/25/6/065701 [ PubMed ] [ CrossRef ] [ Google Scholar ]
- Campbell B. M., Thornton P., Zougmoré R., van Asten P., Lipper L. (2014). Sustainable intensification: what is its role in climate smart agriculture? Curr. Opin. Environ. Sustain. 8 39–43. 10.1016/j.cosust.2014.07.002 [ CrossRef ] [ Google Scholar ]
- Chahine N. O., Collette N. M., Thomas B. C., Genetos D. C., Loots G. G. (2014). Nanocomposite scaffold for chondrocyte growth and cartilage tissue engineering: effects of carbon nanotube surface functionalization. Tissue Eng. Part A. 20 2305–2315. 10.1089/ten.TEA.2013.0328 [ PMC free article ] [ PubMed ] [ CrossRef ] [ Google Scholar ]
- Chakravarthy A. K., Bhattacharyya A., Shashank P. R., Epidi T. T., Doddabasappa B., Mandal S. K. (2012). DNA-tagged nano gold: a new tool for the control of the armyworm, Spodoptera litura Fab. (Lepidoptera: Noctuidae). Afr. J. Biotechnol. 11 9295–9301. 10.5897/AJB11.883 [ CrossRef ] [ Google Scholar ]
- Chevillard A., Angellier-Coussy H., Guillard V., Gontard N., Gastaldi E. (2012). Controlling pesticide release via structuring agropolymer and nanoclays based materials. J. Hazard. Mater. 20 32–39. 10.1016/j.jhazmat.2011.11.093 [ PubMed ] [ CrossRef ] [ Google Scholar ]
- Couvreur P., Dubernet C., Puisieux F. (1995). Controlled drug delivery with nanoparticles: current possibilities and future trends. Eur. J. Pharm. Biopharm. 41 2–13. [ Google Scholar ]
- Cox A., Venkatachalam P., Sahi S., Sharma N. (2017). Reprint of: silver and titanium dioxide nanoparticle toxicity in plants: a review of current research. Plant Physiol. Biochem. 110 33–49. 10.1016/j.plaphy.2016.08.007 [ PubMed ] [ CrossRef ] [ Google Scholar ]
- Daroczi B., Kari G., McAleer M. F., Wolf J. C., Rodeck U., Dicker A. P. (2006). In vivo radioprotection by the fullerene nanoparticle DF-1 as assessed in a zebra fish model. Clin. Cancer Res. 12 7086–7091. 10.1158/1078-0432.CCR-06-0514 [ PubMed ] [ CrossRef ] [ Google Scholar ]
- Das S., Wolfson B. P., Tetard L., Tharkur J., Bazata J., Santra S. (2015). Effect of N-acetyl cysteine coated CdS:Mn/ZnS quantum dots on seed germination and seedling growth of snow pea ( Pisum sativum L.): imaging and spectroscopic studies. Environ. Sci. 2 203–212. 10.1039/c4en00198b [ CrossRef ] [ Google Scholar ]
- de Oliveira J. L., Campos E. V., Bakshi M., Abhilash P. C., Fraceto L. F. (2014). Application of nanotechnology for the encapsulation of botanical insecticides for sustainable agriculture: prospects and promises. Biotechnol. Adv. 32 1550–1561. 10.1016/j.biotechadv.2014.10.010 [ PubMed ] [ CrossRef ] [ Google Scholar ]
- Dilbaghi N., Kaur H., Ahuja M., Kumar S. (2013). Evaluation of tropicamide-loaded tamarind seed xyloglucan nanoaggregates for ophthalmic delivery. Carbohydr. Polym. 94 286–291. 10.1016/j.carbpol.2013.01.054 [ PubMed ] [ CrossRef ] [ Google Scholar ]
- Dimkpa C. O. (2014). Can nanotechnology deliver the promised benefits without negatively impacting soil microbial life? J. Basic Microbiol. 54 889–904. 10.1002/jobm.201400298 [ PubMed ] [ CrossRef ] [ Google Scholar ]
- Dixit R., Wasiullah, Malaviya D., Pandiyan K., Singh U. B., Sahu A.et al. (2015). Bioremediation of heavy metals from soil and aquatic environment: An overview of principles and criteria of fundamental processes. Sustainability 7 2189–2212. 10.3390/su7022189 [ CrossRef ] [ Google Scholar ]
- Du W., Tan W., Peralta-Videa J. R., Gardea-Torresdey J. L., Ji R., Yin Y., et al. (2017). Interaction of metal oxide nanoparticles with higher terrestrial plants: Physiological and biochemical aspects. Plant Physiol. Biochem. 110 210–225. 10.1016/j.plaphy.2016.04.024 [ PubMed ] [ CrossRef ] [ Google Scholar ]
- Ezhilarasi P. N., Karthik P., Chhanwal N., Anandharamakrishnan C. (2012). Nanoencapsulation techniques for food bioactive components: a Review. Food Bioprocess Technol. 6 628–647. 10.1007/s11947-012-0944-0 [ CrossRef ] [ Google Scholar ]
- Feynman R. P. (1996). No Ordinary Genius: The Illustrated Richard Feynman . New York, NY: W.W. Norton & Company. [ Google Scholar ]
- Floros J. D., Newsome R., Fisher W., Barbosa-Cánovas G. V., Chen H., Dunne C. P., et al. (2010). Feeding the world today and tomorrow: the importance of food science and technology. Compr. Rev. Food Sci. Food Saf. 9 572–599. 10.1111/j.1541-4337.2010.00127.x [ PubMed ] [ CrossRef ] [ Google Scholar ]
- Fogel R., Limson J. (2016). Developing biosensors in developing countries: South Africa as a case study. Biosensors 6 : 5 10.3390/bios6010005 [ PMC free article ] [ PubMed ] [ CrossRef ] [ Google Scholar ]
- Fraceto L. F., Grillo R., de Medeiros G. A., Scognamiglio V., Rea G., Bartolucci C. (2016). Nanotechnology in agriculture: which innovation potential does it have? Front. Environ. Sci. 4 : 20 10.3389/fenvs.2016.00020 [ CrossRef ] [ Google Scholar ]
- Ghaani M., Cozzolino C. A., Castelli G., Farris S. (2016). An overview of the intelligent packaging technologies in the food sector. Trends Food Sci. Tech. 51 1–11. 10.1016/j.tifs.2016.02.008 [ CrossRef ] [ Google Scholar ]
- Gibney E. (2015). Buckyballs in space solve 100-year-old riddle. Nature News 10.1038/nature.2015.17987 [ CrossRef ] [ Google Scholar ]
- Gouin S. (2004). Microencapsulation: industrial appraisal of existing technologies and trends. Trends Food Sci. Technol. 15 330–347. 10.1038/nature.2015.17987 [ CrossRef ] [ Google Scholar ]
- Grillo R., Abhilash P. C., Fraceto L. F. (2016). Nanotechnology applied to bio-encapsulation of pesticides. J. Nanosci. Nanotechnol. 16 1231–1234. 10.1016/j.tifs.2003.10.005 [ PubMed ] [ CrossRef ] [ Google Scholar ]
- Grillo R., Melo N. F. S., de Lima R., Lourenço R. W., Rosa A. H., Fraceto L. F. (2010). Characterization of atrazine-loaded biodegradable poly(hydroxybutyrate-co-hydroxyvalerate) microspheres. J. Polym. Environ. 18 26–32. 10.1166/jnn.2016.12332 [ CrossRef ] [ Google Scholar ]
- Grillo R., Pereira A. E., Nishisaka C. S., de Lima R., Oehlke K., Greiner R., et al. (2014). Chitosan/tripolyphosphate nanoparticles loaded with paraquat herbicide: an environmentally safer alternative for weed control. J. Hazard. Mater. 27 163–171. 10.1016/j.jhazmat.2014.05.079 [ PubMed ] [ CrossRef ] [ Google Scholar ]
- Gruère G. P. (2012). Implications of nanotechnology growth in food and agriculture in OECD countries. Food Policy 37 191–198. 10.1016/j.jhazmat.2014.05.079 [ CrossRef ] [ Google Scholar ]
- Gupta A., Eral H. B., Hatton T. A., Doyle P. S. (2016). Nanoemulsions: formation, properties and applications. Soft Matter 12 2826–2841. 10.1039/e5sm02958a [ PubMed ] [ CrossRef ] [ Google Scholar ]
- Gutiérrez J. M., González C., Maestro A., Solè I., Pey C. M., Nolla J. (2008). Nano-emulsions: new applications and optimization of their preparation. Curr. Opin. Colloid Interface Sci. 13 245–251. 10.1039/C5SM02958A [ CrossRef ] [ Google Scholar ]
- Hajirostamlo B., Mirsaeedghazi N., Arefnia M., Shariati M. A., Fard E. A. (2015). The role of research and development in agriculture and its dependent concepts in agriculture [Short Review]. Asian J. Appl. Sci. Eng. 4 10.1016/j.cocis.2008.01.005 [ CrossRef ] [ Google Scholar ]
- Haolong L., Yang Y., Yizhan W., Chunyu W., Wen L., Lixin W. (2011). Self-assembly and ion-trapping properties of inorganic nanocapsule-surfactant hybrid spheres. Soft Matter 7 2668–2673. 10.1039/c0sm01044h [ CrossRef ] [ Google Scholar ]
- Helar G., Chavan A. (2015). Synthesis, characterization and stability of gold nanoparticles using the fungus Fusarium oxysporum and its impact on seed. Int. J. Recent Sci. Res. 6 3181–3318. [ Google Scholar ]
- Hildebrand G. E., Tack J. W. (2000). Microencapsulation of peptides and proteins. Int. J. Pharm. 196 173–176. 10.1016/S0378-5173(99)00415-9 [ PubMed ] [ CrossRef ] [ Google Scholar ]
- Hoffmann M., Holtze E. M., Wiesner M. R. (2007). “Reactive oxygen species generation on nanoparticulate material,” in Environmental Nanotechnology. Applications and Impacts of Nanomaterials eds Wiesner M. R., Bottero J. Y. (New York, NY: McGraw Hill; ) 155–203. [ Google Scholar ]
- Hu Y., Li J., Ma L., Peng Q., Feng W., Zhang L., et al. (2010). High efficiency transport of quantum dots into plant roots with the aid of silwet L-77. Plant Physiol. Biochem. 48 703–709. 10.1016/j.plaphy.2010.04.001 [ PubMed ] [ CrossRef ] [ Google Scholar ]
- Hussein M. Z., Yahaya A. H., Zainal Z., Kian L. H. (2005). Nanocomposite-based controlled release formulation of an herbicide, 2,4-dichlorophenoxyacetate incapsulated in zinc-aluminium-layered double hydroxide. Sci. Technol. Adv. Mater. 6 956–962. 10.1016/j.stam.2005.09.004 [ CrossRef ] [ Google Scholar ]
- Ion A. C., Ion I., Culetu A. (2010). Carbon-based nanomaterials: Environmental applications. Univ. Politehn. Bucharest 38 129–132. [ Google Scholar ]
- Jampilek J., Zaruba K., Oravec M., Kunes M., Babula P., Ulbrich P., et al. (2015). Preparation of silica nanoparticles loaded with nootropics and their in vivo permeation through blood-brain barrier. BioMed Res. Int. 2015 : 812673 10.1155/2015/812673 [ PMC free article ] [ PubMed ] [ CrossRef ] [ Google Scholar ]
- Jerobin J., Sureshkumar R. S., Anjali C. H., Mukherjee A., Chandrasekaran N. (2012). Biodegradable polymer based encapsulation of neem oil nanoemulsion for controlled release of Aza-A. Carbohydr. Polym. 90 1750–1756. 10.1016/j.carbpol.2012.07.064 [ PubMed ] [ CrossRef ] [ Google Scholar ]
- Jiang L. C., Basri M., Omar D., Rahman M. B. A., Salleh A. B., Zaliha R. N., et al. (2012). Green nano-emulsion intervention for water-soluble glyphosate isopropylamine (IPA) formulations in controlling Eleusine indica ( E. indica ). Pest. Biochem. Physiol. 102 19–29. 10.1016/j.pestbp.2011.10.004 [ CrossRef ] [ Google Scholar ]
- Johnston B. F., Mellor J. W. (1961). The role of agriculture in economic development. Am. Econ. Rev. 51 566–593. [ Google Scholar ]
- Kah M. (2015). Nanopesticides and nanofertilizers: emerging contaminants or opportunities for risk mitigation? Front. Chem. 3 : 64 10.3389/fchem.2015.00064 [ PMC free article ] [ PubMed ] [ CrossRef ] [ Google Scholar ]
- Kah M., Hofmann T. (2014). Nanopesticides research: current trends and future priorities. Environ. Int. 63 224–235. 10.1016/j.envint.2013.11.015 [ PubMed ] [ CrossRef ] [ Google Scholar ]
- Kandasamy S., Prema R. S. (2015). Methods of synthesis of nano particles and its applications. J. Chem. Pharm. Res. 7 278–285. [ Google Scholar ]
- Khond V. W., Kriplani V. M. (2016). Effect of nanofluid additives on performances and emissions of emulsified diesel and biodiesel fueled stationary CI engine: a comprehensive review. Renew. Sustain. Energy Rev. 59 1338–1348. 10.1016/j.rser.2016.01.051 [ CrossRef ] [ Google Scholar ]
- Khota L. R., Sankarana S., Majaa J. M., Ehsania R., Schuster E. W. (2012). Applications of nanomaterials in agricultural production and crop protection: a review. Crop Prot. 35 64–70. 10.1016/j.cropro.2012.01.007 [ CrossRef ] [ Google Scholar ]
- Konstantatos G., Sargent E. H. (2009). Solution-processed quantum dot photodetectors. Proc. IEEE 97 1666–1683. 10.1109/JPROC.2009.2025612 [ CrossRef ] [ Google Scholar ]
- Kovochich M., Xia T., Xu J., Yeh J. I., Nel A. E. (2005). Principles and procedures to assess nanoparticles. Environ. Sci. Technol. 39 1250–1256. [ Google Scholar ]
- Kumar S., Bhanjana G., Sharma A., Sidhu M. C., Dilbaghi N. (2014). Synthesis, characterization and on field evaluation of pesticide loaded sodium alginate nanoparticles. Carbohydr. Polym. 101 1061–1067. 10.1016/j.carbpol.2013.10.025 [ PubMed ] [ CrossRef ] [ Google Scholar ]
- Li H., Shan C., Zhang Y., Cai J., Zhang W., Pan B. (2016). Arsenate adsorption by hydrous ferric oxide nanoparticles embedded in cross-linked anion exchanger: effect of the host pore structure. ACS Appl. Mater. Interfaces 8 3012–3020. 10.1021/acsami.5b09832 [ PubMed ] [ CrossRef ] [ Google Scholar ]
- Liu R., Lal R. (2015). Potentials of engineered nanoparticles as fertilizers for increasing agronomic productions. Sci. Total Environ. 514 131–139. 10.1016/j.scitotenv.2015.01.104 [ PubMed ] [ CrossRef ] [ Google Scholar ]
- Liu Y., He L., Mustapha A., Li H., Hu Z. Q., Lin M. (2009). Antibacterial activities of zinc oxide nanoparticles against Escherichia coli O157:H7. J. Appl. Microbiol. 107 1193–1201. 10.1111/j.1365-2672.2009.04303.x [ PubMed ] [ CrossRef ] [ Google Scholar ]
- Llop J., Estrela-Lopis I., Ziolo R. F., González A., Fleddermann J., Dorn M., et al. (2014). Uptake, biological fate, and toxicity of metal oxide nanoparticles. Part. Part. Syst. Charact. 31 24–35. 10.1002/ppsc.201300323 [ CrossRef ] [ Google Scholar ]
- Maruyama C. R., Guilger M., Pascoli M., Bileshy-José N., Abhilash P. C., Fraceto L. F., et al. (2016). Nanoparticles based on chitosan as carriers for the combined herbicides imazapic and imazapyr. Sci. Rep. 6 : 23854 10.1038/srep23854. [ PMC free article ] [ PubMed ] [ CrossRef ] [ Google Scholar ]
- Martirosyan A., Schneider Y. J. (2014). Engineered nanomaterials in food: implications for food safety and consumer health . Int. J. Environ. Res. Public Health 11 5720–5750. 10.3390/ijerph110605720 [ PMC free article ] [ PubMed ] [ CrossRef ] [ Google Scholar ]
- Marzbani P., Afrouzi Y. M., Omidvar A. (2015). The effect of nano-zinc oxide on particleboard decay resistance. Maderas Cienc. Tecnol. 17 63–68. 10.4067/s0718-221x2015005000007 [ CrossRef ] [ Google Scholar ]
- Mason T. G., Wilking J. N., Meleson K., Chang C. B., Graves S. M. (2006). Nanoemulsions: formation, structure, and physical properties. J. Phys. Condens. Matt. 18 R635–R666. 10.1088/0953-8984/18/41/r01 [ CrossRef ] [ Google Scholar ]
- Memarizadeh N., Ghadamyari M., Adeli M., Talebi K. (2014). Preparation, characterization and efficiency of nanoencapsulated imidacloprid under laboratory conditions. Ecotoxicol. Environ. Saf. 107 77–83. 10.1016/j.ecoenv.2014.05.009 [ PubMed ] [ CrossRef ] [ Google Scholar ]
- Mishra V. K., Kumar A. (2009). Impact of metal nanoparticles on the plant growth promoting rhizobacteria. Dig. J. Nanomater. Biostruct. 4 587–592. [ Google Scholar ]
- Mukhopadhyay S. S. (2014). Nanotechnology in agriculture: prospects and constraints. Nanotechnol. Sci. Appl. 7 63–71. 10.2147/NSA.S39409 [ PMC free article ] [ PubMed ] [ CrossRef ] [ Google Scholar ]
- Nima A. Z., Lahiani M. H., Watanabe F., Xu Y., Khodakovskaya M. V., Biris A. S. (2014). Plasmonically active nanorods for delivery of bio-active agents and high-sensitivity SERS detection in planta. RSC Adv. 4 64985–64993. 10.1039/C4RA10358K [ CrossRef ] [ Google Scholar ]
- Nuruzzaman M., Rahman M. M., Liu Y., Naidu R. (2016). Nanoencapsulation, nano-guard for pesticides: a new window for safe application. J. Agric. Food Chem. 64 1447–1483. 10.1021/acs.jafc.5b05214 [ PubMed ] [ CrossRef ] [ Google Scholar ]
- Ozdemir M., Kemerli T. (2016). “Innovative applications of micro and nanoencapsulation in food packaging,” in Encapsulation and Controlled Release Technologies in Food Systems ed. Lakkis J. M. (Chichester: John Wiley & Sons, Ltd; ). [ Google Scholar ]
- Patra J. K., Baek K.-H. (2017). Antibacterial activity and synergistic antibacterial potential of biosynthesized silver nanoparticles against foodborne pathogenic bacteria along with its anticandidal and antioxidant effects. Front. Microbiol. 8 : 167 10.3389/fmicb.2017.00167 [ PMC free article ] [ PubMed ] [ CrossRef ] [ Google Scholar ]
- Perlatti B., Bergo P. L. S., Silva M. F. G., Fernandes J. B., Forim M. R. (2013). “Polymeric nanoparticle-based insecticides: a controlled release purpose for agrochemicals,” in: Insecticides-Development of Safer and More Effective Technologies ed. Trdan S. (Rijeka: InTech; ) 523–550. 10.5772/53355 [ CrossRef ] [ Google Scholar ]
- Pohlmann R., Beck R. C. R., Lionzo M. I. Z., Coasta T. M. H., Benvenutti E. V., Re M. I., et al. (2008). Surface morphology of spray-dried nanoparticle-coated microparticles designed as an oral drug delivery system. Braz. J. Chem. Eng. 25 389–398 [ Google Scholar ]
- Pokropivny V., Lohmus R., Hussainova I., Pokropivny A., Vlassov S. (2007). Introduction to Nanomaterials and Nanotechnology. Tartu: University of Tartu; 225 . [ Google Scholar ]
- Porter A. E., Gass M., Muller K., Skepper J. N., Midgley P. A., Welland M. (2007). Direct imaging of single-walled carbon nanotubes in cells. Nat. Nanotechnol. 2 713–717. 10.1038/nnano.2007.347 [ PubMed ] [ CrossRef ] [ Google Scholar ]
- Prasad R. (2014). Synthesis of silver nanoparticles in photosynthetic plants. J. Nanopart. 2014 : 963961 10.1155/2014/963961 [ CrossRef ] [ Google Scholar ]
- Prasad R., Kumar V., Prasad K. S. (2014). Nanotechnology in sustainable agriculture: present concerns and future aspects. Afr. J. Biotechnol. 13 705–713. 10.5897/AJBX2013.13554 [ CrossRef ] [ Google Scholar ]
- Prasad R., Pandey R., Barman I. (2016). Engineering tailored nanoparticles with microbes: quo vadis. WIREs Nanomed. Nanobiotechnol. 8 316–330. 10.1002/wnan.1363 [ PubMed ] [ CrossRef ] [ Google Scholar ]
- Puglisi G., Fresta M., Giammona G., Ventura C. A. (1995). Influence of the preparation conditions on poly (ethylcyanoacrylate) nanocapsule formation. Int. J. Pharm. 125 283–287. 10.1016/0378-5173(95)00142-6 [ CrossRef ] [ Google Scholar ]
- Pyrzynska K. (2011). Carbon nanotubes as sorbents in the analysis of pesticides. Chemosphere 83 1407–1413. 10.1016/j.chemosphere.2011.01.057 [ PubMed ] [ CrossRef ] [ Google Scholar ]
- Rai V., Acharya S., Dey N. (2012). Implications of nanobiosensors in agriculture. J. Biomater. Nanobiotechnol. 3 315–324. 10.4236/jbnb.2012.322039 [ CrossRef ] [ Google Scholar ]
- Raliya R., Tarafdar J. C., Gulecha K., Choudhary K., Ram R., Mal P., et al. (2013). Review article; scope of nanoscience and nanotechnology in agriculture. J. Appl. Biol. Biotechnol. 1 041–044. [ Google Scholar ]
- Rana S., Kalaichelvan P. T. (2013). Ecotoxicity of nanoparticles. ISRN Toxicol. 2013 : 574648 10.1155/2013/574648 [ PMC free article ] [ PubMed ] [ CrossRef ] [ Google Scholar ]
- Rana S., Kalaichelvan P. T. (2011). Antibacterial effects of metal nanoparticles. Adv. Biotech. 2 21–23. [ Google Scholar ]
- Rodríguez J., Martín M. J., Ruiz A. M., Clares B. (2016). Current encapsulation strategies for bioactive oils: from alimentary to pharmaceutical perspectives. Food Res. Int. 83 41–59. 10.1016/j.foodres.2016.01.032 [ CrossRef ] [ Google Scholar ]
- Sabir S., Arshad M., Chaudhari S. K. (2014). Zinc oxide nanoparticles for revolutionizing agriculture: synthesis and applications. Sci. World J. 2014 : 8 10.1155/2014/925494 [ PMC free article ] [ PubMed ] [ CrossRef ] [ Google Scholar ]
- Sadeghzadeh B. (2013). A review of zinc nutrition and plant breeding. J. Soil Sci. Plant Nutr. 13 905–927. 10.4067/S0718-95162013005000072 [ CrossRef ] [ Google Scholar ]
- Sagadevan S., Periasamy M. (2014). Recent trends in nanobiosensors and their applications - a review. Rev. Adv. Mater. Sci. 36 62–69. [ Google Scholar ]
- Saharan V., Mehrotra A., Khatik R., Rawal P., Sharma S. S., Pal A. (2013). Synthesis of chitosan based nanoparticles and their in vitro evaluation against phytopathogenic fungi. Int. J. Biol. Macromol. 62 677–683. 10.1016/j.ijbiomac.2013.10.012 [ PubMed ] [ CrossRef ] [ Google Scholar ]
- Savina E., Karlsen J. D., Frandsen R. P., Krag L. A., Kristensen K., Madsen N. (2016). Testing the effect of soak time on catch damage in a coastal gillnetter and the consequences on processed fish quality. Food Control 70 310–317. 10.1016/j.foodcont.2016.05.044 [ CrossRef ] [ Google Scholar ]
- Sayes C. M., Fortner J. D., Guo W., Lyon D., Boyd A. M., Ausman K. D., Tao Y. J., et al. (2004). The differential cytotoxicity of water-soluble fullerenes. Nano Lett. 4 1881–1887. 10.1002/btpr.707 [ CrossRef ] [ Google Scholar ]
- Sekhon B. S. (2014). Nanotechnology in agri-food production: an overview. Nanotechnol. Sci. Appl. 7 31–53. 10.2147/NSA.S39406 [ PMC free article ] [ PubMed ] [ CrossRef ] [ Google Scholar ]
- Sertova N. M. (2015). Application of nanotechnology in detection of mycotoxins and in agricultural sector. J. Cent. Eur. Agric. 16 117–130. 10.5513/JCEA01/16.2.1597 [ CrossRef ] [ Google Scholar ]
- Shweta, Tripathi D. K., Singh S., Singh S., Dubey N. K., Chauhan D. K. (2016). Impact of nanoparticles on photosynthesis: challenges and opportunities. Mater Focus 5 405–411. 10.1166/mat.2016.1327 [ CrossRef ] [ Google Scholar ]
- Siegrist M., Stampfli N., Kastenholz H., Keller C. (2008). Perceived risks and perceived benefits of different nanotechnology foods and nanotechnology food packaging. Appetite 51 283–290. 10.1016/j.appet.2008.02.020 [ PubMed ] [ CrossRef ] [ Google Scholar ]
- Silva Mdos S., Cocenza D. S., Grillo R., de Melo N. F., Tonello P. S., de Oliveira L. C., et al. (2011). Paraquat-loaded alginate/chitosan nanoparticles: preparation, characterization and soil sorption studies. J. Hazard. Mater. 190 366–374. 10.1016/j.jhazmat.2011.03.057 [ PubMed ] [ CrossRef ] [ Google Scholar ]
- Singh S., Vishwakarma K., Singh S., Sharma S., Dubey N.K., Singh V.K., et al. (2017). Understanding the plant and nanoparticle interface at transcriptomic and proteomic level: a concentric overview. Plant Gene (in press) 10.1016/j.plgene.2017.03.006 [ CrossRef ] [ Google Scholar ]
- Sun C., Shu K., Wang W., Ye Z., Liu T., Gao Y., et al. (2014). Encapsulation and controlled release of hydrophilic pesticide in shell cross-linked nanocapsules containing aqueous core. Int. J. Pharm. 463 108–114. 10.1016/j.ijpharm.2013.12.050 [ PubMed ] [ CrossRef ] [ Google Scholar ]
- Sun C. Q. (2007). Size dependence of nanostructures: impact of bond order deficiency. Prog. Solid State Chem. 35 1–159. 10.1016/j.progsolidstchem.2006.03.001 [ CrossRef ] [ Google Scholar ]
- Tadros T. F., Izquierdo P., Esquena J., Solans C. (2004). Formation and stability of nanoemulsions. Adv. Colloid Interface Sci. 108-109 303–318. 10.1016/j.cis.2003.10.023 [ PubMed ] [ CrossRef ] [ Google Scholar ]
- Thornhill S., Vargyas E., Fitzgerald T., Chisholm N. (2016). Household food security and biofuel feedstock production in rural Mozambique and Tanzania. Food Sec. 8 953–971. 10.1007/s12571-016-0603-9 [ CrossRef ] [ Google Scholar ]
- Tripathi D. K., Mishra R. K., Singh S., Singh S., Vishwakarma K., Sharma S., et al. (2017b). Nitric oxide ameliorates zinc oxide nanoparticles phytotoxicity in wheat seedlings: implication of the ascorbate-glutathione cycle. Front. Plant Sci 8 : 1 10.3389/fpls.2017.00001 [ PMC free article ] [ PubMed ] [ CrossRef ] [ Google Scholar ]
- Tripathi D. K., Shweta, Singh S., Singh S., Pandey R., Singh V. P.et al. (2016a). An overview on manufactured nanoparticles in plants: uptake, translocation, accumulation and phytotoxicity. Plant Physiol. Biochem. 110 2–12. 10.1016/j.plaphy.2016.07.030. [ PubMed ] [ CrossRef ] [ Google Scholar ]
- Tripathi D. K., Singh S., Singh V. P., Prasad S. M., Chauhan D. K., Dubey N. K. (2016b). Silicon nanoparticles more efficiently alleviate arsenate toxicity than silicon in maize cultiver and hybrid differing in arsenate tolerance. Front. Environ. Sci. 4 : 46 10.3389/fenvs.2016.00046 [ CrossRef ] [ Google Scholar ]
- Tripathi D. K., Singh S., Singh S., Srivastava P. K., Singh V. P., Singh S., et al. (2017a). Nitric oxide alleviates silver nanoparticles (AgNps)-induced phytotoxicity in Pisum sativum seedlings. Plant Physiol. Biochem. 110 167–177. 10.1016/j.plaphy.2016.06.015 [ PubMed ] [ CrossRef ] [ Google Scholar ]
- Tripathi D. K., Tripathi A., Shweta S. S., Singh Y., Vishwakarma K., Yadav G., et al. (2017c). Uptake, accumulation and toxicity of silver nanoparticle in autotrophic plants, and heterotrophic microbes: a concentric review. Front. Microbiol. 8 : 07 10.3389/fmicb.2017.00007 [ PMC free article ] [ PubMed ] [ CrossRef ] [ Google Scholar ]
- Tripathi D. K., Singh S., Singh V. P., Prasad S. M., Dubey N. K., Chauhan D. K. (2017d). Silicon nanoparticles more effectively alleviated UV-B stress than silicon in wheat ( Triticum aestivum ) seedlings. Plant Physiol. Biochem. 110 70–81. 10.1016/j.plaphy.2016.06.026 [ PubMed ] [ CrossRef ] [ Google Scholar ]
- Vannini C., Domingo G., Onelli E., De Mattia F., Bruni I., Marsoni M., et al. (2014). Phytotoxic and genotoxic effects of silver nanoparticles exposure on germinating wheat seedlings. J. Plant Physiol. 171 1142–1148. 10.1016/j.jplph.2014.05.002 [ PubMed ] [ CrossRef ] [ Google Scholar ]
- Vartholomeos P., Fruchard M., Ferreira A., Mavroidis C. (2011). MRI-guided nanorobotic systems for therapeutic and diagnostic applications. Annu. Rev. Biomed. Eng. 13 157–184. 10.1146/annurev-bioeng-071910-124724 [ PubMed ] [ CrossRef ] [ Google Scholar ]
- Venkatachalam P., Jayaraj M., Manikandan R., Geetha N., Rene E. R., Sharma N. C., et al. (2017). Zinc oxide nanoparticles (ZnONPs) alleviate heavy metal-induced toxicity in Leucaena leucocephala seedlings: a physiochemical analysis. Plant Physiol. Biochem. 110 59–69. 10.1016/j.plaphy.2016.08.022 [ PubMed ] [ CrossRef ] [ Google Scholar ]
- Vidotti M., Carvalhal R. F., Mendes R. K., Ferreira D. C. M., Kubota L. T. (2011). Biosensors based on gold nanostructures. J. Braz. Chem. Soc. 22 3–20. 10.1590/S0103-50532011000100002 [ CrossRef ] [ Google Scholar ]
- Viswanathan S., Radecki J. (2008). Nanomaterials in electrochemical biosensors for food analysis- a review. Pol. J. Food Nutr. Sci. 58 157–164. [ Google Scholar ]
- Wan Y., Li J., Ren H., Huang J., Yuan H. (2014). Physiological investigation of gold nanorods toward watermelon. J. Nanosci. Nanotechnol. 14 6089–6094. 10.1166/jnn.2014.8853 [ PubMed ] [ CrossRef ] [ Google Scholar ]
- Wang L., Hu C., Shao L. (2017). The antimicrobial activity of nanoparticles: present situation and prospects for the future. Int. J. Nanomed. 12 1227–1249. 10.2147/IJN.S121956 [ PMC free article ] [ PubMed ] [ CrossRef ] [ Google Scholar ]
- Yang L., Watts D. J. (2005). Particle surface characteristics may play an important role in phytotoxicity of alumina nanoparticles. Toxicol. Lett. 158 122–132. 10.1016/j.toxlet.2005.03.003 [ PubMed ] [ CrossRef ] [ Google Scholar ]
- Yunlong C., Smit B. (1994). Sustainability in agriculture: a general review. Agric. Ecosyst. Environ. 49 299–307. 10.1016/0167-8809(94)90059-0 [ CrossRef ] [ Google Scholar ]
- Zhang Q., Han L., Jing H., Blom D. A., Lin Y., Xin H. L., et al. (2016). Facet control of gold nanorods. ACS Nano 10 2960–2974. 10.1021/acsnano.6b00258 [ PubMed ] [ CrossRef ] [ Google Scholar ]

Sustainable Agriculture Research and Education in the Field: A Proceedings (1991)
Chapter: introduction, introduction.
Charles M. Benbrook
These proceedings are based on a workshop that brought together scientists, farmer-innovators, policymakers, and interested members of the public for a progress report on sustainable agriculture research and education efforts across the United States. The workshop, which was held on April 3 and 4, 1990, in Washington, D.C., was sponsored by the Office of Science and Education of the U.S. Department of Agriculture and the Board on Agriculture of the National Research Council. The encouraging new science discussed there should convince nearly everyone of two facts.
First, the natural resource, economic, and food safety problems facing U.S. agriculture are diverse, dynamic, and often complex. Second, a common set of biological and ecological principles—when systematically embodied in cropping and livestock management systems—can bring improved economic and environmental performance within the reach of innovative farmers. Some people contend that this result is not a realistic expectation for U.S. agriculture. The evidence presented here does not support such a pessimistic assessment.
The report of the Board on Agriculture entitled Alternative Agriculture (National Research Council, 1989a) challenged everyone to rethink key components of conventional wisdom and contemporary scientific dogma. That report has provided encouragement and direction to those individuals and organizations striving toward more sustainable production systems, and it has provoked skeptics to articulate why they feel U.S. agriculture cannot—some even say should not—seriously contemplate the need for such change. The debate has been spirited and generally constructive.
Scholars, activists, professional critics, and analysts have participated in
this debate by writing papers and books, conducting research, and offering opinions about alternative and sustainable agriculture for over 10 years. Over the past decade, many terms and concepts have come and gone. Most people—and unfortunately, many farmers—have not gone very far beyond the confusion, frustration, and occasional demagoguery that swirls around the different definitions of alternative, low-input, organic, and sustainable agriculture.
Fortunately, though, beginning in late 1989, a broad cross-section of people has grown comfortable with the term sustainable agriculture. The May 21, 1990, issue of Time magazine, in an article on sustainable agriculture entitled “It's Ugly, But It Works” includes the following passage:
[A] growing corps of experts [are] urging farmers to adopt a new approach called sustainable agriculture. Once the term was synonymous with the dreaded O word—a farm-belt euphemism for trendy organic farming that uses no synthetic chemicals. But sustainable agriculture has blossomed into an effort to curb erosion by modifying plowing techniques and to protect water supplies by minimizing, if not eliminating, artificial fertilizers and pest controls.
Concern and ridicule in farm publications and during agribusiness meetings over the philosophical roots of low-input, sustainable, or organic farming have given way to more thoughtful appraisals of the ecological and biological foundations of practical, profitable, and sustainable farming systems. While consensus clearly does not yet exist on how to “fix” agriculture's contemporary problems, a constructive dialogue is now under way among a broad cross-section of individuals, both practitioners and technicians involved in a wide variety of specialties.
This new dialogue is powerful because of the people and ideas it is connecting. Change will come slowly, however. Critical comments in some farm magazines will persist, and research and on-farm experimentation will not always lead to the hoped for insights or breakthroughs. Some systems that now appear to be sustainable will encounter unexpected production problems. Nonetheless, progress will be made.
The Board on Agriculture believes that over the next several decades significant progress can and will be made toward more profitable, resource-conserving, and environmentally prudent farming systems. Rural areas of the United States could become safer, more diverse, and aesthetically pleasing places to live. Farming could, as a result, become a more rewarding profession, both economically and through stewardship of the nation's soil and water resources. Change will be made possible; and it will be driven by new scientific knowledge, novel on-farm management tools and approaches, and economic necessity. The policy reforms adopted in the 1990 farm bill, and ongoing efforts to incorporate environmental objectives
into farm policy, may also in time make a significant difference in reshaping the economic environment in which on-farm management decisions are made.
This volume presents an array of new knowledge and insight about the functioning of agricultural systems that will provide the managerial and technological foundations for improved farming practices and systems. Examples of the research projects under way around the country are described. Through exploration of the practical experiences, recent findings, and insights of these researchers, the papers and discussions presented in this volume should demonstrate the value of field- and farm-level systems-based research that is designed and conducted with ongoing input from farmer-innovators.
Some discussion of the basic concepts that guide sustainable agriculture research and education activities may be useful. Definitions of key terms, such as sustainable agriculture, alternative agriculture, and low-input sustainable agriculture, are drawn from Alternative Agriculture and a recent paper (Benbrook and Cook, 1990).
BASIC CONCEPTS AND OPERATIONAL DEFINITIONS
Basic concepts.
Sustainable agriculture, which is a goal rather than a distinct set of practices, is a system of food and fiber production that
improves the underlying productivity of natural resources and cropping systems so that farmers can meet increasing levels of demand in concert with population and economic growth;
produces food that is safe, wholesome, and nutritious and that promotes human well-being;
ensures an adequate net farm income to support an acceptable standard of living for farmers while also underwriting the annual investments needed to improve progressively the productivity of soil, water, and other resources; and
complies with community norms and meets social expectations.
Other similar definitions could be cited, but there is now a general consensus regarding the essential elements of sustainable agriculture. Various definitions place differing degrees of emphasis on certain aspects, but a common set of core features is now found in nearly all definitions.
While sustainable agriculture is an inherently dynamic concept, alternative agriculture is the process of on-farm innovation that strives toward the goal of sustainable agriculture. Alternative agriculture encompasses efforts by farmers to develop more efficient production systems, as well as
efforts by researchers to explore the biological and ecological foundations of agricultural productivity.
The challenges inherent in striving toward sustainability are clearly dynamic. The production of adequate food on a sustainable basis will become more difficult if demographers are correct in their estimates that the global population will not stabilize before it reaches 11 billion or 12 billion in the middle of the twenty-first century. The sustainability challenge and what must be done to meet it range in nature from a single farm field, to the scale of an individual farm as an enterprise, to the food and fiber needs of a region or country, and finally to the world as a whole.
A comprehensive definition of sustainability must include physical, biological, and socioeconomic components. The continued viability of a farming system can be threatened by problems that arise within any one of these components. Farmers are often confronted with choices and sacrifices because of seemingly unavoidable trade-offs—an investment in a conservation system may improve soil and water quality but may sacrifice near-term economic performance. Diversification may increase the efficiency of resource use and bring within reach certain biological benefits, yet it may require additional machinery and a more stable and versatile labor supply. Indeed, agricultural researchers and those who design and administer farm policy must seek ways to alleviate seemingly unwelcome trade-offs by developing new knowledge and technology and, when warranted, new policies.
Operational Definitions
Sustainable agriculture is the production of food and fiber using a system that increases the inherent productive capacity of natural and biological resources in step with demand. At the same time, it must allow farmers to earn adequate profits, provide consumers with wholesome, safe food, and minimize adverse impacts on the environment.
As defined in our report, alternative agriculture is any system of food or fiber production that systematically pursues the following goals (National Research Council, 1989a):
more thorough incorporation of natural processes such as nutrient cycling, nitrogen fixation, and beneficial pest-predator relationships into the agricultural production process;
reduction in the use of off-farm inputs with the greatest potential to harm the environment or the health of farmers and consumers;
productive use of the biological and genetic potential of plant and animal species;
improvement in the match between cropping patterns and the productive potential and physical limitations of agricultural lands; and
profitable and efficient production with emphasis on improved farm management, prevention of animal disease, optimal integration of livestock and cropping enterprises, and conservation of soil, water, energy, and biological resources.
Conventional agriculture is the predominant farming practices, methods, and systems used in a region. Conventional agriculture varies over time and according to soil, climatic, and other environmental factors. Moreover, many conventional practices and methods are fully sustainable when pursued or applied properly and will continue to play integral roles in future farming systems.
Low-input sustainable agriculture (LISA) systems strive to achieve sustainability by incorporating biologically based practices that indirectly result in lessened reliance on purchased agrichemical inputs. The goal of LISA systems is improved profitability and environmental performance through systems that reduce pest pressure, efficiently manage nutrients, and comprehensively conserve resources.
Successful LISA systems are founded on practices that enhance the efficiency of resource use and limit pest pressures in a sustainable way. The operational goal of LISA should not, as a matter of first principles, be viewed as a reduction in the use of pesticides and fertilizers. Higher yields, lower per unit production costs, and lessened reliance on agrichemicals in intensive agricultural systems are, however, often among the positive outcomes of the successful adoption of LISA systems. But in much of the Third World an increased level of certain agrichemical and fertilizer inputs will be very helpful if not essential to achieve sustainability. For example, the phosphorous-starved pastures in the humid tropics will continue to suffer severe erosion and degradation in soil physical properties until soil fertility levels are restored and more vigorous plant growth provides protection from rain and sun.
Farmers are continuously modifying farming systems whenever opportunities arise for increasing productivity or profits. Management decisions are not made just in the context of one goal or concern but in the context of the overall performance of the farm and take into account many variables: prices, policy, available resources, climatic conditions, and implications for risk and uncertainty.
A necessary step in carrying out comparative assessments of conventional and alternative farming systems is to understand the differences between farming practices, farming methods, and farming systems. It is somewhat easier, then, to determine what a conventional practice, method, or system is and how an alternative or sustainable practice, method, or system might or should differ from a conventional one. The following definitions are drawn from the Glossary of Alternative Agriculture (National Research Council, 1989a).
A farming practice is a way of carrying out a discrete farming task such as a tillage operation, particular pesticide application technology, or single conservation practice. Most important farming operations—preparing a seedbed, controlling weeds and erosion, or maintaining soil fertility, for example—require a combination of practices, or a method. Most farming operations can be carried out by different methods, each of which can be accomplished by several unique combinations of different practices. The manner in which a practice is carried out—the speed and depth of a tillage operation, for example—can markedly alter its consequences.
A farming method is a systematic way to accomplish a specific farming objective by integrating a number of practices. A discrete method is needed for each essential farming task, such as preparing a seedbed and planting a crop, sustaining soil fertility, managing irrigation, collecting and disposing of manure, controlling pests, and preventing animal diseases.
A farming system is the overall approach used in crop or livestock production, often derived from a farmer's goals, values, knowledge, available technologies, and economic opportunities. A farming system influences, and is in turn defined by, the choice of methods and practices used to produce a crop or care for animals.
In practice, farmers are constantly adjusting cropping systems in an effort to improve a farm's performance. Changes in management practices generally lead to a complex set of results—some positive, others negative—all of which occur over different time scales.
The transition to more sustainable agriculture systems may, for many farmers, require some short-term sacrifices in economic performance in order to prepare the physical resource and biological ecosystem base needed for long-term improvement in both economic and environmental performance. As a result, some say that practices essential to progress toward sustainable agriculture are not economically viable and are unlikely to take hold on the farm (Marten, 1989). Their contention may prove correct, given current farm policies and the contemporary inclination to accept contemporary, short-term economic challenges as inviolate. Nonetheless, one question lingers: What is the alternative to sustainable agriculture?
PUBLIC POLICY AND RESEARCH IN SUSTAINABLE AGRICULTURE
Farmers, conservationists, consumers, and political leaders share an intense interest in the sustainability of agricultural production systems. This interest is heightened by growing recognition of the successes achieved by innovative farmers across the country who are discovering alternative agriculture practices and methods that improve a farm's economic and environmental performance. Ongoing experimental efforts on the farm, by no
means universally successful, are being subjected to rigorous scientific investigation. New insights should help farmers become even more effective stewards of natural resources and produce food that is consistently free of man-made or natural contaminants that may pose health risks.
The major challenge for U.S. agriculture in the 1990s will be to strike a balance between near-term economic performance and long-term ecological and food safety imperatives. As recommended in Alternative Agriculture (National Research Council, 1989a), public policies in the 1990s should, at a minimum, no longer penalize farmers who are committed to resource protection or those who are trying to make progress toward sustainability. Sustainability will always remain a goal to strive toward, and alternative agriculture systems will continuously evolve as a means to this end. Policy can and must play an integral role in this process.
If sustainability emerges as a principal farm and environmental policy goal, the design and assessment of agricultural policies will become more complex. Trade-offs, and hence choices, will become more explicit between near-term economic performance and enhancement of the long-term biological and physical factors that can contribute to soil and water resource productivity.
Drawing on expertise in several disciplines, policy analysts will be compelled to assess more insightfully the complex interactions that link a farm's economic, ecological, and environmental performance. It is hoped that political leaders will, as a result, recognize the importance of unraveling conflicts among policy goals and more aggressively seizing opportunities to advance the productivity and sustainability of U.S. agriculture.
A few examples may help clarify how adopting the concept of sustainability as a policy goal complicates the identification of cause-and-effect relationships and, hence, the design of remedial policies.
When a farmer is pushed toward bankruptcy by falling crop prices, a farm operation can become financially unsustainable. When crop losses mount because of pest pressure or a lack of soil nutrients, however, the farming system still becomes unsustainable financially, but for a different reason. In the former example, economic forces beyond any individual farmer's control are the clear cause; in the latter case the underlying cause is rooted in the biological management and performance of the farming system.
The biological and economic performance of a farming system can, in turn, unravel for several different reasons. Consider an example involving a particular farm that is enrolled each year in the U.S. Department of Agriculture's commodity price support programs. To maintain eligibility for government subsidies on a continuing basis, the farmer understands the importance of growing a certain minimum (base) acreage of the same crop each year. Hence, the cropping pattern on this farm is likely to lead to a
buildup in soilborne pathogens that attack plant roots and reduce yields. As a result, the farmer might resort to the use of a fumigant to control the pathogens, but the pesticide might become ineffective because of steadily worsening microbial degradation of the fumigant, or a pesticide-resistant pathogen may emerge.
A solution to these new problems might be to speed up the registration of another pesticide that could be used, or relax regulatory standards so more new products can get registered, or both. Consider another possibility. A regulatory agency may cancel use of a fumigant a farmer has been relying upon because of food safety, water quality, or concerns about it effect on wildlife. The farmer might then seek a change in grading standards or an increase in commodity prices or program benefits if alternative pesticides are more costly.
Each of these problems is distinctive when viewed in isolation and could be attacked through a number of changes in policy. The most cost-effective solution, however, will prove elusive unless the biology of the whole system is perceptively evaluated. For this reason, in the policy arena, just as on the farm, it is critical to know what the problem is that warrants intervention and what the root causes of the problem really are.
Research Challenges
In thinking through agricultural research priorities, it should be acknowledged that the crossroads where the sciences of agriculture and ecology meet remain largely undefined, yet clearly promising. There is too little information to specify in detail the features of a truly sustainable agriculture system, yet there is enough information to recognize the merit in striving toward sustainability in a more systematic way.
The capacity of current research programs and institutions to carry out such work is suspect (see Investing in Research [National Research Council, 1989b]). It also remains uncertain whether current policies and programs that were designed in the 1930s or earlier to serve a different set of farmer needs can effectively bring about the types of changes needed to improve ecological management on the modern farm.
In the 1980s, the research community reached consensus on the diagnosis of many of agriculture's contemporary ills; it may take most of the 1990s to agree on cures, and it will take at least another decade to get them into place. Those who are eager for a quick fix or who are just impatient are bound to be chronically frustrated by the slow rate of change.
Another important caution deserves emphasis. The “silver bullet” approach to solving agricultural production problems offers little promise for providing an understanding of the ecological and biological bases of sustainable agriculture. The one-on-one syndrome seeks to discover a new
pesticide for each pest, a new plant variety when a new strain of rust evolves, or a new nitrogen management method when nitrate contamination of drinking water becomes a pressing social concern. This reductionist approach reflects the inclination in the past to focus scientific and technological attention on products and outcomes rather than processes and on overcoming symptoms rather than eliminating causes. This must be changed if research aimed at making agriculture more sustainable is to move ahead at the rate possible given the new tools available to agricultural scientists.
One area of research in particular—biotechnology—will benefit from a shift in focus toward understanding the biology and ecology underlying agricultural systems. Biotechnology research tools make possible powerful new approaches in unraveling biological interactions and other natural processes at the molecular and cellular levels, thus shedding vital new light on ecological interactions with a degree of precision previously unimagined in the biological sciences. However, rather than using these new tools to advance knowledge about the functioning of systems as a first order of priority, emphasis is increasingly placed on discovering products to solve specific production problems or elucidating the mode of action of specific products.
This is regrettable for several reasons. A chance to decipher the physiological basis of sustainable agriculture systems is being put off. The payoff from focusing on products is also likely to be disappointing. The current widespread pattern of failure and consolidation within the agricultural biotechnology industry suggests that biotechnology is not yet mature enough as a science to reliably discover, refine, and commercialize product-based technologies. Products from biotechnology are inevitable, but a necessary first step must be to generate more in-depth understanding of biological processes, cycles, and interactions.
Perhaps the greatest potential of biotechnology lies in the design and on-farm application of more efficient, stable, and profitable cropping and livestock management systems. For farmers to use such systems successfully, they will need access to a range of new information and diagnostic and analytical techniques that can be used on a real-time basis to make agronomic and animal husbandry judgments about how to optimize the efficiencies of the processes and interactions that underlie plant and animal growth.
Knowledge, in combination with both conventional and novel inputs, will be deployed much more systematically to avoid soil nutrient or animal nutrition-related limits on growth; to ensure that diseases and pests do not become serious enough to warrant the excessive use of costly or hazardous pesticides; to increase the realistically attainable annual level of energy flows independent of purchased inputs within agroecosystems; and to maximize a range of functional symbiotic relationships between soil micro-
and macrofauna, plants, and animals. Discrete goals will include pathogen-suppressive soils, enhanced rotation effects, pest suppression by populations of plant-associated microorganisms, nutrient cycling and renewal, the optimization of general resistance mechanisms in plants by cultural practices, and much more effective soil and water conservation systems that benefit from changes in the stability of soil aggregates and the capacity of soils to absorb and hold moisture.
Because of the profound changes needed to create and instill this new knowledge and skills on the farm, the recommendations in Alternative Agriculture (National Research Council, 1989a) emphasize the need to expand systems-based applied research, on-farm experimentation utilizing farmers as research collaborators, and novel extension education strategies—the very goals of the U.S. Department of Agriculture's LISA program.
Future research efforts—and not just those funded through LISA—should place a premium on the application of ecological principles in the multidisciplinary study of farming system performance. A diversity of approaches in researching and designing innovative farming systems will ensure broad-based progress, particularly if farmers are actively engaged in the research enterprise.
Benbrook, C., and J. Cook. 1990. Striving toward sustainability: A framework to guide on-farm innovation, research, and policy analysis. Speech presented at the 1990 Pacific Northwest Symposium on Sustainable Agriculture, March 2.
Marten, J. 1989. Commentary: Will low-input rotations sustain your income? Farm Journal, Dec. 6.
National Research Council. 1989a. Alternative Agriculture. Washington, D.C.: National Academy Press.
National Research Council. 1989b. Investing in Research: A Proposal to Strengthen the Agricultural, Food, and Environmental System. Washington, D.C.: National Academy Press.
Interest is growing in sustainable agriculture, which involves the use of productive and profitable farming practices that take advantage of natural biological processes to conserve resources, reduce inputs, protect the environment, and enhance public health. Continuing research is helping to demonstrate the ways that many factors—economics, biology, policy, and tradition—interact in sustainable agriculture systems.
This book contains the proceedings of a workshop on the findings of a broad range of research projects funded by the U.S. Department of Agriculture. The areas of study, such as integrated pest management, alternative cropping and tillage systems, and comparisons with more conventional approaches, are essential to developing and adopting profitable and sustainable farming systems.
READ FREE ONLINE
Welcome to OpenBook!
You're looking at OpenBook, NAP.edu's online reading room since 1999. Based on feedback from you, our users, we've made some improvements that make it easier than ever to read thousands of publications on our website.
Do you want to take a quick tour of the OpenBook's features?
Show this book's table of contents , where you can jump to any chapter by name.
...or use these buttons to go back to the previous chapter or skip to the next one.
Jump up to the previous page or down to the next one. Also, you can type in a page number and press Enter to go directly to that page in the book.
Switch between the Original Pages , where you can read the report as it appeared in print, and Text Pages for the web version, where you can highlight and search the text.
To search the entire text of this book, type in your search term here and press Enter .
Share a link to this book page on your preferred social network or via email.
View our suggested citation for this chapter.
Ready to take your reading offline? Click here to buy this book in print or download it as a free PDF, if available.
Get Email Updates
Do you enjoy reading reports from the Academies online for free ? Sign up for email notifications and we'll let you know about new publications in your areas of interest when they're released.
share this!
June 20, 2024
This article has been reviewed according to Science X's editorial process and policies . Editors have highlighted the following attributes while ensuring the content's credibility:
fact-checked
trusted source
Greening the food supply chain: Developing sustainable food systems through interdisciplinary collaboration
by University of Connecticut
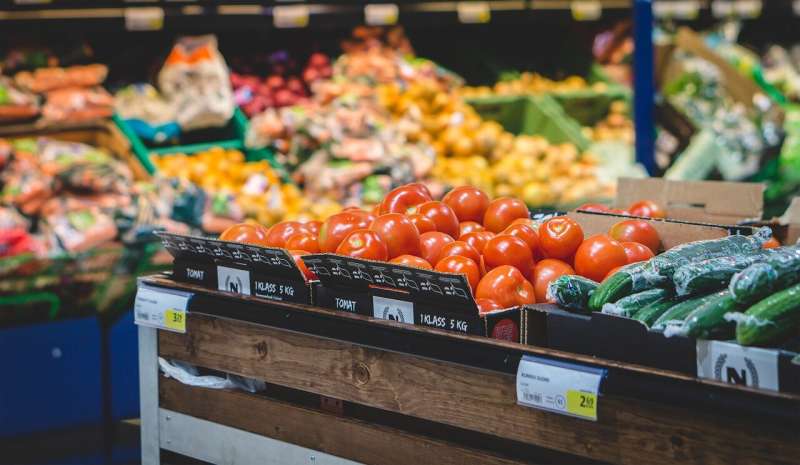
Sustainability is a hot topic in just about every field that engages with the environment, including agriculture. An interdisciplinary group of researchers in UConn's College of Agriculture, Health and Natural Resources has published a paper outlining the current state of sustainable food production research in the Journal of Agriculture and Food Research .
The group includes Yangchao Luo, associate professor of nutritional sciences; Zhenlei Xiao, associate professor-in-residence of nutritional sciences; and Abhinav Upadhyay, assistant professor of animal science. Bai Qu, Luo's Ph.D. student, is the lead author on the paper.
Sustainable food production focuses on creating food systems that are environmentally sound, economically viable, and socially equitable.
"It focuses on the entire food supply chain, from farm to table, ensuring that each step is sustainable, minimizes waste, and reduces the carbon footprint," Luo says.
The paper outlines the key features of sustainable food production including environmental stewardship , economic vitality, innovation and adaptation, and social responsibility.
The paper also reviews green technologies like urban agriculture , food nanotechnology, and plant-based foods, all of which play a role in reducing the negative impacts of food production.
"This is not a new concept, but I think with the development of emergent technology, a lot of things are going on now, it is very important to revisit this concept," Luo says.
This publication provides a holistic and interdisciplinary perspective on the topic.
"Sustainable food production is a very collaborative topic," Luo says. "You cannot do everything on your own."
Sustainable food production encompasses the concept of a circular economy in which the waste from one process or product can be reused elsewhere.
"People have not cared about the waste generated, the impact to the environment, whether it's sustainable or not," Luo says. "People are pretty much profit driven. Now we have to change the whole concept or else the entire agricultural industry cannot be sustainable."
This paper reflects the College and UConn's broader commitment to sustainability, Luo explains.
"There's many things in the College and at the University, campus-wide, that flow into this area that really inspire me to dive deeper into this topic," Luo says.
Luo, co-chair for CAHNR's committee for sustainable agriculture and food production, is currently working with a group of students to develop an organic poultry feed additive made from microalgae.
"You cannot think about sustainable agriculture from a single discipline," Luo says. "It has to be highly collective and collaborative from all three areas—society, environment, and community health. You have to connect all three angles together."
Provided by University of Connecticut
Explore further
Feedback to editors

Saturday Citations: Bulking tips for black holes; microbes influence drinking; new dinosaur just dropped
19 hours ago

China, France launch satellite to better understand the universe
Jun 22, 2024

Key mechanism in nuclear reaction dynamics promises advances in nuclear physics
Jun 21, 2024

Study challenges popular idea that Easter islanders committed 'ecocide'

New AI-driven tool improves root image segmentation

Many more bacteria produce greenhouse gases than previously thought, study finds

Stacking three layers of graphene with a twist speeds up electrochemical reactions

A black hole of inexplicable mass: JWST observations reveal a mature quasar at cosmic dawn

Beyond CRISPR: seekRNA delivers a new pathway for accurate gene editing

Transforming drug discovery with AI: New program transforms 3D information into data that typical models can use
Relevant physicsforums posts, covid virus lives longer with higher co2 in the air.
11 hours ago
Is meat broth really nutritious?
13 hours ago
Periodical Cicada Life Cycle
A dna animation.
Jun 15, 2024
Innovative ideas and technologies to help folks with disabilities
Jun 14, 2024
How do fetuses breathe in the womb?
More from Biology and Medical
Related Stories

Study proposes roadmap for integrating edge AI into farming
Jun 18, 2024

Microbial food as a food production strategy of the future
Apr 12, 2024

Sustainable business models infused with agri-innovation systems can reduce post-harvest food loss and waste
Jun 6, 2024

Insect frass becomes food for protein-rich microalgae
May 29, 2024

New study shows ways forward for future EU food labeling
Mar 27, 2024

Unlocking the potential of brewer's spent grain: Sustainable biorefinery approach and value-added product generation
Jun 26, 2023
Recommended for you

Circular food systems found to dramatically reduce greenhouse gas emissions, require much less agricultural land

New tomato, potato family tree shows that fruit color and size evolved together

Paper-based biosensor offers fast, easy detection of fecal contamination on produce farms
Jun 20, 2024

Improving crops with laser beams and 3D printing
Jun 19, 2024

Hope from an unexpected source in the global race to stop wheat blast

Look to women for sustainable livestock farming bordering the Amazon rainforest, says study
Let us know if there is a problem with our content.
Use this form if you have come across a typo, inaccuracy or would like to send an edit request for the content on this page. For general inquiries, please use our contact form . For general feedback, use the public comments section below (please adhere to guidelines ).
Please select the most appropriate category to facilitate processing of your request
Thank you for taking time to provide your feedback to the editors.
Your feedback is important to us. However, we do not guarantee individual replies due to the high volume of messages.
E-mail the story
Your email address is used only to let the recipient know who sent the email. Neither your address nor the recipient's address will be used for any other purpose. The information you enter will appear in your e-mail message and is not retained by Phys.org in any form.
Newsletter sign up
Get weekly and/or daily updates delivered to your inbox. You can unsubscribe at any time and we'll never share your details to third parties.
More information Privacy policy
Donate and enjoy an ad-free experience
We keep our content available to everyone. Consider supporting Science X's mission by getting a premium account.
E-mail newsletter
Academia.edu no longer supports Internet Explorer.
To browse Academia.edu and the wider internet faster and more securely, please take a few seconds to upgrade your browser .
- We're Hiring!
- Help Center
Sustainable agriculture
- Most Cited Papers
- Most Downloaded Papers
- Newest Papers
- Save to Library
- Last »
- Agriculture Follow Following
- Agronomy Follow Following
- Organic agriculture Follow Following
- Agricultural Economics Follow Following
- Agriculture and Food Studies Follow Following
- Agroecology Follow Following
- Horticulture Follow Following
- Production System of Guava Follow Following
- Organic Farming Follow Following
- Agribusiness Follow Following
Enter the email address you signed up with and we'll email you a reset link.
- Academia.edu Publishing
- We're Hiring!
- Help Center
- Find new research papers in:
- Health Sciences
- Earth Sciences
- Cognitive Science
- Mathematics
- Computer Science
- Academia ©2024

IMAGES
VIDEO
COMMENTS
The Journal of Sustainable Agriculture and Environment (JSAE) is a fully open access journal, publishing high quality, original research, review and opinion papers in all areas of agriculture and environmental sciences and relevant policies. We explicitly encourage submissions on mechanistic understanding, sustainable approaches, and emerging tools to fill current gaps in the publishing industry.
The integration of technology and innovation is a key aspect of water resources. engineering in modern farming. Advanced sensor-based monitoring systems. enable real-time data collection on soil ...
Tel.: +49-4131-677-1582. Academic Editor: Marc A. Rosen. Received: 5 March 2015 / Accepted: 10 June 2015 / Published: 18 June 2015. Abstract: The idea of a sustainable agriculture has gained ...
With the right set of changes in practices and interventions, informed by academic research and practice, there is still hope to achieve SDG 2 on time. ... Sustainable agriculture. Nat Sustain 1 ...
The number of scientific papers focusing on sustainable agriculture, based on Scopus database, June 2020. The abundant scientific material extensively analyses the objectives, principles, and measures (indicators) of SA, provides recommendations for programs for SA implementation, as well as reports on successes on pathways toward SA and ...
Sustainable agriculture seeks to balance human nutritional needs with the preservation of environmental quality and the economic viability of agricultural systems. Principles of resource conservation, economic resilience, social equity, and competitiveness underpin this approach. Despite its potential to address crucial issues like food security, energy sustainability, and environmental ...
Since the Green Revolution, worldwide agriculture has been characterized by a typical top-down approach. The degree of autonomy, creativity, and responsibility of farmers has been limited by the continuous external inputs of chemicals, machinery, advice, subsidies and knowledge.The issue of sustainability has brought complexity and uncertainty to this mainly linear process of innovation ...
This section publishes research on sustainable agriculture, food security, and environmental protection. It covers topics such as crop production, agroecosystems, bioenergy, biotechnology, and policy.
There are two valuable outcomes from this research. The first is a framework for understanding the components of sustainable agriculture. The second outcome is in highlighting ways for actors involved with sustainable agriculture to deal with the complexity and multiplicity of this concept in a constructive manner. 1.
Explore the latest full-text research PDFs, articles, conference papers, preprints and more on SUSTAINABLE AGRICULTURE. Find methods information, sources, references or conduct a literature review ...
Again, a considerable gap between Sustainable Agriculture research involving "landscape" is related to natural and cultural domains. However, the keywords such as "Sustainability" and "organic farming " has a relationship with research trends. ... EfD Discussion Paper 09-09, Environment for Development Initiative and Resources for the Future ...
There is a dearth of literature that provides a bibliometric analysis concerning the role of Artificial Intelligence (AI) in sustainable agriculture therefore this study attempts to fill this research gap and provides evidence from the studies conducted between 2000-2021 in this field of research. The study is a systematic bibliographic analysis of the 465 previous articles and reviews done ...
Research paper Published 24 May 2022 Updated 27 May 2022 ISBN: 978 1 78413 526 3 DOI: 10.55317/9781784135263 Professor Tim Benton ... The arguments in support of both Version 1 and Version 2 of sustainable agriculture and food systems tend to be primarily based on assumptions that may be pragmatic ('that is the way the world is'), or that ...
Sustainable Agriculture Research (p-ISSN 1927-050X e-ISSN 1927-0518) is an international, double-blind peer-reviewed, open-access journal. ... Each paper published in Journal of Agricultural Science is assigned a DOI®number, which appears beneath the author's affiliation in the published paper.
The implication of the nanotechnology research in the agricultural sector is become to be necessary even key factor for the sustainable developments. In the agri-food areas pertinent applications of nanotubes, fullerenes, biosensors, controlled delivery systems, nanofiltration, etc. were observed ( Ion et al., 2010 ; Sabir et al., 2014 ).
Sustainable Agricultural Systems Science White Paper U.S. Department of Agriculture Research, Education and Economics Office of the Chief Scientist July 24, 2012. The concept of sustainability has been prominent since the 1987 report of the World Commission on Environment and Development (Bruntland, 1987). Sustainability and its relevance to U ...
this debate by writing papers and books, conducting research, and offering opinions about alternative and sustainable agriculture for over 10 years. Over the past decade, many terms and concepts have come and gone. Most people—and unfortunately, many farmers—have not gone very far beyond the confusion, frustration, and occasional ...
Because of inconstant nature, production and storage; FYM have N, P, and K contents stretching from 0.01% to 1.9% on dry weight basis. The ne decomposed FYM contains 0.2% of P 2O5, 0.5% N, and 0.5 ...
JETIR2109027 Journal of Emerging Technologies and Innovative Research (JETIR) www.jetir.org a174 Current research needs for sustainable agriculture Irene F Lalrintluangi1 Dipti Bisarya1*, Vinai Kumar2 and Lalnunsanga3, 1M. Sc. Agronomy, School of Agriculture, Lovely Professional University, Phagwara, Punjab
An interdisciplinary group of researchers in UConn's College of Agriculture, Health and Natural Resources has published a paper outlining the current state of sustainable food production research ...
Sustainable agriculture is the efficient production of safe, h igh-quality agricultural products in a way that protects and improve s the natural environment, the
Gender Inequality in Nepalese Agriculture: Issues Concerning Sustainability and Food Security. Despite of the advancement in technology and excessive use of chemical fertilizers for agricultural production, about 870 million people around the globe are hunger stricken due to various resource scarcities.
Anagha Bhilare, (2013), Sustainable Agriculture and Land Management, Research India Publications, International Journal of Agriculture and Food Science Technology. Volume 4, Number 9, pp. 881-886.
The rise of advanced artificial intelligence (edge AI) could well mark the beginning of a new era for sustainable agriculture. A recent study proposes a roadmap for integrating this technology ...
Sustainability implies maintaining high yields in the face of major shocks, and agricultural practices that have acceptable environmental impacts. This review paper aimed to examine how and why ...