Climate change and the built environment - a systematic review
- Open access
- Published: 11 May 2024

Cite this article
You have full access to this open access article
- Ibrahim Tajuddeen 1 &
- Seyed Masoud Sajjadian 2
334 Accesses
9 Altmetric
Explore all metrics
Recent intergovernmental panel on climate change reports have once again emphasised the effective measures to reduce greenhouse gas emissions and the importance of the built environment. Historically, passive and active solutions are known for their potential to make the built environment more environmentally friendly. Recently, a significant number of studies covered the effectiveness of such solutions under distinct current and different future climate and emission predictions. Through the PRISMA framework, this paper presents a comprehensive state-of-the-art review of such studies within the last 10 years (2013–2023) to understand their impact, their tangible applications, and their empirical evidence. Local ecosystems, weather patterns, geographical and cultural challenges dictate the solutions for a warmer future. Among the solutions, as expected, passive solutions remain most effective even though a combination with active ones is necessary regardless of the context. The review in this paper is expandable beyond the effective reported solutions and it highlights the most effective solutions under different climate zones.
Similar content being viewed by others
Cohesion: Our Environment—Building Better and Smarter
Climate Change and the Built Environment in the Tropics – Is Carbon Enough to Assess Human Impact?
Building Resilient Cities to Climate Change
Avoid common mistakes on your manuscript.
1 Introduction
1.1 review of intergovernmental panel on climate change (ipcc) reports.
Once again, the significant impacts of anthropogenic activities in warming up the climate have been captured in the IPCC’s Sixth Assessment Report (IPCC, 2021 ). CC is posing an increased frequency and intensity of heat waves, with irrevocable changes in dehydration, heat stroke and heat exhaustion, as well as mortality (IPCC, 2021 ; WMO & WHO, 2015 ). Inherently, humans will find all possible measures to mitigate these adverse effects, leading to an unprecedented increase in cooling demand and consequently greenhouse gas (GHG) emissions which further promote the CC (Mehmood et al., 2022 ). The increase of the GHG is responsible for an above-average surface temperature within the span of 1951–2010 (Climate Change, 2014 : Synthesis, 2014 ). Thus, the IPCC has emphasised that more and more reduction in GHG is needed to avoid global temperature going beyond the 1.5 °C warming limit. In the Working Group III contribution to the Sixth Assessment Report, under the Summary for Policy Makers (SPM), section C.7.3, the IPCC asserts that bottom-up studies have shown that by 2050, up to 61% (8.2 Gt C0 2 ) of global building emissions can be alleviated (Shukla et al., 2022 ). Under the same section, the IPCC mentioned that sufficiency policies that avoid the demand for energy and materials contribute about 10% to this potential, while energy efficiency policies contribute about 42%, and renewable energy policies contribute about 9%. The report went on by saying that the largest portion of the mitigation potential of new buildings is available in developing countries while in developed countries the biggest mitigation potential lies within the retrofit of existing buildings. The 2020–2030 decade is critical for expediting the development of skills needed to fully capture the mitigation potential of buildings (IEA, 2018 ).
Even though peculiar mitigation potentials to the built environment are being developed, to stress the impact of CC, some scientific studies among numerous ones related to the science of the total environment in the literature have pointed out the solutions to mitigate the trend of environmental degradation arising from CC. For example, mitigations concerning the reduction in the loss of natural resources, like precipitation (Meshram et al., 2018 ) and reduction in the loss of potentials for sustainable rural and urban land usage, as well as wetland use (Aslam et al., 2023 ; Waqar et al., 2023 , 2024a , 2024b ; Zhao et al., 2023 ). Some studies also try to understand the effects of pollution on the environment arising from human behaviours affecting the climate, like black carbon aerosol contaminated air mass (Hussain et al., 2023 ); concentrations of polycyclic aromatic hydrocarbons (Ambade et al., 2021a , 2021b ); concentrated particles matter (PM 10 and PM 2.5 ) (Ambade, 2016 ; Ambade et al., 2022 ); and microbiological contamination (Naz et al., 2024 ). In all of these studies, the main objectives are to set aside measures in preparedness to combat CC in the near future.
Furthermore, in order to set a specific goal to widen the recognition of measures to combat CC, the IPCC in its Fifth Assessment Report (AR5) (Edenhofer et al., 2014 ), created Representative Concentration Pathways (RCP) scenarios to assess future possible risks and debate how decisions may influence the coming future. The RCP contain approximately thirty candidate scenarios, however, predominantly, four primary scenarios were reported by researchers (Bass & New, 2023 ; Vuuren et al., 2011 ). These four scenarios modelled by different climate groups across the globe are the RCP 8.5 RCP 6, RCP 4.5 and RCP 2.6 (Fig. 1 ). Each RCP defines a specific emissions pattern, the projected energy consumption and the subsequent radiative forcing (Moss et al., 2010 ). The radiative forcing in W/m 2 is the influence a factor has in tempering the balance of incoming and outgoing energy in the Earth-atmosphere system (Moss et al., 2010 ). The RCP 8.5 was developed in Austria, using the MESSAGE model and the Integrated Assessment Framework by the International Institute for Applied Systems Analysis (IIASA). It is characterised by increasing GHG over time and radiative forcing (> 8.5 W/m 2 ), representative of scenarios in the literature that lead to high GHG concentration levels (Riahi et al., 2007 ). The RCP 6 was developed by the AIM modelling group at the National Institute for Environmental Studies (NIES) in Japan. It is known as a stabilisation scenario where total radiative forcing (W/m 2 ) is stabilised shortly after 2100, without further going beyond, through the application of technologies and strategies for reducing GHG (Fujino et al., 2006 ; Hijioka et al., 2008 ).
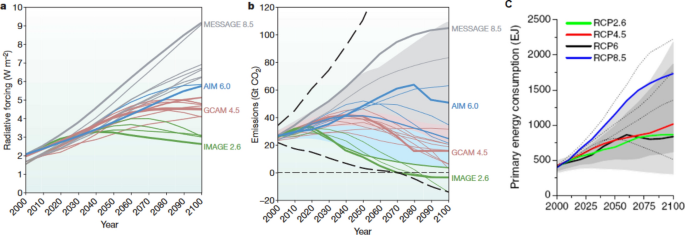
Future emission, radiative forcing and primary energy consumption under different scenarios (Waqar et al., 2024b )
The RCP 4.5 was developed by the GCM modelling group at the Pacific Northwest National Laboratory’s Joint Global Change Research Institute (JGCRI) in the United States. It is also a stabilisation scenario, where the total radiative forcing (> 4.5 W/m 2 ) stabilises just after 2100, and also not going beyond the future radiative target level (Clarke et al., 2007 ; Smith & Wigley, 2006 ; Wise et al., 2009 ). The RCP 2.5 was developed by the IMAGE modelling group in the Netherlands. The emission pathway is representative of scenarios in the literature that lead to very low GHG concentration levels. It is a scenario which peaked and declined, having the highest radiative forcing level of (> 3.1 W/m 2 ) by 2050, and returns to 2.6 W/m 2 by 2100 (Vuuren et al., 2006 , 2011 ).
The IPCC focus and evidences are essential to this paper to rigorously emphasize the potential of the foreseeable CC impacts to the built environment, and to understand the corresponding mitigation measures. Several studies have widely reported different passive, active and renewable measures used in the built environment (Cardinale et al., 2013 ; Herrera-Gomez & Quevedo-Nolasco, 2017 ; Pierangioli et al., 2017 ; Rañeses et al., 2021 ; Toe & Kubota, 2015 ). Only recently have some studies (due to the emerging global urge towards climate mitigations) focused on reviews of some past investigations which analyse the role of passive, active and renewable solutions in the built environment, and having practical applications in mitigating the effect of CC in the current and different future climate scenarios (Andric et al., 2019 ; Bass & New, 2023 ; Díaz-Lopez et al., 2022 ; Fereidani et al., 2021 ; Nair et al., 2022 ; Ozarisoy & Altan, 2021 ; Perez-Andreu et al., 2018 ; Soaresa et al., 2017 ; Zhou et al., 2020a , 2020b ). This paper aims to provide a comprehensive evaluation of viable passive and active solutions that have demonstrated efficacy in CC mitigation and adaption.
1.2 Gap and contribution of this paper
Previous analyses on active and passive investigations to mitigating CC for either current or future scenarios, collated in past literature reviews have raised questions regarding the completeness of their analysis. For example, in a review paper by Andric et al., ( 2019 ), some investigations on passive and active strategies as well as their favourable impacts on renovation measures were reported under future climate scenarios. However, among the investigations on the strategies reviewed, the most recent was published in 2018 (Andric et al., 2018 ). Although, the review in Andric et al. ( 2019 ) summarily reported the impacts of some of the investigated passive strategies targeted toward future climate scenarios, the reported investigations were few (n = 13). Similarly, Fereidani et al., ( 2021 ) comprehensively reviewed a wide range of different passive and active strategies, but the review ended with a summary of a few reports (5 in number) where energy consumption on heating/cooling was included under future climate scenarios. In fact, among these reports, only one (Andric et al., 2020 ), published in 2020, explicitly discussed passive strategies (green roof, green wall, insulation and efficient window glazing) to address the impacts of future climate scenarios. Perez-Andreu et al., ( 2018 ) reported an in-exhaustive summary of CC’s impact on heating and cooling demand, with some investigations amongst involved corresponding passive strategies to mitigate such impact. Comparable to the aforementioned reports in the reviews (Andric et al., 2019 ; Fereidani et al., 2021 ), the most recent of these investigations was published in 2017 (Pierangioli et al., 2017 ). Considering the period between 2017 and 2023, more investigations on passive and active strategies under future climate scenarios have been published and therefore a need for an update is necessary.
There are a limited number of studies that cover recent research on effective active and passive measures for CC mitigation. To the best of our knowledge, this is the first comprehensive update that includes the latest strategies specifically effective for warmer conditions. The specific contribution of this paper is an expandable, comprehensive and up-to-date review of CC mitigation measures.
2 Methodology
Initially, five combinations of keywords were used to retrieve records from the Scopus database the largest scientific database covering most of the peer-reviewed (Q1 and Q2) journals published by Elsevier, Springer, MDPI, SAGE, Wiley and Sons, Taylor & Francis. The searches were limited to a 10-year window period, covering all articles (journals and conference proceedings) published between 2013 and January 2023, in the English Language. The keyword combinations are climate change, built environment (2764 records); climate change, building operation, impact (395 records); climate change, building operation, built environment (83); climate change, buildings, passive solutions, active solutions (44); climate change, IPCC, built environment (17). These five keyword combinations were coined to reflect the specific goal and the filtering method proposed in this paper (Fig. 2 ).
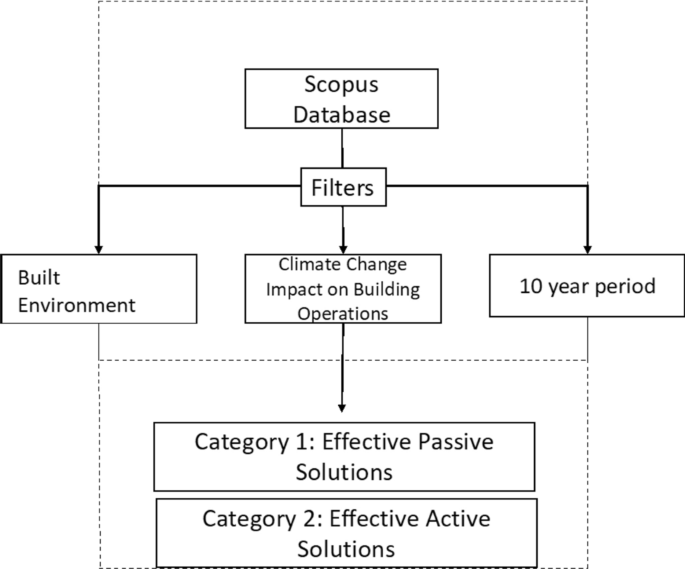
Method for the filtering of studies included
A total of 3299 records were found and all records were exported to an Excel Package, where initial screening of duplicates and no Author(s) was conducted. All screenings were done using the method of the Preferred Reporting Items for Systematic Reviews and Meta-Analysis (PRISMA) (Fig. 3 ). To justify the choice of PRISMA in this paper, a recent review study (Cui et al., 2022 ) on the effect of CC on critical infrastructure proved the PRISMA review system to be efficient in a systematic review where records of studies of interest are screened and deliberately categorised under different sections for in-depth analysis. Therefore, a total of 156 records were screened out for duplicates and no Author(s), and 3143 were the remainder. Studies which do not fall within the scope of the built environment (2700 records) were directly screened out right from the initial title screening stage, and a total number of 443 were remaining. These remainders were further meticulously and rigorously screened out in the abstract screening stage, with the target to extract the full-text studies which contain reports on passive, renewable active solutions, and 62 studies were recorded as new studies and reports. These new studies were considered for full eligibility as detailed in Fig. 3 by the PRISMA chart. This paper was only limited to passive and active solutions which reported only empirical investigations, and those without such were rather not retrieved. Because this paper is a systematic update, a further 12 reviews were separately screened and included as previous studies. These studies are termed as the previous version of the review (see Fig. 3 ). Some 79 reports from the previous version of the review were included as parts of the total reports of included studies. Other relevant 4 sources from citation searching were included. In all, a total number of 78 studies and 145 reports were recorded.
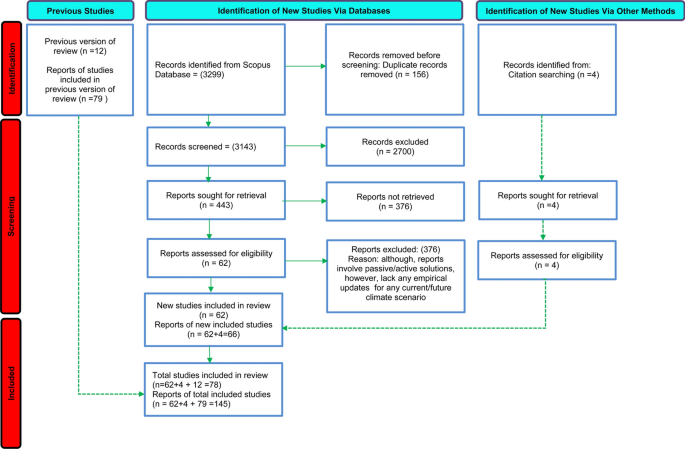
PRISMA framework adopted in this study
This section encompasses the reports of the effective passive solutions and empirical findings cover the impacts of these effective solutions on both energy demand for cooling/heating and thermal comfort of occupants for current and future climate scenarios. A summary of key studies is provided in Table 1 .
3.1 Category 1: effective passive solutions
3.1.1 ventilation-related measures.
Cui et al., ( 2022 ) used the Bayesian bootstrap as a promising technique to quantitatively evaluate the robustness of thermal comfort against uncertain future climate scenarios (the 2030s, 2050s and 2080s). However, the results concluded that adaptive night ventilation is potentially the key effective solution in all scenarios. Mehmood et al., ( 2022 ) conducted a study by using a two-story multi-family building as the baseline archetype in southern Asia, Pakistan. In order of importance, ventilative cooling, reflective and ventilated roofs, shading in windows, and roof insulation were the effective solutions for energy savings and reduction in discomfort hours, for 2020, 2050, and 2080 climate scenarios. The study reported that the passive solutions can improve energy demands from 13.1 to 7.1 kWh/m 2 and indoor discomfort from 320 to 131 h. In view of future higher temperatures (2050, 2080), Nunes and Giglio ( 2022 ) used Morris sensitivity analysis for a current and future climate and concluded that natural ventilation (Natural vent), solar absorptance of the envelope (Envelope Abs ) and thermal transmittance of the walls (Walls Trans ) respectively, are the most important parameters for the Degree Hour for Cooling (DHC); while Envelope Abs and Walls Trans , in a respective order, are the most important parameters for energy savings with regards to cooling for all the climate scenarios. The Morris’ elementary effect used by the author is summarised in the equations below to determine the indices \(u, {u}^{*} and \sigma \) :
where \(i\) is variable; \(r\) is the number of samples path; \(j\) is each path in the sample space of each variable \(i\) ; \({u}_{i}\) is the mean of the elementary effects of the variable \(i\) ; \({u}^{*}\) is the mean, in absolute value, of the elementary effects of the variable \(i\) ; \({\sigma }_{i}\) is the standard deviation of the elementary effect of the variable \(i\) ; \({d}_{i}\left({x}_{j}\right)\) is the elementary effect of the \(ith\) variable in path \(j\) .
A comparison of \(u\) allows the identification of parameters which have more influence on the model response. The higher the \(u\) , the greater the influence, \({u}^{*}\) being more comprehensive. Moreover, the \({\sigma }_{i}\) allows the identification of parameters with nonlinear behaviour or those that interact with other parameters, and the higher \({\sigma }_{i}\) the greater the interaction with other parameters, or more elaborate nonlinear behaviour.
For a current climate (2016), Costanzoa et al., ( 2018 ) compared the result of the overheating hour of an existing Passivhaus and found using a hybrid ventilation strategy instead of mechanical ventilation with heat recovery, lowering the insulating materials thickness (up to a third) applied to the roof and to the walls, as well as using double-glazed low-emissive windows in place of triple-glazed ones, is the best scenario analysis, and reduce the overheating hour to less than 20% compared to the existing building. In the study by Laouadi et al., ( 2020 ), indoor overheating guidelines of the Chartered Institute of Building Services Engineers (CIBSE) and the Passive House Institute (PHI) were used to compare the effect of natural ventilation (Natural vent) and exterior shade on overheating risk. With neutral nighttime temperature between 25 °C and 26 °C, according to CIBSE and PHI, the authors found that the natural ventilation significantly reduced the minimum nighttime temperature to 26 °C (close to neutral temperature for sleep) compared to exterior shade having nighttime temperature above 28 °C (This study only considers the current climate). Botti and Ramos ( 2017 ) used environmental solutions-virtual environment (IES-VE) simulation and sensitivity analysis to rank the effective measures of a care home. The findings show that solar shading, natural ventilation and thermal mass, respectively, are the most effective for current and future climate scenarios. Similar to Botti and Ramos ( 2017 ), Heracleous et al., ( 2021 ), also used IES-VE simulation to assess the potential of heat recovery ventilation and all other passive measures (e.g. cross ventilation; insulation on floor, wall, and roof; e-low glazing and shading devices), on the degree hour for cooling. The authors noted the combination of these measures could lead to a reduction in degree hour for cooling by 51% in the current climate, 60% in the 2050 period and 66.4% in the 2090 period. Hamdy et al., ( 2017 ) considered a current and future climate to test different ventilation rates in alleviating the risk of overheating in Dutch house stocks. A ventilative cooling rate of 5 Air change rate (ACH) on average, could reduce the overheating risk by 90% for the current climate, and 65% on average, for the future outdoor rising temperature (5.4 °C higher). For the climate scenario, A2 for 2030, Roetzel et al., ( 2013 ) identified typical patterns and key parameter optimisation for three climate zones across the world. Among the occupants’ scenarios optimised, using an office cellular room, the result depicts that natural night ventilation of at least 6 h is optimum, and WWR above 70% could enhance the thermal comfort condition indoors for a future warmer climate. Although, Hooff et al., ( 2014 ), considered a future climate in a study which investigated a range of climate change adaption measures, but the future climate being considered was a representative one (2006 period). The authors claimed that the heat waves across this period could be similar to those of the possible future climate scenarios (e.g. IPCC A2 scenario). The results of the investigated adaption measures in the study showed that exterior solar shading followed by natural ventilation could significantly mitigate overheating. Solgi et al., ( 2017 ) conducted experiments on the benefits of night ventilation at 15 ACH with Phase Change Materials (PCM), and the peak temperature for two summer months, July and September, were reduced by 6.54 °C and 4.38 °C respectively. The study claimed that in the month of September, the peak temperature was reduced to 26.79 °C and also noted that this fell below the maximum threshold comfort temperature (28 °C). For a climate model CESM RCP 8.5, Wang et al., ( 2017 ) compared mixed-mode ventilation and adaptive active measures (the combination of adjustment of room temperature setpoint, HVAC operation hours and minimum airflow fractions of VAV boxes). The mixed-mode ventilation effective solution can reach a reduction of 7.4% (728.4 GJ) in whole building source energy use in 2080s in Washington DC, while the adaptive measures can reach a reduction of 2.5% (246.1 GJ) in whole building source energy use in 2080s in Washington DC.
Lapisa et al., ( 2020 ) identified parameter sensitivity for different passive cooling solutions using the Non-dominated Sorting Genetic Algorithm II (NDGA-II). The results emphasised that, for the southern regions of France, the optimum set point temperature considering natural night ventilation is 18–26 °C. Also, for a trade-off between energy demand and thermal discomfort for current and 2080, the authors suggested that the albedo value of the solar reflectance of the cool roof could be 0.46–0.74. For the minimised building primary energy consumption for heating and lighting (EP [kWh/m 2 /yr]) and degree-hours of summer thermal discomfort (DH [°Ch]) through the passive measures for the future climate scenario, the authors described the formulation of the objective function of the NDGA-II based on the number of objectives ( \(DH\) and \(EP\) ) in the following equations as:
By the above objective function, the Pareto optimal solution was achieved through the trade-off of the objectives ( \(DH\) and \(EP\) ), normalized between the minimum and maximum values of the arbitrary coefficient \({(R}_{EP}, {R}_{DH}\) ,) with respect to A,B with C as the possible optimal solution (Fig. 4 ).
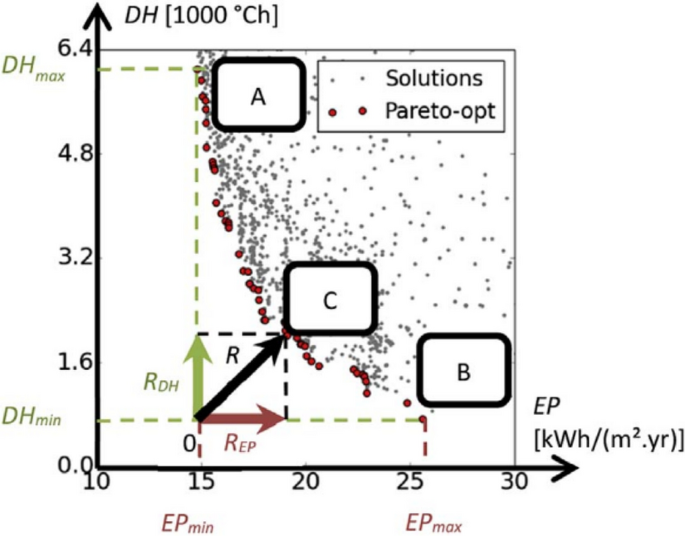
Pareto optimal solutions and trade-off solution (A, B, C) (Andric et al., 2020 )
For \({R}_{EP}\) and \({R}_{DH}\) in A and B: \({R}_{EP}=0\) (most energy efficient), \({R}_{DH}=1\) (most summer discomfort hour), \({R}_{DH}=0\) (least summer discomfort hour), \({{\text{R}}}_{{\text{EP}}}=1\) (least energy efficient).
The trade-off solution C which is the Pareto optimal solution, C is represented in the study by a minimum value of \(\overline{R}\) given as:
where \({R}_{EP}\) and \({R}_{DH}\) are weighing factors which define the trade-off and were given in the study as:
Yildiz ( 2015 ) adopted Givoni’s bioclimatic chart to assess the potential impacts of passive strategies on energy demand and thermal comfort level for different regions in Turkey. For the region of Istanbul, the potential use of evaporative cooling together with thermal mass and ventilation have been shown to have good promise to thermal comfort, with 1·4% for current typical meteorological year (TMY2), 3·97% for 2020s, 4·75% for 2050s and 5·95% for 2080.
Miri and Babakhani ( 2021 ) explored the potential of a vernacular windcatcher in a mountainous region, in the specific Mediterranean-to-cold climate in Western Iran to provide energy-efficient cooling and ventilation. By considering data from the current climate scenario, the authors explored the natural forces of wind and buoyancy using computational fluid dynamics (CFD), and the results of the investigation proved that in fact, the traditional windcatcher is more effective than the natural ventilation and cooling systems which are provided only through the openings of windows and doors by 101%, and its performance is comparable to modern wind catchers. Likewise, Pelletier and Calautit ( 2021 ) compared the performance of a windcatcher with a heat transfer device (HTD) to a windcatcher with a novel helical coil heat transfer device (HCHTD), however, under the current hot climate scenario in Sydney, Australia. After the analyses using a CFD simulation, the results showed that the windcatcher model with the HCHTD led to an increase of 4% airflow than the HTD model, which can achieve a comfortable level of cooling ranging from 14.25 K to 8.6 K for wind speeds of 1–4 m/s. It is also capable of meeting fresh air ventilation requirements in low wind speed scenarios. The authors further emphasised that, while the 4% increase in airflow might seem insignificant, it corresponds to a clear increase of 18 L/s of fresh air. This quantity of fresh air could double the required fresh air ventilation for a single occupant, the authors concluded.
3.1.2 Phase change materials (PCM) related measures
According to the summary of the overall passive measures in Table 1 , from 1b to 1c, one can notice how PCM out-numbered the remaining passive measures. This stresses the importance that authors had given to the application of PCM on energy savings and thermal comfort. However, one limitation is that most investigations on PCM or PCM plus other measures are only based on the current or unspecified climate scenario (see Sect. 4 for more details). For example, without specifying any climate scenario (Chernousov & Chan, 2016 ; Meng et al., 2015 ; Rahimpoura, 2017 ; Saffari et al., 2016 ; Ur Rehman et al., 2021 ; Vega et al., 2022 ; Wu et al., 2018 ; Zeinelabdein et al., 2020 ; Zhao et al., 2019 ). Ur Rehman (Ur Rehman et al., 2021 ) compared corn husk composite material embedded with PCM, with standard brick construction, using a double-story school building located in Islamabad, Pakistan. The study found that 27 °C indoor comfort temperature was achieved without the need for mechanical cooling, and 31 °C was achieved for the standard brick construction. Meng et al., ( 2015 ) compared the fluctuation in indoor temperature for two identical hypothetical small rooms in China. One room without PCM and a new room with PCM (SP29 & RT18), and found that the new room with PCM can decrease the temperature fluctuation by 6.7 °C in summer and 12.7 °C in winter. Wu et al., ( 2018 ) used a couple of heat and moisture transfer (HAMT) models to test the energy-saving potentials of a phase change humidity control material (PCHCM) for different climate locations, but without specifying any climate period for the locations. After experimental validations of the base-case set-up, the study found that the maximal energy-saving potential rate of the PCHCM could be 19.57% for the Paris climate. Saffari et al., ( 2016 ) compared different PCM melting points (27 °C, 25 °C and 23 °C) against different HVAC operation schedules using a single zone base-case and also using the Madrid climate zone. For the 24 h HVAC operation schedule, the PCM with 27 °C melting point was found to achieve the highest annual energy savings of 10–15%, the 25 °C PCM melting point achieved 10–13% annual energy savings and the 23 °C PCM melting point achieved 8–10% annual energy savings. Considering the positioning of PCM in an office building in a generic climate of Hong Kong, Chernousov and Chan (Chernousov & Chan, 2016 ) considered its placement at the inner building envelope. This allows the PCM to reduce the morning peak power by up to about 5%. Rahimpour et al. ( 2017 ) devised the use of a 24 mm thick PCM in the roof, walls and floor as a demand response mechanism to reducing or shifting cooling and heating loads using three models; lightweight, brick-wall and PCM-enhanced lightweight buildings.. By using the CondFD algorithm, in the EnergyPlus platform, the results showed that the annual energy savings in Hobart and Melbourne (among the five cities analysed) are 21.8 and 16.7% respectively. Zeinelabdein ( 2020 ) investigates the potential of integrating PCM, as a Thermal Energy Storage (TES) with night cooling using computational fluid dynamics (CFD), and found the cooling system to meet 42% of the cooling load while also saving 67% of energy for the cooling requirement. By considering the parts of the works in the life cycle assessment of the integration of PCM in lightweight buildings conducted by Vega et al., ( 2022 ) in Chile, by also using the CondFD algorithm similar to (Rahimpoura, 2017 ), the findings showed that PCM integration in walls and roof decreases electric energy by 52.8% in Antofagasta and 36.3% in Calama. Zhao et al., ( 2019 ) investigated the integration of PCM with a solar heating system in a public building after studying the generic meteorological climate of Tibet. The study compared three schemes (‘A’, ‘B’ and ‘C’) of the solar heating system, and the results of the study concluded that the solar heating system, scheme ‘A’, integrated with the PCM led to 92% energy savings for a building requiring daytime (09.00 h-04.00 h) heating, and 64% energy savings for a building requiring 24 h full-time heating.
Contrary to the above studies, other studies only specified the current climate periods ( Thantonga, 2018 ; Al-Yasiri & Szabó, 2022 ; Gallardo & Berardi, 2022 ; Soudian & Berard, 2019 ; Zhou, 2022 ), for example, in a study by Gallardo and Berardi (Gallardo & Berardi, 2022 ), using an experimental base-case set-up, an experimental procedure was used to test the energy savings of a Radiant Panel Ceiling PCM (RCP-PCM) in Toronto, Canada. The study concluded that the RCP-PCM can shift cooling load to off-peak hours, and can absorb heat gain between 180 and 230 wh/m 2 . The study only compares the energy-saving potentials of the system for the current climate (September 2021). In the study by Zhou ( 2022 ), a dynamic transient dynamic platform was developed, integrating PCM walls with Building Integrated Photovoltaics (BIPVs). The results showed that due to solar shading, the vertical BIPVs may reduce building cooling load by 7.6% and peak power by 7.2%. The synergy of PCM walls and BIPVs could resist heat flux for a shorter period during summer time and provide heat for a longer period during winter time, with a higher magnitude in both cases when considering the current climate period. Al-Yasiri and Szabó ( 2022 ) investigated and compared the indoor temperature and heat gain reduction of two experimental setups (with and without PCM), in the current climate. The set-up with PCM proved to enhance the indoor temperature by 2 °C and reduce heat gain by 56W, compared to the set-up without PCM.
Similar to the night ventilation effect on PCM (Solgi et al., 2017 ; Zeinelabdein et al., 2020 ), and PCM integration with other measures ( Rahimpoura, 2017 ; Gallardo & Berardi, 2022 ; Zhao et al., 2019 ; Zhou, 2022 ); Soudian and Berard ( 2019 ), compared different ventilation flow rates (0, 2, 5 and 10 ACH) for night ventilation to test the effect of PCM integrated in walls and ceiling for the current climates of Toronto and New York in the summer. For the ventilation flow rate of 10 ACH, the cooling energy use intensity (EUI) was reduced by 19.7% and 7% in both Toronto and New York respectively, compared to the baseline without PCM. The results also claimed that the 10 AHC ventilation flow rate performed better. Thantong ( 2018 ) also compared the energy-saving potentials of two house models. One with a solar chimney PCM (SC-PCM), and the other with a simple concrete wall. The result showed that ventilation reduced the heat gain by 57.2% through the south wall with SC-PCM compared to the simple concrete wall.
Again, contrary to all the studies above on PCM which only considered current or generic climates, Sajjadian et al., ( 2015 ) assessed the potential of PCM on energy savings and thermal comfort of a near Passivhaus standard building in the UK, for current and future climate scenarios (2020, 2050, 2080). The results indicated that in the summer month of August 2080, cooling load savings of up to 128.1 kWh could be attained.
3.1.3 Insulation-related measures
Although, while investigations of some studies showed how insulations performed better as a passive solution than other passive strategies, others showed a contrast. For example, considering the current climate scenario, Calama-González et al., ( 2022 ) compared the performance of an externally insulated façade with a double skin façade (DSF) on the south and north façades of a building. The authors found that the double skin façade provides 67–85% comfort condition compared to the externally insulated façade with a comfort condition of 36–39%. However, since this study only considered the current climate, other different possibilities may arise for other future climate scenarios. Conversely, by considering the current and future climate scenarios, Guarda et al., ( 2019 ) compared the performances of typologies with rock wool, glass wool and Expanded Polystyrene (EPS) insulations against a base typology without any insulation. For the 2080 scenario, the authors confirmed that the base case typology can only achieve 17.9% comfort hours, while the rock wool, glass wool, and EPS typologies achieved 27.2%, 27.2% and 24.9%, respectively. The authors also noted that in all the climate scenarios, the glass wool and rock wool present the same performances. Again, without specifically relating to any climate scenario, Lingard ( 2021 ) confirmed the performance of solid wall insulation with low U-value glazing as a retrofitting option for the UK housing stock. The study found that retrofitting could reduce heat pump heating demand by 65%.
In a study on the impact of environment and energy of a net zero energy residential building, Cusenza et al., ( 2022 ) compared the performances of four design scenarios. The main outcome of the study showed that cellulose fibre insulation with BIPVs (with battery storage) has the lowest global energy requirement (GER), which is 18% lower than the expanded polystyrene (XPS) insulation with BIPVs (without battery storage). Contrary to the above study where integration of well insulation-based model designs gave rise to better energy savings, remarkable unprecedented results documented by Hugo et al., ( 2021 ) on the performance of a highly insulated model shows that, while there was about 0.73 °C decrease in average indoor temperature in the highly insulated model, the energy consumption increases by 3.5% when retrofitted with a roof top greenhouse (RTG) in the current climate scenario. In fact, the study further documented that by 2100, the energy consumption in the highly insulated model with the retrofit measure will rise up by 11% for the climate scenario of 2100. For a more continuous favourable performance of highly insulated buildings, the authors finally recommended an appropriate choice of RTG for any retrofit measure for the peculiar climate scenarios of Southern African cities. On further arguments on the performance of insulation-based measures against other passive measure, i.e., double-skin facade, contrary to the study in Sajjadian et al. ( 2015 ), Pierangioli et al., ( 2017 ) argued that an externally insulated system plus a high resistance window performed better in terms of energy savings than other passive measures in all cases including office buildings and two residential buildings. The results of the study showed a reduction of 64%, 67% and 70% energy savings for the two residential buildings and the office buildings respectively, for the future climate scenario of 2066–2095. In each case, the results demonstrated the comparison of the externally-insulated systems integrated in the buildings with the un-insulated base cases. Although this study considered the current and future climate contrary to Calama-González et al., 2022 , the reference climate is Central Italian and also does not consider a double skin façade as part of the compared passive measures.
3.1.4 Fenestration, roof and other related measures
Mahdy and Nikolopoulou ( 2014 ) compared the performances of combining different window to wall ratios (WWR) and glass types of window openings, in different climate zones in Egypt, under current and three future climate scenarios (2020, 2050, 2080). According to the findings of the investigation, by considering all the climate scenarios, < 10% WWR with a 6.4 mm glass reflective glass (having 8% stainless steel glass) could provide the best energy consumption (minimum) while maintaining better thermal comfort condition for all the climate scenarios. Similarly, Mahmoud et al., ( 2022 ) also investigated the performances of different glass types on energy consumption, although, in the cold climate of Canada and future climate scenarios were not considered. The results of the investigation showed that Double glass low E 6/6 mm Air gap is 18.63% better than the single clear reflective glass of the base-case yearly, in terms of energy consumption. Using a statistical Multiple Linear Regression model and a least-square estimate, Pajek et al., ( 2022 ), conducted a test of several passive design parameters to assess their relevance on energy consumption on heating, cooling and total energy consumption, across five countries in Europe (Ljubljana, Athens, Porto, Milan, Moscow) and under three future climate scenarios (2020, 2050, 2080). The assessments concluded that the U-value of the opaque envelope (thermal transmittance) was the most relevant parameter influencing the total energy consumption and the energy demand on heating, while the window-to-floor area (WFR) was the most relevant parameter for the cooling load. More so, in an earlier study, Pajek and Košir ( 2021 ) compared the performances of three building models with the same usable floor areas on energy savings and overheating for current and future climate scenarios, according to the Slovenian Energy Performance Certificate classification. According to the results of the study, the model with the best overall performance especially in view of the future climate scenario, meets the recommendation of the passive design parameters (Table 1 ), with the WFR at 15%.
Cirrincion et al., ( 2021 ) compared the effect of green roofs (GRs) installed on buildings and standard buildings without GRs, with respect to climate change and demographic growth, in the cities of Italy and Luxembourg. The effects of the GRs showed a 20–50% and 3–15% reduction in energy savings in Esch-sur-Alzette and Palermo respectively. For indoor comfort, the results of the study further showed that, with the presence of the GRs, there was a shift in the thermal sensation of PMV from an acceptable-moderate level (PMV ≥ 0.5) to a moderate-normal level (PMV ≤ 0.5). In contrast, Cascone ( 2022 ) only consider the current climate by comparing the performance of a bio-based GR (recycled polyethene granules) with a commercially traditional one in the Mediterranean climate in Italy. By using experimental set-up and measurements with sensors, the author detailed that the bio-based GR led to a reduction of 2 °C in the indoor surface temperature as compared to the traditional roof, as well as a corresponding reduction in energy consumption. Similarly, Sabati et al., ( 2020 ) also considered the current climate scenario and used an experimental set-up involving heat flux sensors and thermocouples, to compare the performance of rooftop greenery systems (RTGS) with non-green rooftops in a sub-tropical climate in Australia. Based on the comparison, the cooling load result using the DesignBuilder simulation showed that a reduction of 13.5% in energy could be saved with RTGS. By considering the integration of GR with other passive measures as retrofitting intervention, Sartor and Calmon ( 2019 ), in a generic climate in Brazil, have shown that the overall performance of all the measures on a neighbourhood building achieved a 15% reduction in energy consumption. However, in this case, the impact of the GR is very minute, contributing to only a 0.2% reduction, while the ventilated façade had the highest significance, contributing to about 10%. Andric et al., ( 2020 ) were the first and only authors to study the energy performance of green systems (on roofs and walls) for the current and future climate in Qatar. The authors modelled a two-story residential building to serve as a representative building stock of Qatar (2020, 2050, 2080). After documenting the projected energy consumption of the building without mitigation measures (increase by up to 9%, 17%, and 30% in 2020, 2050, and 2080, respectively), the study concluded that integrating a 50 mm XPS and the installation of energy-efficient windows (30% energy savings) proved more energy efficient than the addition of green systems on walls and roofs (3% energy savings) under the climate condition. Although, this study contradicts others on the performance of green systems.
Away from green roof systems, cool roofs, based on their albedo parameter, have also shown promise in reducing energy consumption and enhancing comfort. For example, Pokhrel et al., ( 2019 ) explored the combination of active and passive building energy sustainability and mitigating options, using the weather research and forecast (WRF) model, with the building effect parameterization and building energy model (BEP-BEM) schemes, under the current climate. Based on the mitigating options explored, the findings showed that the cool roof, control of indoor temperature set-point and the use of efficient air-conditioning (AC) equipment reduced the total energy demand by 6.9%, 9.9% and 16.15%, respectively. When simultaneously considering all options, the result also showed that the total energy could be reduced by 40%. Again, in the study conducted by Kolokotroni et al., ( 2013 ), on the impacts of different albedo values for the cool roof, and under the current climate scenario, the simulation results showed that the realistic optimum albedo value to achieve about 3–6% overall heating and cooling reduction is 0.6–0.7. Together with this value, the authors also recommended an air change rate of 2ACH. On the contrary, Ortiz et al., ( 2022 ), instead suggested that a reflective roof with an albedo of 0.89 combined with AC set-point adjustment could lead to a 20% reduction in cooling energy demand in the highest-burden neighbourhoods in New York, under a warming climate. The results of this study were based on the current and future climate scenarios, using the Weather Research and Forcast (WRF) model.
In a study on the impact of air infiltration rate on heating demand, Liu et al., ( 2021 ) first conducted a comprehensive field investigation into the space heating performance of airport terminals across various cities in China. Through the potential of the adaptive passive strategy of adjusting air tightness, the study concluded that adjusting the air tightness at the top and bottom openings against severe infiltration rate, as well as using radiant floor heating could jointly lead to 84% energy savings on heating. Because the investigation in this study was conducted between 2012 and 2019, it is important to note that this study only focused on the current situation of the higher energy consumption for space heating in airport terminals.
Among some studies which used emerging control systems, Lucchino and Goia ( 2023 ) most recently used a multi-domain model-based control (MBC) algorithm for an adaptive façade based on a flexible double skin façade (DSF). Although, the study only considered the current climate for three seasonal periods (winter, summer and mid-summer). By using a Building energy simulation tool (BES) to co-simulate with the MBC to select the best DSF, the findings showed that the MBC could save 70% of energy while simultaneously maintaining occupied comfort hours > 80% (falling within the selected comfort criteria for all the domains). The authors claimed that these results are when comparing the MBC with other traditional control systems, i.e., schedule and rule-based controls. One can say the outcome of this study could not have been better emphasized if any future climate scenario had been adopted to test the performance of the MBC on the DFS. In the study, the authors considered four domain priorities (illuminance on the working plane, \({\overline{E}}_{plane}\) ; CO2 concentration in the room, \(\Delta \) CO2; operative temperature in the room,T op ; as well as the heating and cooling demand, \(\left|{Q}_{heat}\right|+ \left|{Q}_{cool}\right|\) ) to check the performance of the adaptive DSF. Based on the domains, the controlled MBC optimisation problem on the performance of the DSF coupled with the HVAC system was formulated by the authors in Eqs. ( 11 ) and ( 12 ) thus:
where: \(\left|{Q}_{heat}\right|+ \left|{Q}_{cool}\right|\) is the sum of heating and cooling demand; \(OCC\) is the presence of the occupants; \(AFP\) is the possible airflow paths; \(OP\) is the position of the openings; \(F\) : is the fan settings (operation and flow); \(SH\) is the shading position; \(\varphi \) is the angle of the slats of the DSF.
Additionally, the four domains were subject to the following constraints:
Threshold values of 1st and 2nd LEVEL in Eq. ( 12 ) are presented in Table 2 based on the authors’ assumptions:
For studies on building layout and form as effective passive measures, Ahmadian et al., ( 2022 ) developed an optimisation framework to identify the most energy-efficient built form and urban geometry for the future built environment which can adapt to the changing climate scenarios. Among the four built forms optimised, compared to the pavilion built form (least efficient), the tunnel-court form (most efficient) could lead to an energy demand of 45KWh/m 2 by 2050, and the pavilion could lead to an energy demand of 100WKh/m 2 by 2050.
Although the authors did not recommend any effective passive or active solution, Lei et al., ( 2022 ) projected the effect of climate change on thermal comfort in heritage apartments in both hot summer and cold climate zones in China, under the current (TMY) and future (2050) climate scenario. The authors found that an increase of 58–60% in the predicted average overheating hours is likely by 2050, compared to 4–44% overheating hours under the current climate scenario. They concluded that some climate change adaptation measures like solar shading, passive cooling and insulation, are currently studied in an ongoing study to mitigate the impact of overeating in view of any future climate scenario.
3.2 Category 2: effective active solutions
For PV-related active measures, Hyung An et al., ( 2018 ) compared the performances of heating and cooling loads of amorphous-Si (a-Si) building-integrated photovoltaic (BIPV) window (a-Si BIPV) and clear glass double-layer used in an office building in Korea for the current climate and concluded that the a-Si BIPV windows could reduce 18.2% energy demand on both cooling and heating compared to the double-layer windows. Italos et al., ( 2022 ) similarly conducted an energy performance analysis of an existing residential apartment by comparing two models. One with the integration of a double skin façade containing an effective active solar energy system (BIPVs), glazing system as well as rambling planting, and another model without. While this study focused on an unspecified climate scenario in Cyprus, parts of the results proved that the active solar energy system could cover the proposed energy consumption of the building by up to 63%. For the current and future climate, Kimiya Aram et al., ( 2022 ) also proved that the use of active PV solar systems in combination with other effective passive and active (efficient ACs) solutions, 65.14% and 86.18% energy consumption could be reduced in the current climate and future climate scenario of Iran. Also, the PV solar system could produce 90% of the building’s energy demand by 2080. Furthermore, for an existing mixed-mode building in Cyprus, and also without a specified climate scenario, Efthymiou et al., ( 2020 ), saw the possibility of using active solar BIPVs (along with their effective operation) as passive shading devices, as part of a double shell. While the other passive measures (80 mm polystyrene insulation, PVC double glazing) account for 85% and 50% reduction in heating and cooling load, the energy output from the BIPVs could cover the remaining 50% of the cooling load. Stockholm Chiu et al., ( 2013 ) investigated whether the design of a renewable energy system (RES) based on present-day data could lead to efficient energy performance in a future climate scenario, using a passive representative building in Tehran, Iran. Firstly, part of the study showed that the design of a RES with present energy data is vulnerable to the impact of CC. Secondly, using an optimisation technique concerning the influence of future CC scenario (2038), the summarily of the authors’ findings showed that the optimal RES (fit for the future climate scenario), decreased the annual electricity demand of the building by 2.29% between 2019 and 2038. The optimised RES share was also shown to cover the annual electricity demand shifts from 95.4% in 2019 to 102% in 2038, due to the growth of about 4.5% power generated by the PV systems, showing the reliability of the PV system over the long-term.
Perez-Andreu et al., ( 2018 ) conducted a comprehensive study for the current climate scenario (TMY2) and four temperature projections of two periods (2048–2052 and 2096–2100) under the following four climate-change scenarios: CNRM-CM5 RCP4.5, CNRM-CM5 RCP8.5, MPI-ESM-LR RCP4.5, and MPI-ESM-LR RCP8.5, in Valencia, Spain. The authors developed eight models based on a typical Mediterranean residential building to reflect a combination of different passive and active measures. Among all the models (including the base model), ‘model 8’, where all combinations of passive measures plus heat recovery system, as well as extra mechanical ventilation, were implemented, irrespective of climate scenario, the energy savings of at least 80% could be achieved in 2048–2052. Again, the authors’ results showed that, in model 8, at least 65% energy savings could be achieved in the 2096–2100 period. The study also separately mentioned the contribution of adding the heat recovery system and the mechanical ventilation in model 8 to have 19% energy savings in the (MPI-ESM-LR RCP 8.5 scenario) in the 2096–2100 period, compared to ‘model 7’ (with no extra mechanical ventilation).
Sobhani et al., ( 2020 ) considered the potential of active free cooling Latent heat thermal energy storage (LHTES) to mitigate the cooling requirement of the passive house which may be subject to overheating in summer. LHTES systems use night cooling to provide solutions for sustainable cooling with the use of renewable cooling sources (Stockholm Chiu et al., 2013 ). Under the climate of Stockholm for which the scenario was not specified by the author, and through a multi-objective optimisation on cooling supply for various LHTES, the authors concluded that 75% of the cooling requirement could be met with the optimised LHTES.
Similar to the optimisation technique using NSGA-II (Andric et al., 2020 ), Mostafazadeh et al., ( 2023 ) introduce a novel simulation-based multi-objective optimisation approach by modifying the NSGA-III algorithm. This is the first proposed novel modified algorithm (prNSGA-III) to optimise active and passive energy retrofit measures under future climate change scenarios. Based on the optimised implemented retrofit measures (see Table 1 f) of a residential building in Tehran, Iran, about up to 46% thermal discomfort can be reduced in the future climate scenario. The authors also emphasised the promising potential of the prNSGA-III in optimisation techniques for retrofit measures in other various future climate zones and scenarios. The multi-objective optimisation function of the prNSGA-III was formulated by the authors through the thermal comfort objective function as follows:
where \(TDH\) = thermal discomfort hours; \(DOH\) =annual discomfort hours; \(OH\) =occupied hours.
The multi-objective optimisation is thus:
Subject to:
where \({EP}_{0}\) and \({TDH}_{0}\) are environmental performance and thermal discomfort hours of the baseline building (representing the two core constraints of the multi-objective optimization); \(LCC\) is the life cycle cost; \(x\) is the vector of decision variables representing a specific retrofit strategy; and \(X\) is the feasible set of decision vectors.
For the above multi-objective pareto optimisation, the prNSGA-III developed by the authors operates such the best retrofit measures are selected to maximise the EP and LCC and to minimise the TDH.
Likewise, Luo and Oyedele ( 2022 ) deployed a novel hybrid-genetic algorithm and artificial neural network (GA-ANN) for optimisation purposes to select the optimal retrofit measures under changing future climate scenarios, implemented on two campus buildings (Northavon house and Q building) in Bristol, UK. The authors found that selected retrofit measures from current climate conditions may no longer work under future climate change scenarios, with at most 4.7% over estimation or 54.7% underestimation of energy, lifetime cost and carbon. Using the hybrid novel optimisation technique (GA-ANN), the authors recommended effective retrofit active and passive measures (see Table 1 f).
Some studies reported the effectiveness of heat pumps, and the performance of some other active measures depending on the climate scenarios, for example, Rahif et al., ( 2022 ), conducted a time-integrated discomfort assessment for a base case of nearly zero energy dwelling, with no active free cooling in Brussels under current and future climate scenario. The authors first found that up to a 528% increase in overheating risk and about a 32% decrease in undercooling risk are projected by the end of the century. By comparing the performances of active systems, a reversible air-to-water heat pump (S02) could save HVAC energy consumption to about 6–13% compared to a gas-fired boiler + an air conditioner (S01). Secondly, the sensitivity analysis of the study showed that HVAC strategy, heating set-point, and cooling set-point are among the most influential parameters determining the HVAC primary energy use.
For the performance of active measure emission scenarios, Masi et al., ( 2021 ) conducted a sensitivity analysis by the use of modified weather files based on the representative concentration pathways (RCP) of the fifth assessment of the IPCC report, to determine the influence of climate change on photovoltaic productivity. The findings showed that climate change may bring an increase in the productivity of the photovoltaic from 3.3 KWp to 6.9 KWp peak power for the worst scenario emission, leading to a decrease in electricity demand from 28 to 17%. Similarly, Kharseh et al., ( 2015 ) investigated the impact of global climate change on the performance of GSHP systems in different climate zones (Stockholm, Doha, Istanbul). From the findings of the study, for a building with a standard thermal quality of building envelope (TQBE) as classified by the author, the GSHP annual energy consumption is projected to decrease by 5% (Stockholm) in cold climate and increase by 17.7% (Doha) in hot climate in 2050 compared to 2014. Equivalently, for a building with a low TQBE, the GSHP is projected to decrease by 8.5% in cold climates and increase by 18% in hot climates. Evidently, it can be said that the GCC has a significant impact on the performance of GSHP. This was also corroborated in the study by Shen and Lukes ( 2015 ), where the increase in global warming by 2–3 °C reduces the annual performance of GSHP in future climate scenarios for residential buildings, in the four USA cities under consideration. In fact, the study concluded that for this reason, there may be less competitiveness in the choice of GSHP in the future warming in the climate context of the USA.
4 Discussion
4.1 analysis of studies and critique.
From the previous section, it can be noticed that the number of studies dealing with effective climate adaptive or retrofitting or passive and active as well as renewable measures to the built environment, especially under different future climate scenarios, has increased from the past 5 years until 2023 compared to years that preceded. However, based on the summary in Table 1 , one can notice that some studies only consider any effective measure under the Current Scenario (herein onward referred to as TMY), and even more studies failed to clearly specify whether any investigation was under TMY/Future Climate Scenario. TMY/Future Climate Scenario is herein onward referred to as TMY/FCS. Therefore, based on intuition, they are considered in such studies as ‘Generic’ in this paper.
It would be worthwhile to compare studies strictly on all effective active and passive measures based on reckoning (see Table 3 ) and currency (see Fig. 5 ). Although, other studies were a combination of any active and passive measures. Again, with reference to Fig. 5 , it is important to clarify that ventilation means it was the most sensitive passive measure against any other passive measure combined with it in the papers, thereby, the category was simply termed ventilation in the figure. This was a similar case with PCM, insulation, fenestration and GRs/cool roofs in the same figure. For simplicity, the Generic climate studies are merged with the MTY studies in Fig. 6 . By comparison of these studies to similar ones, impacts of climate adaptive measures could be very much extended under the TMY/FCS but were not. For example, while some studies relating to measures on ventilation effectiveness and overheating comprehensively projected the impacts and sensitivity of these measures under the TMY/FCS (Andric et al., 2018 , 2019 , 2020 ; Cui et al., 2022 ; Díaz-Lopez & Serrano-Jiménez et al., 2022 ; Fereidani et al., 2021 ; Mehmood et al., 2022 ; Nair et al., 2022 ; Ozarisoy & Altan, 2021 ; Perez-Andreu et al., 2018 ; Rañeses et al., 2021 ; Soaresa et al., 2017 ; Zhou et al., 2020a , 2020b ), others were only restricted to the TMY or the Generic climate (Andric et al., 2018 , 2020 ; Cui et al., 2022 ; Díaz-Lopez & Serrano-Jiménez et al., xxxx; Fereidani et al., 2021 ; Nunes & Giglio, 2022 ; Perez-Andreu et al., 2018 ; Zhou et al., 2020a ). Similarly, for studies relating to PCM (or PCM in combination with other measures) and their impacts on energy savings, only one study (Meng et al., 2015 ) comprehensively considered the TMY/FCS, although, the year of the study was in 2015 (the study clearly shown in the boxplot in Fig. 5 ), while the remaining PCM related studies reported, only considered the TMY or any Generic climate (Botti & Ramos, 2017 ; Costanzoa et al., 2018 ; Hamdy et al., 2017 ; Heracleous et al., 2021 ; Hooff et al., 2014 ; Laouadi et al., 2020 ; Lapisa et al., 2018 ; Miri & Babakhani, 2021 ; Pelletier & Calautit, 2021 ; Roetzel et al., 2013 ; Sandaruwan et al., 2022 ; Solgi et al., 2017 ; Ur Rehman et al., 2021 ; Wang et al., 2017 ; Yildiz, 2015 ).
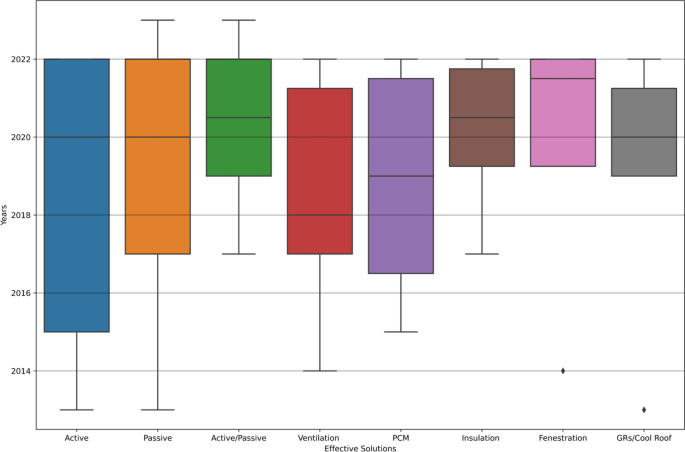
Boxplot showing effective solutions against the year of publication
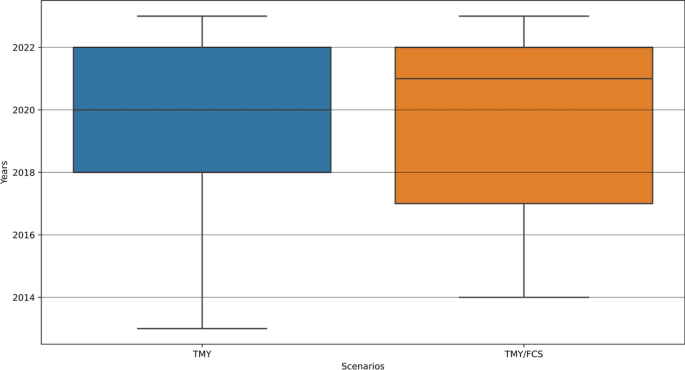
Boxplot showing studies based on climate scenarios against the year of publication
This means that more comprehensive studies on the impacts of PCM under future emission scenarios are lacking and could be explored. Again, among studies relating to insulations plus other climate adaptive measures, only (Edenhofer et al., 2014 ; Saffari et al., 2016 ; Zeinelabdein et al., 2020 ) considered the TMY/FCS while their Counterpart (Chernousov & Chan, 2016 ; Faccani et al., 2017 ; Wu et al., 2018 ) only considered the TMY or any Generic climate. Other studies combined effective measures as either ventilation through fenestrations (windows) plus any other measure(s), thermal transmittance of fenestrations plus any other measure(s), or shading devices of fenestrations plus any other measure(s). Three of these studies (Gallardo & Berardi, 2022 ; Vega et al., 2022 ; Zhou, 2022 ), considered the TMY/FCS while only a study (Zhao et al., 2019 ) was based on the TMY. On effective measures pertaining GRs or albedos of cool roofs, only three studies (Al-Yasiri & Szabó, 2022 ; Lingard, 2021 ; Toe & Kubota, 2015 ) were done under TMY/FCS, and the others ( Thantonga et al., 2018 ; Calama-González et al., 2022 ; Guarda et al., 2019 ; Sajjadian et al., 2015 ; Soudian & Berard, 2019 ) under just the TMY. Some most recent studies have shown rewards of emerging optimisation techniques (Bayesian Bootstrap, NSGA-II, prNSGA-III, GA-ANN, MBC) to optimising adaptive passive/actives under TMY/FCS (Andric et al., 2020 ; Cirrincion et al., 2021 ; Kolokotroni et al., 2013 ; Pokhrel et al., 2019 ; Rañeses et al., 2021 ), although one study only considered the TMY (Hugo et al., 2021 ). To the best of our knowledge, there is yet any study on the robustness of Model Predictive Control (MPC) in optimising any climate adaptive measures (for example, innovative involutions and PCM) based on future climate scenarios. This emerging field of optimisation techniques could be explored further.
5 Conclusion and area of future study
This paper started by re-emphasising the glaring threats of the future warming climate scenarios through evidence from the recent IPCC reports. After, it reviewed up-to-date reports on effective active and passive solutions to mitigate such threats from past studies. The following are the key conclusions of this paper:
By geography, there is a paucity of studies in Africa within the scope of this paper, with only three reports recorded (Hooff et al., 2014 ; Vega et al., 2022 ; Zeinelabdein et al., 2020 ). This could potentially be an area to look into in future research.
Among the EU and UK papers with Mediterranean to oceanic and temperate climates, for both housing dwellings and commercial buildings, the most effective solutions are natural ventilation/ventilative cooling. In almost all cases of these instances, future climate scenarios were considered.
In subtropical to hot regions in Asia, the most effective solution is the PCM in public buildings. However, not all the papers fully considered the future climate scenarios.
In most of the Middle-East regions with semi-arid to arid climates, ventilation/windcatchers and PV are the most effective solutions. While some considered the future climate scenarios, others do not.
In different regions across the US and Canada for both public buildings and households, the most effective solutions are any exterior shading, low E double glass, PCM and reflective roof. Only future climate scenarios are discussed in these regions.
In South American regions, the notable effective solutions are Insulation, envelope thermal capacity and ventilation and PCM, with few studies having focused on future climate scenarios.
Among the papers on Australia having a sub-tropical to hot-humid climate, the notable most effective solutions are green roofs for office buildings and PCM for residential buildings. However, none considered the future climate scenario.
This paper only utilizes data from the Scopus database, future research could expand the database using the same method.
Data availability
The data used to support the findings of this study are included within the article.
Ahmadian, E., Bingham, C., Elnokaly, A., & Sodagar, V. I. (2022). Impact of climate change and technological innovation on the energy performance and built form of future cities. Energies, 15 , 8592. https://doi.org/10.3390/en1522859
Article Google Scholar
Al-Yasiri, Q., & Szabó, M. (2022). Building envelope integrated phase change material under hot climate towards efficient energy and CO 2 emission saving. In 7th international conference on smart and sustainable technologies (SpliTech) 2022. https://doi.org/10.23919/SpliTech55088.2022.9854246
Ambade, B., Kumar, A., & Latif, M. (2021). Emission sources, characteristics and risk assessment of particulate bound polycyclic aromatic hydrocarbons (PAHs) from traffic sites. Research Square. https://doi.org/10.21203/rs.3.rs-328364/v1
Ambade, B. (2016). Characterization of PM 10 over urban and rural sites of Rajnandgaon, central India. Natural Hazards, 80 , 589–604. https://doi.org/10.1007/s11069-015-1985-2
Ambade, B., Kumar, S. T., Lokesh, K. S., & Chandra, D. U. (2022). Understanding sources and composition of black carbon and PM 2.5 in urban environments in East India. Urban Science . https://doi.org/10.3390/urbansci6030060
Ambade, B., Kumar, T. S., Kumar, T., & Shankar, S. S. (2021a). Characterization of PAHs and n-Alkanes in Atmospheric Aerosol of Jamshedpur City, India. Journal of Hazardous, Toxic, and Radioactive Waste . https://doi.org/10.1061/(ASCE)HZ.2153-5515.0000490
Andric, I., Fournier, J., Lacarriere, B., Le Corre, O., & Ferrao, P. (2018). The impact of global warming and building renovation measures on district heating system techno-economic parameters. Energy, 150 , 926–937. https://doi.org/10.1016/j.energy.2018.03.027
Andric, I., Kamal, A., & Al-Ghamdi, S. G. (2020). Efficiency of green roofs and green walls as climate change mitigation measures in extremely hot and dry climate: Case study of Qatar. Energy Reports, 6 , 2476–2489. https://doi.org/10.1016/j.egyr.2020.09.006
Andric, I., Koc, M., & Al-Ghamdi, S. G. (2019). A review of climate change implications for built environment: Impacts, mitigation measures and associated challenges in developed and developing countries. Journal of Cleaner Production, 211 , 83–102. https://doi.org/10.1016/j.jclepro.2018.11.128
Aslam, R. W., Shu, H., Yaseen, A., Sajjad, A., & Zain, S. U. (2023). Identification of time-varying wetlands neglected in Pakistan through remote sensing techniques. Environmental Science and Pollution Research, 30 , 74031–74044. https://doi.org/10.1007/s11356-023-27554-5
Bass, B., & New, J. (2023). How will United States commercial building energy use be impacted by IPCC climate scenarios? Energy, 263 , 125945. https://doi.org/10.1016/j.energy.2022.125945
Botti, A., & Ramos, M. (2017). Adapting the design of a new care home development for a changing climate. International Journal of Building Pathology, 35 , 417–433. https://doi.org/10.1108/IJBPA-11-2016-0028
Calama-González, C. M., Leon-Rodríguez, L. A., & Suarez, R. (2022). Assessing thermal comfort in the Mediterranean social housing stock through test cells: comparison of double-skin, externally insulated and non-retrofitted facades. Case Studies in Thermal Engineering, 38 , 102369. https://doi.org/10.1016/j.csite.2022.102369
Cardinale, N., Rospi, G., & Stefanizzi, P. (2013). Energy and microclimatic performance of Mediterranean vernacular buildings: the Sassi district of Matera and the Trulli district of Alberobello. Building and Environment, 59 , 590–598. https://doi.org/10.1016/j.buildenv.2012.10.006
Cascone, S. (2022). The energy-efficient design of sustainable green roofs in Mediterranean climate: An experimental study. Energy and Buildings, 273 , 112427. https://doi.org/10.1016/j.enbuild.2022.112427
Chernousov, A. A., & Chan, Y. B. B. (2016). Numerical simulation of thermal mass enhanced envelopes for office buildings in subtropical climate zones. Energy and Buildings, 118 (214), 225. https://doi.org/10.1016/j.enbuild.2016.02.054
Chiu, J. N. W., Gravoille, P., & Martin, V. (2013). Active free cooling optimization with thermal energy storage in Stockholm. Applied Energy, 109 , 523–529. https://doi.org/10.1016/j.apenergy.2013.01.076
Cirrincion, L., Marvuglia, A., & Scaccianoce, G. (2021). Assessing the effectiveness of green roofs in enhancing the energy and indoor comfort resilience of urban buildings to climate change: Methodology proposal and application. Building and Environment, 205 , 108198. https://doi.org/10.1016/j.buildenv.2021.108198
Clarke, L. E., Edmonds, J. A., Jacoby, H. D., Pitcher, H., Reilly, J. M., & Richels, R. (2007). Scenarios of greenhouse gas emissions and atmospheric concentrations. Sub-report 2.1a of synthesis and assessment product 2.1. Climate Change Science Program and the Subcommittee on Global Change Research, Washington DC. http://www.climatescience.gov/Library/sap/sap2-1/finalreport/default.htm
Costanzoa, V., Fabbrib, K., & Piraccini, S. (2018). Stressing the passive behavior of a Passivhaus: An evidence-based scenario analysis for a Mediterranean case study. Building and Environment, 142 , 265–277. https://doi.org/10.1016/j.buildenv.2018.06.035
Cui, C., Raslan, R., Korolija, I., & Chalabi, Z. (2022). On the robustness of thermal comfort against uncertain future climate: A Bayesian bootstrap method. Building and Environment, 226 , 109665. https://doi.org/10.1016/j.buildenv.2022.109665
Cusenza, M. A., Guarino, F., Longo, S., & Cellura, M. (2022). An integrated energy simulation and life cycle assessment to measure the operational and embodied energy of a Mediterranean net zero energy building. Energy and Buildings, 254 , 111558. https://doi.org/10.1016/j.enbuild.2021.111558
da Guarda, E. L. A., Gabriel, E., Domingos, R. M. A., Durante, L. C., Callejas, I. J. A., Sanches, J. C. M., & Rosseti, K. D. C. (2019). Adaptive comfort assessment for different thermal insulations for building envelope against the effects of global warming in the mid-western Brazil. IOP Conference Series: Earth and Environmental Science, 32 , 012057. https://doi.org/10.1088/1755-1315/329/1/012057
De Masi, R. F., Festa, V., Gigante, A., Mastellone, M., Ruggiero, S., & Vanoli, G. P. (2021). Effect of climate changes on renewable production in the mediterranean climate: Case study of the energy retrofit for a detached house. Sustainability, 13 , 8793. https://doi.org/10.3390/su13168793
Díaz-Lopez, C., Serrano-Jiménez, A., Lizana, J., Lopez-García, E., Molina-Huelva, M., & Barrios-Padura, A. (2022). Passive action strategies in schools: A scientific mapping towards eco-efficiency in educational buildings. Journal of Building Engineering, 45 , 103598. https://doi.org/10.1016/j.jobe.2021.103598
Edenhofer, O., Pichs-Madruga, R. Y., Sokona, E., Farahani, S., Kadner, K., & Seyboth, A., et al. (2014). IPCC, 2014: Summary for policymakers. In: Climate change 2014: Mitigation of climate change. contribution of working group III to the fifth assessment report of the intergovernmental panel on climate change . Cambridge University Press, Cambridge.
Efthymiou, M., Kontonis, P. R., Leonidou, A., Kazanakis, G., & Vassiliades, C. (2020). Investigation of sun protection issues via the active and passive building integration of active solar energy systems: A case study of the renovation of an existing building in Cyprus. IOP Conference Series: Earth and Environmental Science, 410 , 012063. https://doi.org/10.1088/1755-1315/410/1/012063
Fereidani, N. A., Rodrigues, E., & Gaspar, A. R. (2021). A review of the energy implications of passive building design and active measures under climate change in the Middle East. Journal of Cleaner Production, 305 , 127152. https://doi.org/10.1016/j.jclepro.2021.127152
Fujino, J., Nair, R., Kainuma, M., Masui, T., & Matsuoka, Y. (2006). Multigas mitigation analysis on stabilization scenarios using aim global model. The Energy Journal, 27 , 343–354.
Gallardo, A., & Berardi, U. (2022). Experimental evaluation of the cooling performance of radiant ceiling panels with thermal energy storage. Energy and Buildings, 262 , 112021. https://doi.org/10.1016/j.enbuild.2022.112021
Hamdy, M., Carlucci, S., Hoes, P., & Hensen, J. L. M. (2017). The impact of climate change on the overheating risk in dwellings: A Dutch case study. Building and Environment, 122 , 307–323. https://doi.org/10.1016/j.buildenv.2017.06.031
Heracleous, C., Michael, A., Savvides, A., & Hayles, C. (2021). Climate change resilience of school premises in Cyprus: An examination of retrofit approaches and their implications on thermal and energy performance. Journal of Building Engineering, 44 , 103358. https://doi.org/10.1016/j.jobe.2021.103358
Herrera-Gomez, S. S., Quevedo-Nolasco, A., & Pérez-Urrestarazu, L. (2017). The role of green roofs in climate change mitigation, a case study in Seville (Spain). Building and Environment, 123 , 575–584. https://doi.org/10.1016/J.BUILDENV.2017.07.036
Hijioka, Y., Matsuoka, Y., Nishimoto, H., Masui, T., & Kainuma, M. (2008). Global GHG emission scenarios under GHG concentration stabilization targets. Journal of Global Environmental Engineering, 13 , 97–108.
Google Scholar
Hugo, J., Plessis, C., & Masenge, A. (2021). Retrofitting Southern African cities: A call for appropriate rooftop greenhouse designs as climate adaptation strategy. Journal of Cleaner Production, 312 , 127663. https://doi.org/10.1016/j.jclepro.2021.127663
Hussain, A. J., Sankar, T. K., & Vithanage, M. (2023). Black carbon emissions from traffic contribute sustainability to air pollution in urban cities of India. Water, Air, and Soil Pollution, 234 , 217. https://doi.org/10.1007/s11270-023-06232-9
Article CAS Google Scholar
Hyung An, J., Yoon, J. H., An, Y. S., & Heo, E. (2018). Heating and cooling performance of office buildings with a-Si BIPV windows considering operating conditions in temperate climates: The case of Korea. Sustainability, 10 , 4856. https://doi.org/10.3390/su10124856
IEA. (2018). The future of cooling. In: Opportunities for energy-efficient air conditioning . IEA Publications, Paris. https://www.iea.org/reports/the-future-of-cooling
IPCC. (2021). Climate change 2021: The physical science basis. In Contribution of working group I to the sixth assessment report of the intergovernmental panel on climate change . Cambridge University Press, Cambridge.
Italos, C., Patsias, M., Yiangou, A., Stavrinou, S., & Vassiliades, C. (2022). Use of double skin façade with building integrated solar systems for an energy renovation of an existing building in Limassol, Cyprus: Energy performance analysis. Energy Reports, 8 , 15144–15161. https://doi.org/10.1016/j.egyr.2022.11.088
Kharseh, M., Altorkmany, L., Al-Khawaja, M., & Hassani, F. (2015). Analysis of the effect of global climate change on ground source heat pump systems in different climate categories. Renewable Energy, 78 , 219–225. https://doi.org/10.1016/j.renene.2015.01.017
Kimiya Aram, K., Taherkhani, R., & Šimelyte, A. (2022). Multistage optimization toward a nearly net zero energy building due to climate change. Energies, 15 , 983. https://doi.org/10.3390/en15030983
Kolokotroni, M., Gowreesunker, B. L., & Giridharan, R. (2013). Cool roof technology in London: An experimental and modelling study. Energy and Buildings, 67 , 658–667. https://doi.org/10.1016/j.enbuild.2011.07.011
Laouadi, A., Bartko, M., & Lacasse, M. A. (2020). A new methodology of evaluation of overheating in buildings. Energy and Buildings, 226 , 110360. https://doi.org/10.1016/j.enbuild.2020.110360
Lapisa, R., Bozonnet, E., Salagnac, P., & Abadie, M. O. (2018). Optimized design of low-rise commercial buildings under various climates—energy performance and passive cooling strategies. Building and Environment, 132 , 83–95. https://doi.org/10.1016/j.buildenv.2018.01.029
Lei, M., van Hooff, T., Blocken, B., & Roders, A. N. (2022). The predicted effect of climate change on indoor overheating of heritage apartments in two different Chinese climate zones. Indoor and Built Environment, 31 , 1986–2006. https://doi.org/10.1177/1420326X221085861
Lingard, J. (2021). Residential retrofit in the UK: The optimum retrofit measures necessary for effective heat pump use. Building Services Engineering Research and Technology, 42 , 279–292. https://doi.org/10.1177/0143624420975707
Liu, X., Zhang, T., Liu, X., Li, L., Lin, L., & Jiang, Y. (2021). Energy saving potential for space heating in Chinese airport terminals: The impact of air infiltration. Energy, 215 , 119175. https://doi.org/10.1016/j.energy.2020.119175
Lucchino, E. C., & Goia, F. (2023). Multi-domain model-based control of an adaptive façade based on a flexible double skin system. Energy and Buildings, 285 , 112881. https://doi.org/10.1016/j.enbuild.2023.112881
Luo, X. J., & Oyedele, L. O. (2022). Life cycle optimisation of building retrofitting considering climate change effects. Energy and Buildings, 258 , 111830. https://doi.org/10.1016/j.enbuild.2022.111830
Mahdy, M. M., & Nikolopoulou, M. (2014). Evaluation of fenestration specifications in Egypt in terms of energy consumption and long term cost-effectiveness. Energy and Buildings, 69 , 329–343. https://doi.org/10.1016/j.enbuild.2013.11.028
Mahmoud, S., Saad, M. M., Elshelfa, M., Abdelkhalik, H., & Fahmy, M. (2022). Comparative analysis of occupant thermal comfort, energy consumption, and CO 2 emissions for an educational building in cold climate: A case study in canada. IOP Conference Series: Earth and Environmental Science, 1056 , 012027. https://doi.org/10.1088/1755-1315/1056/1/012027
Mehmood, S., Lizana, J., Núnez-Peiro, M., Maximov, S. A., & Friedrich, D. (2022). Resilient cooling pathway for extremely hot climates in southern Asia. Applied Energy, 325 , 19811. https://doi.org/10.1016/j.apenergy.2022.119811
Meng, E., Yu, H., Liu, C., Sun, Z., & Zhou, B. (2015). Thermal performance of a new type of phase change material room in summer and winter. Energy Procedia, 121 , 2193–2200. https://doi.org/10.1016/j.proeng.2015.09.092
Meshram, S. G., Singh, S. K., & Meshram, C. (2018). Statistical evaluation of rainfall time series in concurrence with agriculture and water resources of Ken River basin, Central India (1901–2010). Theoretical and Applied Climatology, 134 , 1231–1243. https://doi.org/10.1007/s00704-017-2335-y
Miri, P., & Babakhani, P. (2021). On the failure of the only vernacular windcatcher in the mountainous region of Western Iran: Opportunities for energy-efficient buildings. Journal of Cleaner Production, 295 , 126383. https://doi.org/10.1016/j.jclepro.2021.126383
Moss, R. H., Edmonds, J. A., Hibbard, K. A., Manning, M. R., Rose, S. K., van Vuuren, D. P., Carter, T. R., et al. (2010). The next generation of scenarios for climate change research and assessment. Nature, 463 , 747–756. https://doi.org/10.1038/nature08823
Mostafazadeh, F., Eirdmousa, S. J., & Tavakolan, M. (2023). Energy, economic and comfort optimization of building retrofits considering climate change: A simulation-based NSGA-III approach. Energy and Buildings, 280 , 112721. https://doi.org/10.1016/j.enbuild.2022.112721
Nair, A. M., Wilson, C., Huang, M. J., Griffiths, P., & Hewitt, N. (2022). Phase change materials in building integrated space heating and domestic hot water applications: A review. Journal of Energy Storage, 54 , 105227. https://doi.org/10.1016/j.est.2022.105227
Naz, I., Ahmad, I., Waqar, R. A., Quddoos, A., & Yaseen, A. (2024). Integrated assessment and geostatistical evaluation of groundwater quality through water quality indices. Water, 16 , 23. https://doi.org/10.3390/w16010063
Nunes, G., & Giglio, T. (2022). Effects of climate change in the thermal and energy performance of low-income housing in Brazil—assessing design variable sensitivity over the 21st century. Renewable and Sustainable Energy Reviews, 168 , 112885. https://doi.org/10.1016/j.rser.2022.112885
Ortiz, L., Gamarro, H., Gonzalez, J. E., & McPhearson, T. (2022). Energy burden and air conditioning adoption in New York City under a warming climate. Sustainable Cities and Society, 76 , 103465. https://doi.org/10.1016/j.scs.2021.103465
Ozarisoy, B., & Altan, H. (2021). Systematic literature review of bioclimatic design elements: Theories, methodologies and cases in the South-eastern Mediterranean climate. Energy and Buildings, 250 , 111281. https://doi.org/10.1016/j.enbuild.2021.111281
Pajek, L., & Košir, M. (2021). Exploring climate-change impacts on energy efficiency and overheating vulnerability of bioclimatic residential buildings under Central European climate. Sustainability, 13 , 6791. https://doi.org/10.3390/su13126791
Pajek, L., Potocˇnik, J., & Košir, M. (2022). The effect of a warming climate on the relevance of passive design measures for heating and cooling of European single-family detached buildings. Energy and Buildings, 261 , 111947. https://doi.org/10.1016/j.enbuild.2022.111947
Pelletier, K., & Calautit, J. (2021). Analysis of the performance of an integrated multistage helical coil heat transfer device and passive cooling windcatcher for buildings in hot climates. Journal of Building Engineering, 48 , 103899. https://doi.org/10.1016/j.jobe.2021.103899
Perez-Andreu, V., Aparicio-Fernandez, C., Martínez-Ibernon, A., & Vivancos, J. (2018). Impact of climate change on heating and cooling energy demand in a residential building in a Mediterranean climate. Energy, 165 , 63–74. https://doi.org/10.1016/j.energy.2018.09.015
Pierangioli, L., Cellai, G., Ferrise, R., Trombi, G., & Bindi, M. (2017). Effectiveness of passive measures against climate change: case studies in Central Italy. Building Simulation, 10 , 459–479. https://doi.org/10.1007/S12273-016-0346-8
Pokhrel, R., Ramírez-Beltran, N. D., & González, J. E. (2019). On the assessment of alternatives for building cooling load reductions for a tropical coastal city. Energy and Buildings, 182 , 131–143. https://doi.org/10.1016/j.enbuild.2018.10.023
Rahif, R., Norouziasas, A., Elnagar, E., Doutreloup, S., Pourkiaei, S. M., Amaripadath, D., Romain, A., Fettweis, X., & Attia, S. (2022). Impact of climate change on nearly zero-energy dwelling in temperate climate: Time-integrated discomfort, HVAC energy performance, and GHG emissions. Building Environ, 223 , 109397. https://doi.org/10.1016/j.buildenv.2022.109397
Rahimpoura, Z., Faccani, A., Azuatalama, D., Chapmana, A., & Verbič, G. (2017). Using thermal inertia of buildings with phase change material for demand response. Energy Procedia, 121 , 102–109. https://doi.org/10.1016/j.egypro.2017.07.483
Rañeses, M. K., Chang-Richards, A., Wang, K. I., & Dirks, K. N. (2021). Housing for now and the future: a systematic review of climate-adaptive measures. Sustainabilty, 13 , 6744. https://doi.org/10.3390/su13126744
IPCC. (2014). Climate change 2014: Synthesis, 2014. In: Report contribution of working groups I, II and III to the fifth assessment report of the intergovernmental panel on climate change . IPCC, Geneva.
Riahi, K., Grübler, A., & Nakicenovic, N. (2007). Scenarios of long-term socio-economic and environmental development under climate stabilization. Technological Forecasting and Social Change, 74 , 887–935. https://doi.org/10.1016/j.techfore.2006.05.026
Roetzel, A., Tsangrassoulis, A., & Dietrich, U. (2013). Impact of building design and occupancy on office comfort and energy performance in different climates. Building and Environment, 71 , 165–175. https://doi.org/10.1016/j.buildenv.2013.10.001
Sabati, M., Rasul, M. G., Anwar, M., Khan, M. M. K., & Sedaghat, A. (2020). An experimental rooftop greenery setup in a subtropical/hot-humid climate for sustainable development and mitigation of climate change. EVER . https://doi.org/10.1109/EVER48776.2020.9242972
Saffari, M., Gracia, A., Ushak, S., & Cabeza, L. F. (2016). Economic impact of integrating PCM as passive system in buildings using Fanger comfort model. Energy and Buildings, 112 , 159–172. https://doi.org/10.1016/j.enbuild.2015.12.006
Sajjadian, S. M., Lewis, J., & Sharples, S. (2015). The potential of phase change materials to reduce domestic cooling energy loads for current and future UK climates. Energy and Buildings, 93 , 83–89. https://doi.org/10.1016/j.enbuild.2015.02.029
Sandaruwan, B. R. M., Siriwardana, C., Amaratunga, D., Haigh, R., & Robert, D. (2022). Climate change impacts on built environment: A systematic review. In 12th international conference on structural engineering and construction management: Proceedings of the ICSECM 2021 (pp. 443–459). https://doi.org/10.1007/978-981-19-2886-4_31
Sartor, T., & Calmon, J. L. (2019). Analysis of the impacts of retrofit actions on the life cycle energy consumption of typical neighbourhood dwellings. Journal of Building Engineering, 21 , 158–172. https://doi.org/10.1016/j.jobe.2018.10.009
Shen, P., & Lukes, J. R. (2015). Impact of global warming on performance of ground source heat pumps in US climate zones. Energy Conversion and Management, 101 , 632–643. https://doi.org/10.1016/j.enconman.2015.06.027
Shukla, P. R., Skea, J., Slade, R., Al Khourdajie, A., van Diemen, R., & McCollum, D., et al. (2022). IPCC, 2022: Summary for policymakers. In Climate change 2022: mitigation of climate change. Contribution of working group III to the sixth assessment report of the intergovernmental panel on climate change . Cambridge University Press, Cambridge. https://doi.org/10.1017/9781009157926.001 .
Smith, S. J., & Wigley, T. M. L. (2006). MultiGas forcing stabilization with minicam. The Energy Journal, 27 , 373–392.
Soaresa, N., Bastosa, J., Pereiraa, L. D., Soares, A., Amarala, A. R., Asadia, E., et al. (2017). A review on current advances in the energy and environmental performance of buildings towards a more sustainable built environment. Renewable and Sustainable Energy Reviews, 77 , 845–860. https://doi.org/10.1016/j.rser.2017.04.027
Sobhani, H., Shahmoradi, F., & Sajadi, B. (2020). Optimization of the renewable energy system for nearly zero energy buildings: A future-oriented approach. Energy Conversion and Management, 224 , 113370. https://doi.org/10.1016/j.enconman.2020.113370
Solgi, E., Kari, B. M., Fayazc, R., & Taheri, H. (2017). The impact of phase change materials assisted night purge ventilation on the indoor thermal conditions of office buildings in hot-arid climates. Energy and Buildings, 150 , 488–497. https://doi.org/10.1016/j.enbuild.2017.06.035
Soudian, S., & Berard, U. (2019). Assessing the effect of night ventilation on PCM performance in high-rise residential buildings. Journal of Building Physics, 43 , 229–249. https://doi.org/10.1177/1744259119848128
Thantonga, P., Khedari, J., & Chantawong, P. (2018). Investigation of thermal performance by applying a solar chimney with PCM towards the natural ventilation of model house under Climate of Thailand. Materials Today: Proceedings, 5 , 14862–14867.
Toe, D. H. C., & Kubota, T. (2015). Comparative assessment of vernacular passive cooling techniques for improving indoor thermal comfort of modern terraced houses in hot-humid climate of Malaysia. Solar Energy, 114 , 229–258. https://doi.org/10.1016/j.solener.2015.01.035
Ur Rehman, A., Ghafoor, N., Sheikh, S. R., Kausar, Z., Rauf, F., Sher, F., et al. (2021). A study of hot climate low-cost low-energy eco-friendly building envelope with embedded phase change material. Energies, 14 , 3544. https://doi.org/10.3390/en14123544
van Hooff, T., Blocken, B., Hensen, J. L. M., & Timmermans, H. J. P. (2014). On the predicted effectiveness of climate adaptation measures for residential buildings. Building Environment, 82 , 300–316. https://doi.org/10.1016/j.buildenv.2014.08.027
Van Vuuren, D. P., Eickhout, B., Lucas, P. L., & den Elzen, M. G. J. (2006). Long-term multi-gas scenarios to stabilise radiative forcing—exploring costs and benefits within an integrated assessment framework. The Energy Journal, 27 , 201–233.
Van Vuuren, D. P., Stehfest, E., Den Elzen, M. G. J., Deetman, S., Hof, A., Isaac, M., et al. (2011). RCP 2.6 : Exploring the possibility to keep global mean temperature change below 2 °C. Climatic Change, 109 , 95. https://doi.org/10.1007/s10584-011-0152-3
Vega, M., Llantoy, N., Chafer, M., Ushak, S., & Cabeza, L. F. (2022). Life cycle assessment of the inclusion of phase change materials in lightweight buildings. Journal of Energy Storage, 56 , 105903. https://doi.org/10.1016/j.est.2022.105903
Wang, L., Liu, X., & Brown, H. (2017). Prediction of the impacts of climate change on energy consumption for a medium-size office building with two climate models. Energy and Buildings, 157 , 218–226. https://doi.org/10.1016/j.enbuild.2017.01.007
Waqar, R. A., Shu, H., Javid, K., Pervaiz, S., Mustafa, F., Raza, D., Ahmed, B., Quddoos Al-Ahmadi, S., & Atef, W. H. (2024b). Wetland identification through remote sensing: Insights into wetness, greenness, turbidity, temperature, and changing landscapes. Big Data Research, 35 , 100416. https://doi.org/10.1016/j.bdr.2023.100416
Waqar, R. A., Shu, H., Tariq, A., Naz, I., Nasar, M. A., Quddoos, A., Javid, K., Mustafa, F., & Aeman, H. (2024a). Monitoring landuse change in Uchhali and Khabeki wetland lakes, Pakistan using remote sensing data. Gondwana Research, 129 , 252–267. https://doi.org/10.1016/j.gr.2023.12.015
Waqar, R., Shu, H., & Yaseen, A. (2023). Monitoring the population change and urban growth of four major Pakistan cities through spatial analysis of open source data. Annals of GIS, 29 , 355–367. https://doi.org/10.1080/19475683.2023.2166989
Wise, M., Calvin, K., Thomson, A., Clarke, L., Bond-Lamberty, B., Sands, R., et al. (2009). Implications of limiting CO 2 concentrations for land use and energy. Science, 324 , 1183–1186. https://doi.org/10.1126/science.1168475
WMO and WHO. (2015). Heat waves and health: Guidance on warning-system development . WMO and WHO. https://public.wmo.int/en/resources/library/heatwaves-and-health-guidance-warning-system-development
Wu, Z., Qin, M., & Zhang, M. (2018). Phase change humidity control material and its impact on building energy consumption. Energy and Buildings, 174 , 254–261. https://doi.org/10.1016/j.enbuild.2018.06.036
Yildiz, Y. (2015). Impact of climate change on passive design strategies. Engineering Sustainability . https://doi.org/10.1680/ensu.14.00044
Zeinelabdein, R., Omer, S., & Mohamed, E. (2020). Parametric study of a sustainable cooling system integrating phase change material energy storage for buildings. Journal of Energy Storage, 32 , 101972. https://doi.org/10.1016/j.est.2020.101972
Zhao, J., Yua, Y., Haghighat, F., Lu, J., & Feng, G. (2019). Investigation of energy performance and operational schemes of a Tibet-focused PCM-integrated solar heating system employing a dynamic energy simulation model. Energy, 172 , 141–154. https://doi.org/10.1016/j.energy.2019.01.125
Zhao, Z., Islam, F., Ali, L. S., Tariq, A., Nawaz, M., Islam, U. I., Bibi, T., Ur Rahman, N., Ahmad, W., Waqar, R. A., Raza, D., & Atef, W. H. (2023). Comparison of three machine learning algorithms using google earth engine for land use land cover classification. Rangeland Ecology and Management . https://doi.org/10.1016/j.rama.2023.10.007
Zhou, Y. (2022). Demand response flexibility with synergies on passive PCM walls, BIPVs, and active air-conditioning system in a subtropical climate. Renewable Energy, 199 , 204–255. https://doi.org/10.1016/j.renene.2022.08.128
Zhou, Y., Zheng, S., Liu, Z., Wen, T., Ding, Z., Yan, J., et al. (2020b). Passive and active phase change materials integrated building energy systems with advanced machine-learning based climate-adaptive designs, intelligent operations, uncertainty-based analysis and optimisations: A state-of-the-art review. Renewable and Sustainable Energy Reviews, 130 , 109889. https://doi.org/10.1016/j.rser.2020.109889
Zhou, Y., Zheng, S., & Zhang, G. (2020a). A review on cooling performance enhancement for phase change materials integrated systems—flexible design and smart control with machine learning applications. Journal of Building Engineering, 174 , 106786. https://doi.org/10.1016/j.buildenv.2020.106786
Download references
Author information
Authors and affiliations.
Faculty of Civil Engineering, Silesian University of Technology, Akademicka 5, 44-100, Gliwice, Poland
Ibrahim Tajuddeen
School of Computing, Engineering and the Built Environment, Edinburgh Napier University, Edinburgh, EH10 5DT, Scotland
Seyed Masoud Sajjadian
You can also search for this author in PubMed Google Scholar
Corresponding author
Correspondence to Seyed Masoud Sajjadian .
Ethics declarations
Conflict of interest.
The author declare that they have no conflict of interest.
Additional information
Publisher's note.
Springer Nature remains neutral with regard to jurisdictional claims in published maps and institutional affiliations.
Rights and permissions
Open Access This article is licensed under a Creative Commons Attribution 4.0 International License, which permits use, sharing, adaptation, distribution and reproduction in any medium or format, as long as you give appropriate credit to the original author(s) and the source, provide a link to the Creative Commons licence, and indicate if changes were made. The images or other third party material in this article are included in the article's Creative Commons licence, unless indicated otherwise in a credit line to the material. If material is not included in the article's Creative Commons licence and your intended use is not permitted by statutory regulation or exceeds the permitted use, you will need to obtain permission directly from the copyright holder. To view a copy of this licence, visit http://creativecommons.org/licenses/by/4.0/ .
Reprints and permissions
About this article
Tajuddeen, I., Sajjadian, S.M. Climate change and the built environment - a systematic review. Environ Dev Sustain (2024). https://doi.org/10.1007/s10668-024-04962-2
Download citation
Received : 16 May 2023
Accepted : 21 April 2024
Published : 11 May 2024
DOI : https://doi.org/10.1007/s10668-024-04962-2
Share this article
Anyone you share the following link with will be able to read this content:
Sorry, a shareable link is not currently available for this article.
Provided by the Springer Nature SharedIt content-sharing initiative
- Climate change
- Passive strategies
- Active strategies
- Built environment
- Find a journal
- Publish with us
- Track your research
Academia.edu no longer supports Internet Explorer.
To browse Academia.edu and the wider internet faster and more securely, please take a few seconds to upgrade your browser .
Enter the email address you signed up with and we'll email you a reset link.
- We're Hiring!
- Help Center
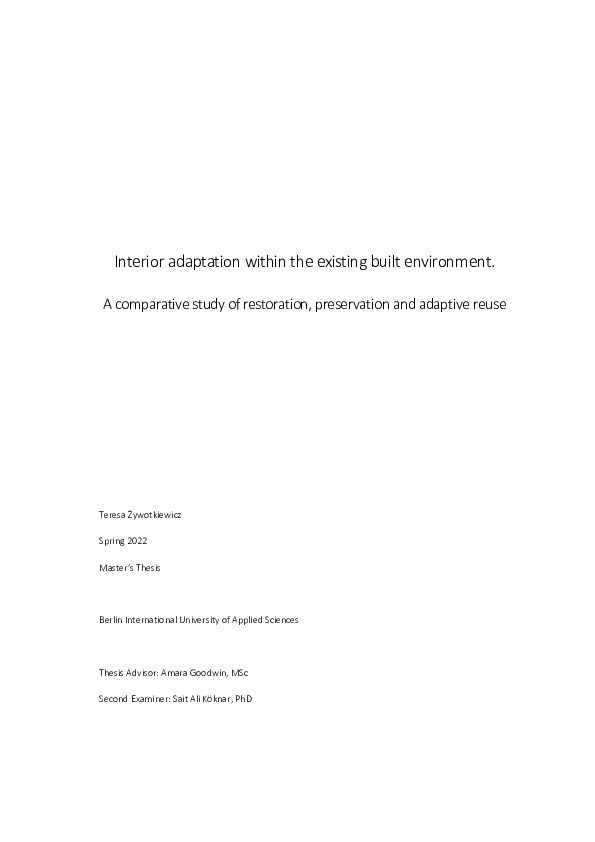
Thesis - Interior adaptation within the existing built environment. A comparative study of restoration, preservation and adaptive reuse

This thesis explores the fundamental aspects of interior architecture regarding the existing built environment. It focuses on the necessity of restoration, preservation and adaptive reuse as possible solutions when approaching a new project concerning an existing building. Through the study of books, current journals and relevant projects, a universal understanding is formed about approaches that can be used to react to the existing built environment. Also discussed are the more challenging situations that can arise, such as how to deal with heritage, contentious places or the concept of memory of place. Examples and case studies are worldwide to appeal to a greater audience. The thesis outlines and defines possibilities for repairing, restoring, and protecting the existing built environment while keeping them practical in the current time. Before concluding the thesis, a design project, done in conjunction with the thesis, is discussed and explores how the different approaches and interventions discussed can be used to react to a site based on the specific site’s history, value and intangible qualities.
Related Papers
Iran University of Science & Technology
Ehsan Masoud
Changing and repurposing existing buildings for their continued use was quite common in the past and structurally safe buildings were adapted to meet new functions and needs. In modern conservation theory, Adaptive Reuse is an important means of preserving cultural heritage. The main question is what are the priorities and shortcomings of adaptive reuse theoretical references within interior architecture based on comparative study with Nara Document parameters. The research method of this study is qualitative, with logical argument as a strategy. The priorities were studied and then the most important weaknesses and drawbacks of these approaches to Adaptive Reuse were analyzed in a comparative study with the Nara Grid by 32 semi-structured interviews with experts in the fields of Architecture, Interior architecture and conservation. The results show four main Adaptive Reuse priorities extracted from the reviewed literature: Host Space Function, Programmatic Approach to New Use, Tech...
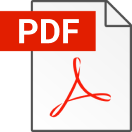
Inas Abdelsabour
Existing old buildings were considered as the city’s culture reminder. By the time, they could be kept via adaptation for contemporary usages. That adopting considered the context of the environmental, social and economic idea of the prior eras, related to the building’s life cycle, that guided by local solutions. This paper started with the adaptation process definition with an evaluation of a number of building’s state to bring out the different potential outcomes for discussing the future adaptation possibilities, especially that known as “alterations and extensions”. Therefore, by rethinking of the new addition’s integration into the heritage buildings will support the heritage value and fit new functions to present innovative design process approaches in the field of heritage preservation’s design. By analysing some case studies, the study achieved some criteria for designing the alterations and extensions by making them an effective component in the design of old heritage buil...
Ramola Lewis
6-page report giving an overview of the topic - completed for Bachelors degree in Architecture
Natascha Meuser
The architectural monuments of every society form a part of its cultural heritage and must be preserved for future generations. Today this process involves a range of complex challenges. The conservation of monuments entails not only assessing and evaluating those monuments but also engaging in a wide range of public relations activities. The aim of this course is therefore to help students gain a good working knowledge of architectural fundamentals as well as architectural history and theory. As such, this lecture series is divided into three broad modules: • History and Theory • Methods and Tools • Concepts and Projects During the course students will undertake a great deal of research, developing their own questions and viewpoints and gaining academic insights. They will work their way through academic tasks and apply what they have learnt to investigate a research question of their own choice.
REDUCE, REUSE, RETHINK AND PRESERVE: THE REUSE OF HISTORICAL BUILDINGS AS A STRATEGY FOR ENVIRONMENTAL SUSTAINABILITY AND HERITAGE APPRECIATION (Atena Editora)
Atena Editora
This article seeks to explore adaptive reuse as a form of connection between the preservation of architectural and urban heritage and the sustainability of the built environment. Reuse in architecture can make the use of spaces more effective while preserving memory, as new life is given to buildings that have potential for use. Furthermore, bringing new function to an underutilized or disused historic building means avoiding complete demolition and less need for construction. Historic buildings represent much more than simply a physical construction, but also something that brings identity and character to the city and serves as a witness to the history of the place. Adaptive reuse is considered a preservation strategy; however it is only effective if it brings social fruition to the building. In this study, the category of reuse represents a new way of conceiving architecture in the 21st century. In this context, we discuss the reasons that make the reuse of architecture and urban ambience a viable alternative, in many cases, for the sustainable preservation of heritage and for the best use of a potential built environment. The criteria that make adaptive reuse an effective strategy for both environmental sustainability and heritage preservation are also presented and discussed. To be considered sustainable, adaptive reuse must preserve the historical value of the building and, at the same time, holistically bring social, economic and environmental advantages to it.
IntechOpen eBooks
Maya Hassan
nilufer saglar onay
Interior architecture is mainly concerned with adapting existing buildings to new uses and requirements. While determining the extent of intervention, the historic and cultural background of the building plays a very important role. Therefore in adaptive reuse, before starting to develop design proposals, buildings of cultural significance need to be analyzed carefully in order to determine architectural and spatial potentials. This paper aims to evaluate the process and results of a design studio, which was realized during 2014-2015 Fall Semester in the ITU Department of Interior Architecture. The main purpose of the studio experience was to create adaptive reuse proposals for a historic commercial building by focusing on the theme of “functional unity”. In the first phase of the study, course program was organized in three basic steps: analyzing spatial potential, determining compatible use and developing project proposals. At the end of every step there was a jury to evaluate each phase. Every step had its own priorities and criteria for the jury. After evaluations project proposals were classified according to their main foci as well as advantages and disadvantages of different approaches in terms of functional unity. As a result it was observed that in historic buildings there are different ways of maintaining functional unity based on the intention of the intervention. While identifying compatible use or uses for a historic building, functional unity needs to be evaluated as one of the basic design criteria in order to retain its cultural significance. This is mainly because a historic building can fully reveal it’s potential only if it is experienced and evaluated as a whole.
Esra Ozkan Yazgan
In this study, the Museum of Innocence, a personal museum fictionalized in parallel to Orhan Pamuk's novel of the same name, is examined in context of adaptive re-use on the basis of its adaptive reuse fiction directing the transformation of the Brukner Apartment. By reason of the fact that it is built both in architectural and literal fields collaterally, the Museum of Innocence has an unusual transformation story created within the intersection of fact and fiction. On that sense, as promoting an alternative way to reuse a historical house, it sets a unique example to discuss the transformation and evolution of residential environment and its sustainability within the urban context. Through the study, the transformation process of the Brukner Apartment and the dynamics of this transformation are discussed. Focusing on the method of the transformation and the content of the new usage, creation of the adaptive reuse fiction in collaboration of architecture and literature through an interdisciplinary dialog and configuration of the content of the fiction to be based on everyday life practices are highlighted. Analyzing the new spatial situation of the Museum of Innocence, success of the adaptive re-use fiction to ensure the sustainability of urban, cultural and social structure is exposed.
Jack Chongbut
Winsor house has a long standing continuous history for 100 years. It presents a unique setting especially with its architectural styles, its land use components and spatial patterns reflecting the change of their living’s patterns. The characteristics of the house is generally crowed, placed in rows and separated by narrow walkways; some ancient style wooden houses. This report is focused on the cultural significance of Winsor house and proposing a conservational plan of this house and contributing to the general understanding of its value and how to conserve the house for cultural tourism. There is need for a conservational plan to be presented to the community, local government, private and government sectors in order to set a conservational plan. These conservational and developing plans should avoid adverse impacts on the authenticity and physical aspects of cultural heritage. Clearly, there is a need to conserve its cultural heritage attractions. And this affords the opportunity to enhance tourism’s economic contribution to a community and a country. Therefore, these conservational and developing plans can be the tool to create a future in which a stable residential core is enlivened and sustained by a widespread system of retail activities, supported by essential infrastructure and community facilities and made more attractive by well-maintained open spaces and monuments.
Dicle AYDIN
RELATED PAPERS
Plant Production Science
asana matsuura
Quaternaire
carozza laurent
Journal of Environment and Ecology
OBINNA CHUKWU
mario schmidt
ნინიკო თასოშვილი
Endocrinology
Gloria Delgado
Raúl López Fernández
Mansoor Amiji
Daniela Patiño Rodriguez
Alzheimer's & Dementia
Koichi Ishiguro
Journal of Agricultural Research
Dr Mohamed Abdel-Raheem
Dr. Chandana Unnithan
pasquale londrillo
Journal of NeuroVirology
Nicole Barp
Happy Utami Ambarsih
Revista Espanola De Educacion Fisica Y Deportes
Jaime Lopez Prado
Musiken i Uppsala under stormaktstiden. Part 2
Jan Olof Rudén
Indonesian Journal of Statistics and Applications
Nur Iriawan
International Journal of Ophthalmology and Clinical Research
Revista Española de Podología
Julián García Carrasco

RELATED TOPICS
- We're Hiring!
- Help Center
- Find new research papers in:
- Health Sciences
- Earth Sciences
- Cognitive Science
- Mathematics
- Computer Science
- Academia ©2024
- Help & contact
Built Environment and Architecture as a Resource
- Aarhus School of Architecture
- Research Lab 1: Transformation
Publications : Book / Anthology / Thesis / Report › Anthology › Research › peer-review
- architecture
- built environment
- sustainability
Artistic research
Access to document.
- http://arkitekturforskning.net/na/issue/publishing
Research output
- 2 Book chapter
Research output per year
Publications : Chapter in Book/Report/Conference proceeding › Book chapter › Research
Introduction
T1 - Built Environment and Architecture as a Resource
A2 - Chudoba, Minna
A2 - Hynynen, Ari
A2 - Rönn, Magnus
A2 - Toft, Anne Elisabeth
PY - 2020/7/30
Y1 - 2020/7/30
N2 - This anthology is the proceedings publication from the 2018 NAF Symposium “Built Environment and Architecture as a Resource”.Focusing its discussions on a research interest shared by The Nordic Association of Architectural Research and its collaborators from Tampere University of Technology in Finland, the book is dedicated to reflections on how cities, neighbourhoods, buildings, and citizens can become resilient and what role architects and urban planners may play in this process. It gives an account of some of the many future challenges of society, and it discusses the social and cultural construction of concepts and theories that define society’s understanding of resilience in relationship to the design of the built environment.Editors: Minna Chudoba, Ari Hynynen, Magnus Rönn and Anne Elisabeth ToftContributing authors:Ida Andersson, Dalia Milián Bernal, Lars Nicolai Bock, Minna Chudoba, Lionel Devlieger, Julia Donner, Ranja Hautamäki, Hella Hernberg, Ari Hynynen, Guillermo Martín Jiménez, Anna Kholina, Matti Kuittinen, Magnus Rönn, Birgitte Tanderup Eybye, Anne Elisabeth Toft, Inge Vestergaard
AB - This anthology is the proceedings publication from the 2018 NAF Symposium “Built Environment and Architecture as a Resource”.Focusing its discussions on a research interest shared by The Nordic Association of Architectural Research and its collaborators from Tampere University of Technology in Finland, the book is dedicated to reflections on how cities, neighbourhoods, buildings, and citizens can become resilient and what role architects and urban planners may play in this process. It gives an account of some of the many future challenges of society, and it discusses the social and cultural construction of concepts and theories that define society’s understanding of resilience in relationship to the design of the built environment.Editors: Minna Chudoba, Ari Hynynen, Magnus Rönn and Anne Elisabeth ToftContributing authors:Ida Andersson, Dalia Milián Bernal, Lars Nicolai Bock, Minna Chudoba, Lionel Devlieger, Julia Donner, Ranja Hautamäki, Hella Hernberg, Ari Hynynen, Guillermo Martín Jiménez, Anna Kholina, Matti Kuittinen, Magnus Rönn, Birgitte Tanderup Eybye, Anne Elisabeth Toft, Inge Vestergaard
KW - arkitektur
KW - resilience
KW - bæredygtighed
KW - NAF/NAAR
KW - architecture
KW - built environment
KW - sustainability
M3 - Anthology
SN - 978-91-983797-4-7
T3 - NAAR Proceedings Series
BT - Built Environment and Architecture as a Resource
PB - Nordic Academic Press of Architectural Research
CY - Sverige
T2 - NAF/NAAR SYMPOSIUM 2018
Y2 - 31 May 2018 through 1 June 2018
Built Environment Thesis
Description.
This module follows on from the module entitled 'Built Environment Research'. This is the capstone module for the MSc in the Built Environment Regulations. Candidates will be invited to conduct research to a master's degree level in a topic area of their choosing, within the broad field of the Built Environment Regulations.
Candidates will learn through their research journey the skills required to write a mini-thesis (15,000 words) and an academic paper (7,000 words) from the same research design, with a research supervisor appointed to each learner. Candidates will be encouraged to present their research at various stages through circle meetings with lecturing staff and their peers while also participating and sharing their research in workshops scheduled throughout the semester.
Candidates will be encouraged to present their research findings at an external and/or internal conference. Subsequently, conference research papers which reach a sufficiently high academic standard will be invited to contribute to a peer-reviewed GMIT journal appropriate for the Built Environment.
Learning Outcomes
Critically evaluate current problems and new insights at the forefront of the built environment regulations.
Conduct searches of literature and consult and critically use databases and other sources of information to pursue detailed investigations of an unfamiliar problem.
Demonstrate problem-solving and reflective critical thinking in dialogue with the literature and apply ethically appropriate primary and secondary research data collection methodologies to a chosen topic.
Design and conduct investigations, experiments and simulations, to analyse and interpret data, and draw conclusions using the principles of validity and reliability in the data analysis.
Contribute to the development of scientific/technological knowledge in one or more areas of the built environment regulations.
Write a mini-thesis and a technical paper, and synthesise one’s own work and that of others in the academic field.
Disseminate and debate built environment regulation research among peers.

Built Environment: Theses
- Books & eBooks
- Journals & Databases
- eTutorials & Guides
- Citing & Referencing This link opens in a new window
Theses in the Library
There is a selection of Built Environment related theses in the library. They are shelved in subject and date order in cabinets in the library. Folders listing titles and authors are held at the Library Desk. Browse through these to find topics of interest to you. Alternatively, follow the guidelines in the video below to search for listings of titles and subject areas on the library catalogue.
Please note: Theses are for library use only.
DART-Europe
DART-Europe was founded in 2005 as a partnership of national and university libraries and consortia to improve global access to European research theses. The DART-Europe E-theses Portal is managed by UCL Library Services .
The DART-Europe partners help to provide researchers with a single European Portal for the discovery of Electronic Theses and Dissertations. Free access to 825,310 open access research theses from 619 Universities in 28 European countries.
EthOS - British Library

EThOS is the UK’s national thesis service which aims to maximise the visibility and availability of the UK’s doctoral research theses. It demonstrates the quality of UK research, and supports the UK Government’s open access principle that publications resulting from publicly-funded research should be made freely available for all researchers, providing opportunities for further research.
Research @THEA
Research@THEA
Research@THEA is a free electronic resource where you can search the collections of the Technological Universities (TU) research output in a single search. Alternatively you can opt to search the research from a single TU using the individual site details.
Open Access Theses & Dissertations

OATD.org aims to be the best possible resource for finding open access graduate theses and dissertations published around the world
Digital Commons Network

The Digital Commons Network brings together free, full-text scholarly articles from hundreds of universities and colleges worldwide. Curated by university librarians and their supporting institutions, the Network includes a growing collection of peer-reviewed journal articles, book chapters, dissertations, working papers, conference proceedings, and other original scholarly work.
BASE , one of the world's most voluminous search engines especially for academic web resources, is operated by Bielefeld University Library . BASE provides more than 240 million documents, including dissertations and theses from more than 8,000 content providers. You can access the full texts of about 60% of the indexed documents for free (Open Access).
With PQDT Open, you can read the full text of open access dissertations and theses free of charge (primarily North American in content).
- << Previous: eTutorials & Guides
- Next: Citing & Referencing >>
- Last Updated: Oct 13, 2023 10:08 AM
- URL: https://lit.libguides.com/builtenvironment
The Library, Technological University of the Shannon: Midwest

Meeting postponed
More information
Built environment
Stichting schutsluis alblasserdam.
In the engineering and social sciences, the term ‘built environment’, or built world, refers to the human-made environment that provides the setting for human activity, ranging in scale from buildings to cities and beyond. It has been defined as "the human-made space in which people live, work and recreate on a day-to-day basis."
The built environment encompasses places and spaces created or modified by people to serve their needs of accommodation, organisation and representation.
The sciences of the built environment cover architecture, urbanism, building technology, civil engineering, landscaping and the management of built stock mutations and operations.
In recent years public health research has expanded the definition of "built environment" to include healthy food access, community gardens, mental health, physical health, "walkability", and "bikeability".
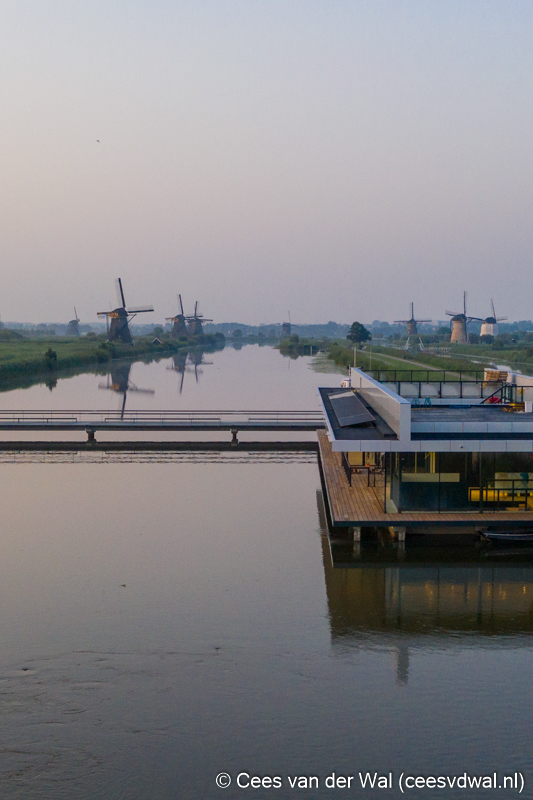
Initiator Arie den Boer | P.O.Box 235, 2950 AE Alblasserdam | E: [email protected]
A guide to decarbonizing the built environment
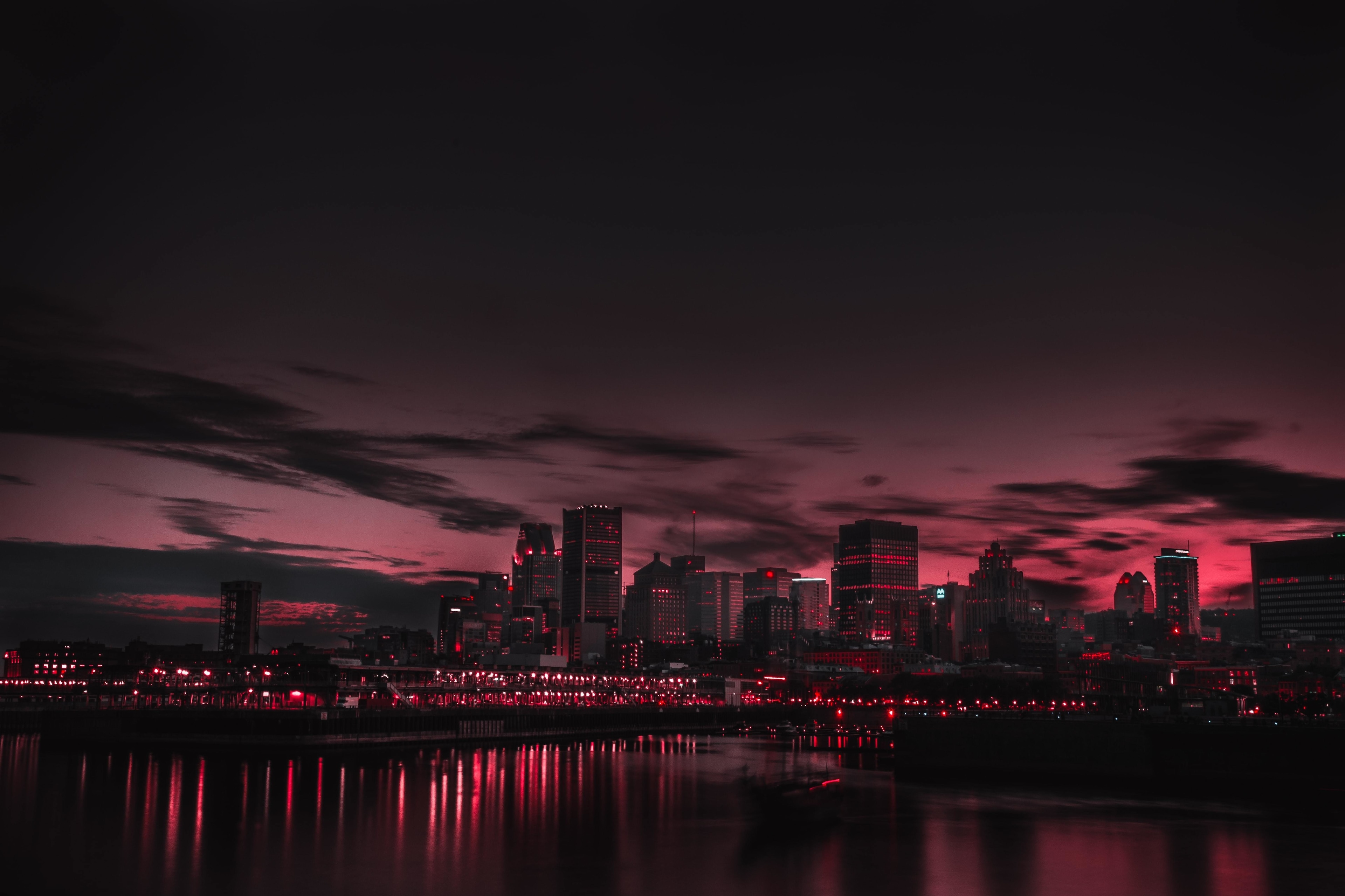
The built environment produces 40% of global emissions - which makes it a huge opportunity to meet our climate goals. Image: Marc-Olivier Jodoin / Unsplash
.chakra .wef-1c7l3mo{-webkit-transition:all 0.15s ease-out;transition:all 0.15s ease-out;cursor:pointer;-webkit-text-decoration:none;text-decoration:none;outline:none;color:inherit;}.chakra .wef-1c7l3mo:hover,.chakra .wef-1c7l3mo[data-hover]{-webkit-text-decoration:underline;text-decoration:underline;}.chakra .wef-1c7l3mo:focus,.chakra .wef-1c7l3mo[data-focus]{box-shadow:0 0 0 3px rgba(168,203,251,0.5);} Mark Edward Rose
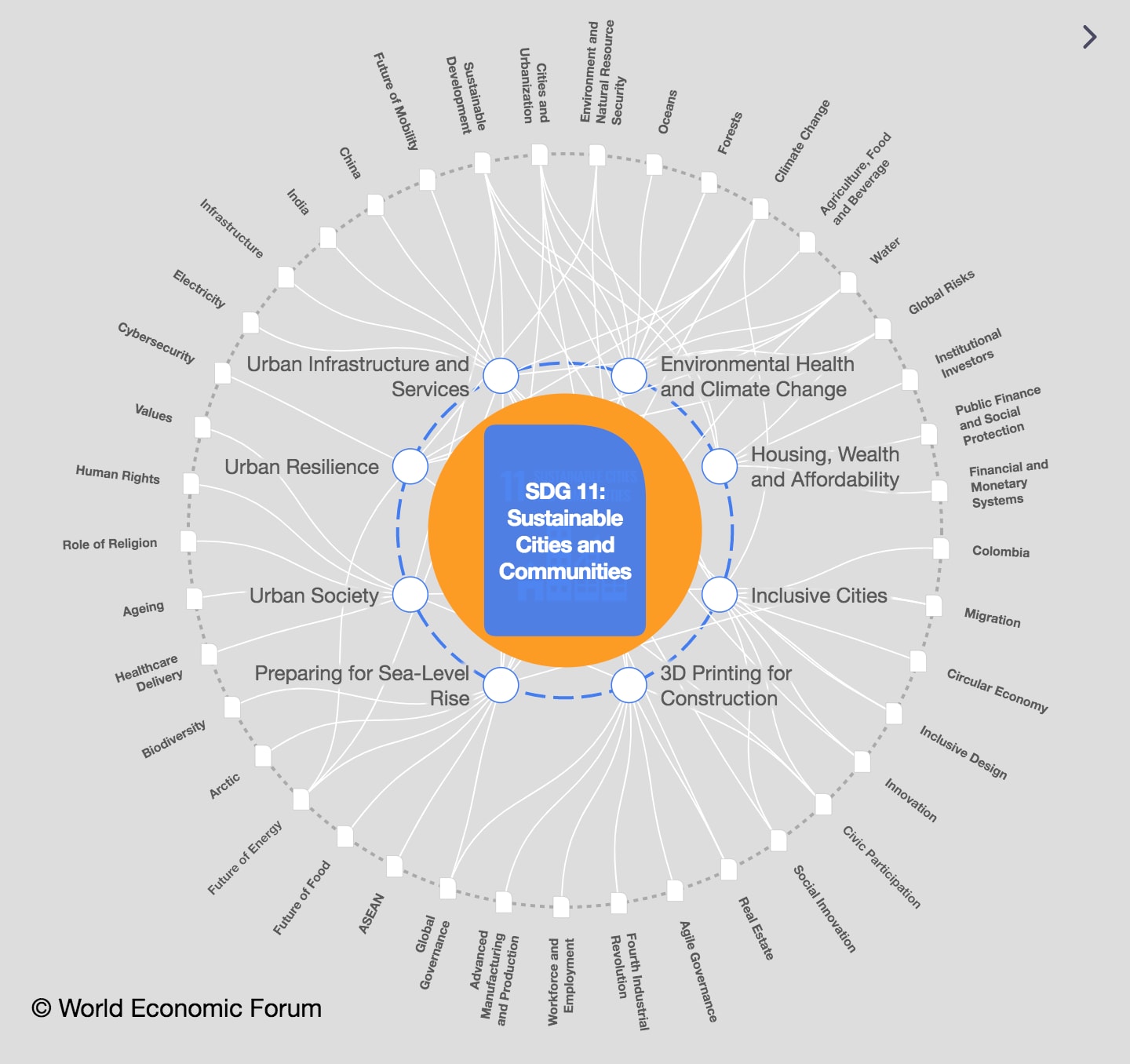
.chakra .wef-9dduvl{margin-top:16px;margin-bottom:16px;line-height:1.388;font-size:1.25rem;}@media screen and (min-width:56.5rem){.chakra .wef-9dduvl{font-size:1.125rem;}} Explore and monitor how .chakra .wef-15eoq1r{margin-top:16px;margin-bottom:16px;line-height:1.388;font-size:1.25rem;color:#F7DB5E;}@media screen and (min-width:56.5rem){.chakra .wef-15eoq1r{font-size:1.125rem;}} SDG 11: Sustainable Cities and Communities is affecting economies, industries and global issues
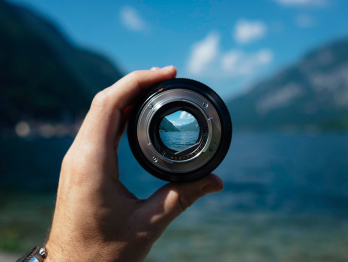
.chakra .wef-1nk5u5d{margin-top:16px;margin-bottom:16px;line-height:1.388;color:#2846F8;font-size:1.25rem;}@media screen and (min-width:56.5rem){.chakra .wef-1nk5u5d{font-size:1.125rem;}} Get involved with our crowdsourced digital platform to deliver impact at scale
Stay up to date:, sdg 11: sustainable cities and communities.
Listen to the article
- Cutting emissions from the built environment is urgently needed if we are to meet our climate goals.
- Buildings account for around 40% of total global energy use and emissions.
- From transportation to new-build and retrofit – here are 10 priority areas for public and private sector decision-makers to focus on.
Having spent my entire career working in real estate, it is simultaneously humbling and daunting to acknowledge that buildings account for around 40% of total global energy use and emissions.
I suspect that decarbonization will profoundly impact almost every aspect of my personal and professional life – but with creativity, innovation and the use of technology I believe we can deliver the transformation required. Below, I set out a priority list of issues in our built environment where collaboration between companies and governments is urgently needed to accelerate progress towards our common goals.
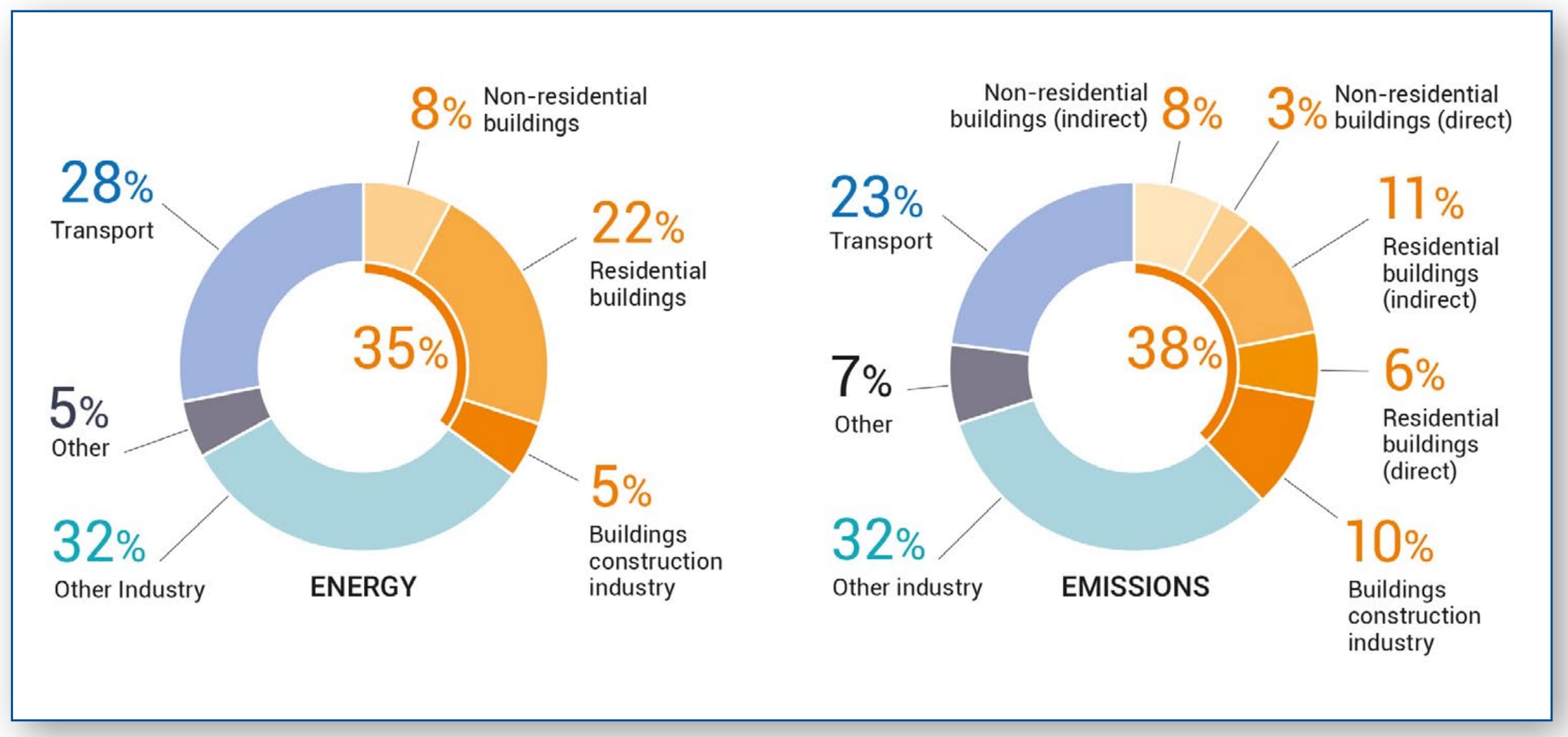
Urban urgency
Cities account for 75% of global emissions and are often most exposed to the consequences of climate change. Of the world’s 17 largest cities, 14 are coastal, making them particularly vulnerable to climate-related extreme weather events. 'Necessity is the mother of invention' takes on new meaning as cities are thrust into the frontline of the battle to reduce climate impacts and mitigate their consequences. Regulations around building construction and operation, and planning policies that promote sustainable transport, are among the key areas in which forward-thinking cities like New York are leading the charge.
Transportation
Pandemic-induced lockdowns showed us the benefits of reduced vehicle movements. Emissions fell 88% across Europe during quarantine. Restrictions – and eventual bans – on combustion engine vehicles are being introduced in cities to accelerate the adoption of electric vehicles, often starting with public transport infrastructure. A Clean Air Zone introduced in Birmingham, UK, has materially altered travel patterns (see figure below). Cycle lanes introduced in Paris as a response to COVID are being retained permanently. Carbon accounting must increasingly sit at the heart of policymaking around urban transportation.
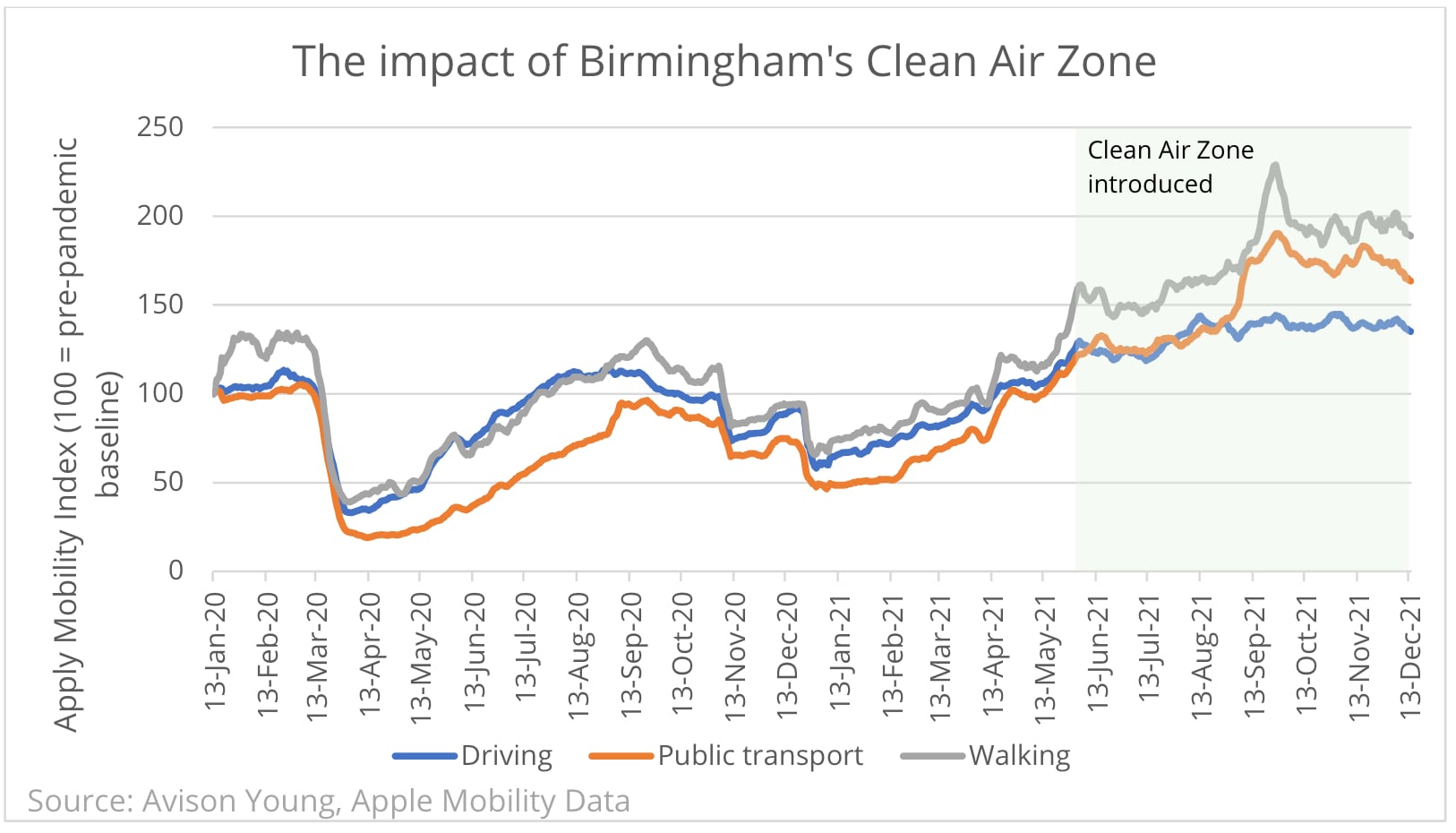
The war for talent
The pandemic has also impacted the labour market, as characterised by plentiful job openings and record levels of resignations. Companies’ environmental, social and governance (ESG) credentials are set to play an ever-greater role in attracting and retaining top talent, who increasingly believe their employer should have a social and environmental conscience. The buildings they occupy, the locations they select, and the ways in which they allow their staff to work will have a profound impact on companies’ carbon footprints. In turn, this will influence whether they are seen as an ' employer of choice '.
Building construction
Over the next 40 years, the equivalent of a city the size of Paris is expected to be built every week. Given that concrete production alone makes up 8% of total global emissions, the way we design and construct new buildings needs to change radically to achieve our climate goals. Improvements in design, innovative materials, new construction techniques and increased use of technology are all helping to minimize the ' whole-life carbon ' impact of new developments. The use of digital twins to regulate energy usage in buildings is showing tremendous promise. Given that typical buildings exceed their designed energy usage by 3.8 times , the power of digital twins to diagnose and rectify such inefficiency makes a material contribution towards reducing emissions.
Retrofit vs. new construction
Perhaps the biggest challenge is the vast stock of existing buildings that need to continue in use – but which must be operated more efficiently. Retrofitting occupied buildings presents practical challenges of upgrading facilities without disrupting business operations, as well as the more nebulous issue of cost allocation and financial viability. Early adopters in both public and private sectors are providing templates for others to follow. However, in the UK and the EU, government regulation around building standards is already accelerating obsolescence in order to alter the balance of the cost-benefit equation. Other jurisdictions are likely to follow their lead. Financing and undertaking the retrofits needed is a huge challenge but also offers huge commercial opportunities for those with the right skills and vision.
Green finance
Legislation may be slow to impact the real estate sector directly, but successive waves of regulatory and market forces are already impacting the banking and financial industries upon which the property market is constructed. Whether in the form of construction loans or mortgage finance, banks’ exposure to climate risk is increasingly being measured, monitored and costed. Funds explicitly targeting 'green' investments are becoming commonplace; those avoiding 'brown' ones even more so. Sustainable finance is already growing exponentially and will soon impact the cost and availability of debt and equity for every real estate project.
Money talks (and walks)
Real estate is inherently geographically fixed, yet every location on the planet is exposed to its own unique combination of regulatory and physical climate risks. Both occupiers and investors are reassessing their locational exposure in light of these new risks, which are only now being recognized and appropriately priced. Rents and capital values are already adjusting at the building level , but climate impacts on value at urban and regional levels are – so far – less easy to discern. Property insurance costs have soared in response to recent extreme weather events – this, surely, is a leading indicator of how the real estate value landscape is being reshaped by climate change and our response to it.
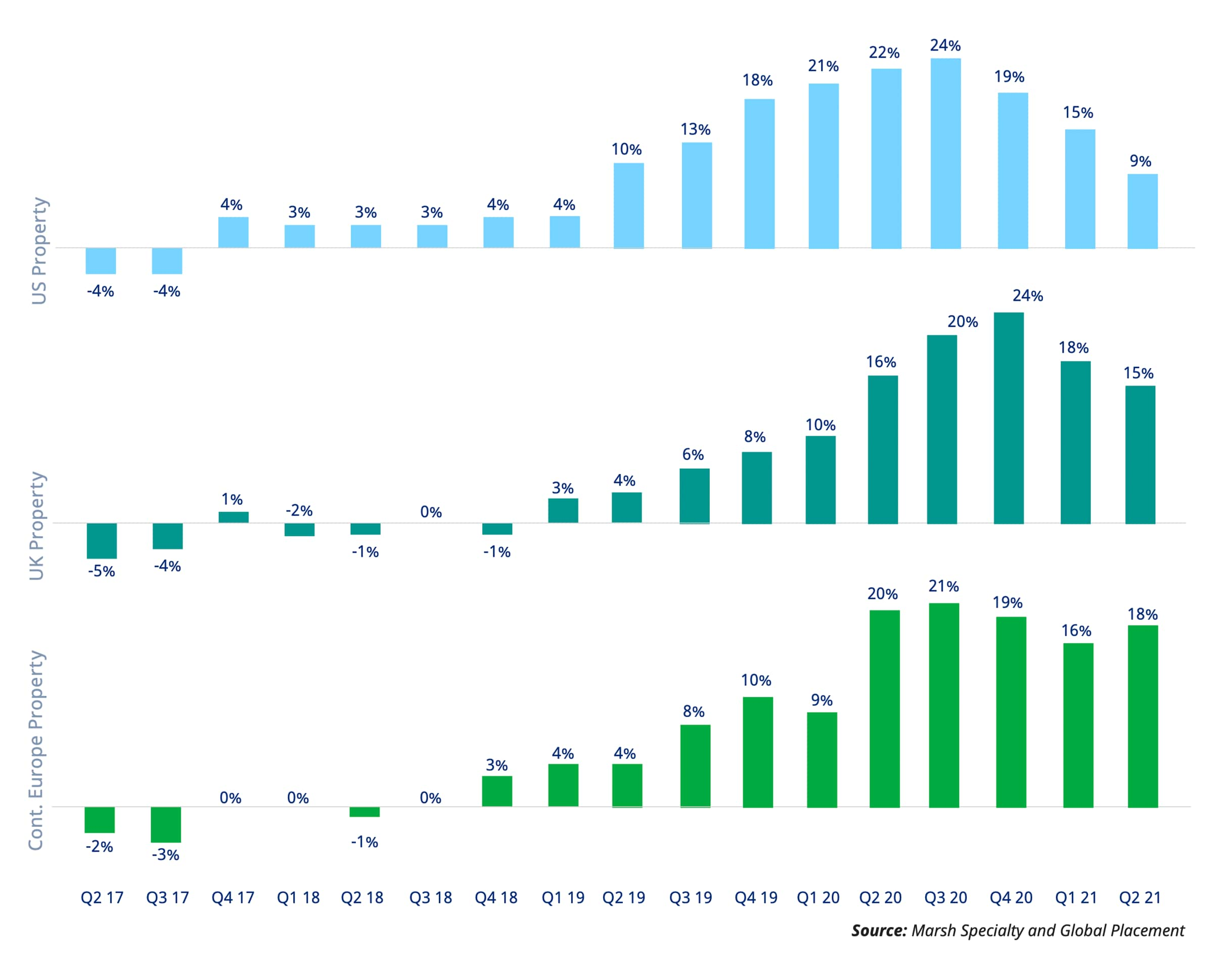
The big picture
The impacts of climate change fall disproportionately on parts of the world and sections of society that are least able to protect themselves or recover from climate events. From loss of home or livelihood to loss of life, millions of people face a rising tide of social and economic impact as a result of climate change. As the World Bank so neatly put it: “Climate change is more than an environmental crisis – it is a social crisis.”
I believe that we are all part of a human ecosystem, at the heart of which lie the towns and cities that form the backdrop to our lives. The real estate industry was responsible for creating the buildings in which we live, work and play; but we cannot solve the current crisis on our own. Now we must urgently reach out to our partners elsewhere in the public and private sector to take on the challenge of decarbonizing the built environment for the benefit of society as a whole.
Don't miss any update on this topic
Create a free account and access your personalized content collection with our latest publications and analyses.
License and Republishing
World Economic Forum articles may be republished in accordance with the Creative Commons Attribution-NonCommercial-NoDerivatives 4.0 International Public License, and in accordance with our Terms of Use.
The views expressed in this article are those of the author alone and not the World Economic Forum.
Related topics:
The agenda .chakra .wef-n7bacu{margin-top:16px;margin-bottom:16px;line-height:1.388;font-weight:400;} weekly.
A weekly update of the most important issues driving the global agenda
.chakra .wef-1dtnjt5{display:-webkit-box;display:-webkit-flex;display:-ms-flexbox;display:flex;-webkit-align-items:center;-webkit-box-align:center;-ms-flex-align:center;align-items:center;-webkit-flex-wrap:wrap;-ms-flex-wrap:wrap;flex-wrap:wrap;} More on Forum Institutional .chakra .wef-nr1rr4{display:-webkit-inline-box;display:-webkit-inline-flex;display:-ms-inline-flexbox;display:inline-flex;white-space:normal;vertical-align:middle;text-transform:uppercase;font-size:0.75rem;border-radius:0.25rem;font-weight:700;-webkit-align-items:center;-webkit-box-align:center;-ms-flex-align:center;align-items:center;line-height:1.2;-webkit-letter-spacing:1.25px;-moz-letter-spacing:1.25px;-ms-letter-spacing:1.25px;letter-spacing:1.25px;background:none;padding:0px;color:#B3B3B3;-webkit-box-decoration-break:clone;box-decoration-break:clone;-webkit-box-decoration-break:clone;}@media screen and (min-width:37.5rem){.chakra .wef-nr1rr4{font-size:0.875rem;}}@media screen and (min-width:56.5rem){.chakra .wef-nr1rr4{font-size:1rem;}} See all
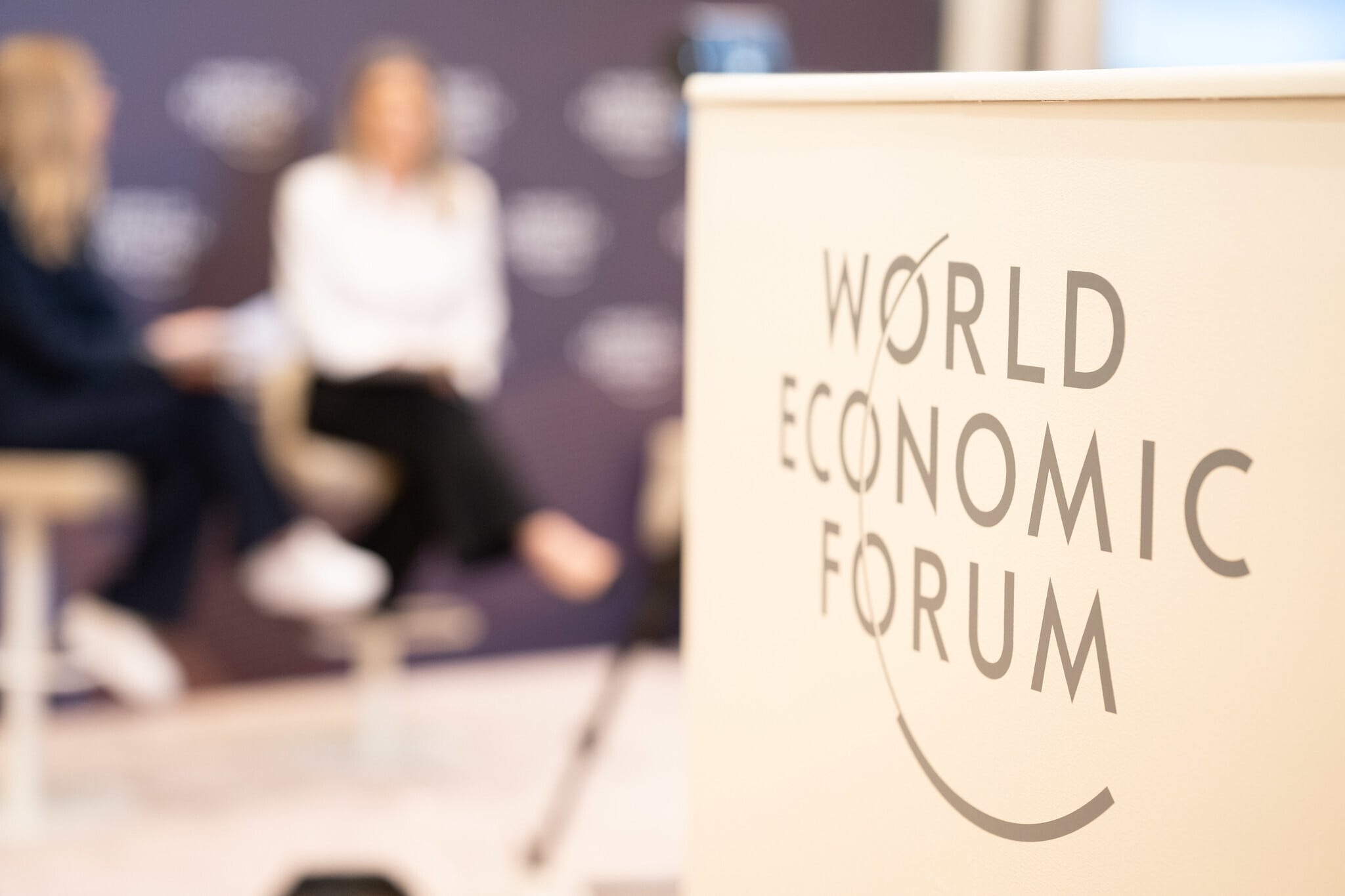
Reflections from MENA at the #SpecialMeeting24
Maroun Kairouz
May 3, 2024
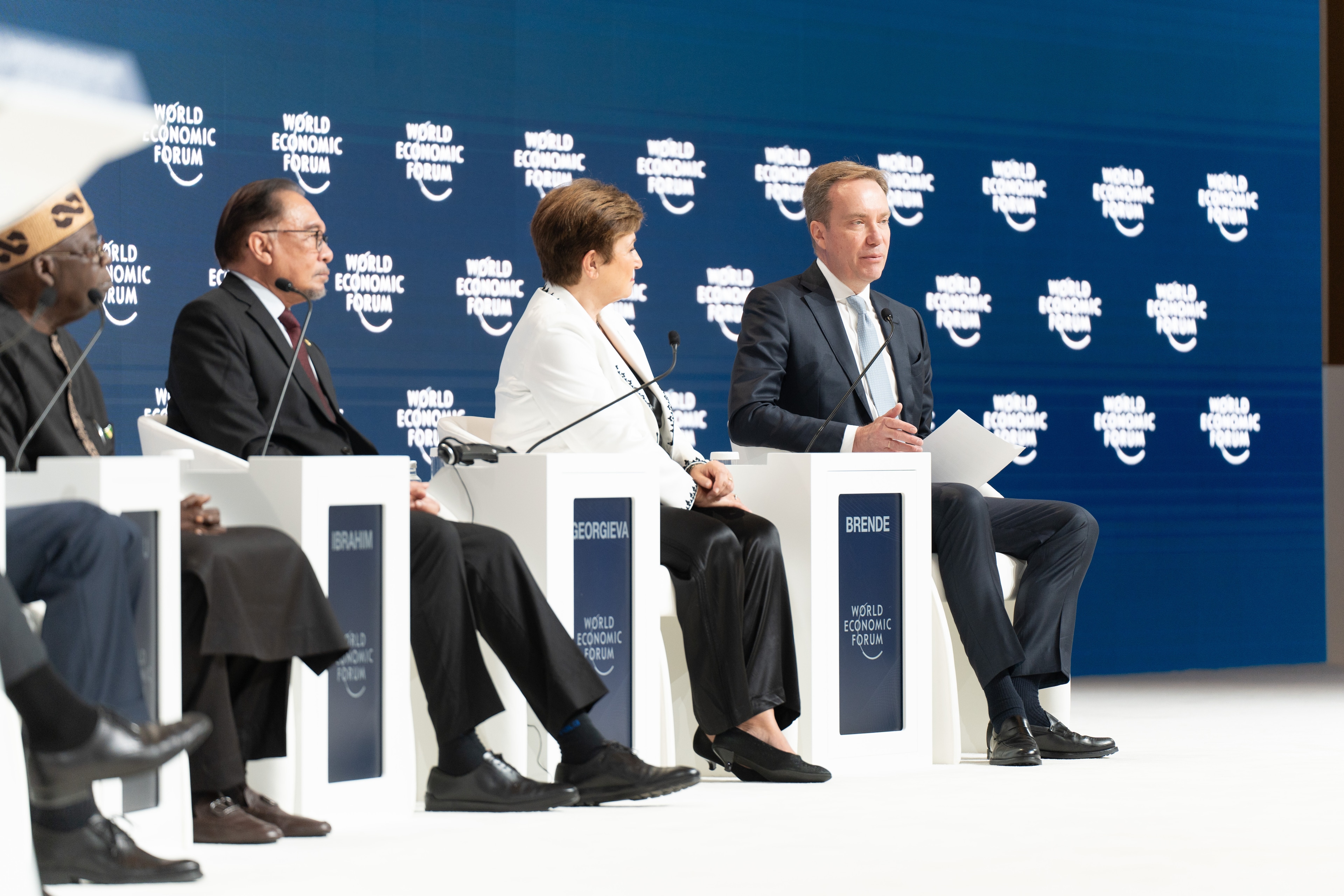
Day 2 #SpecialMeeting24: Key insights and what to know
Gayle Markovitz
April 28, 2024
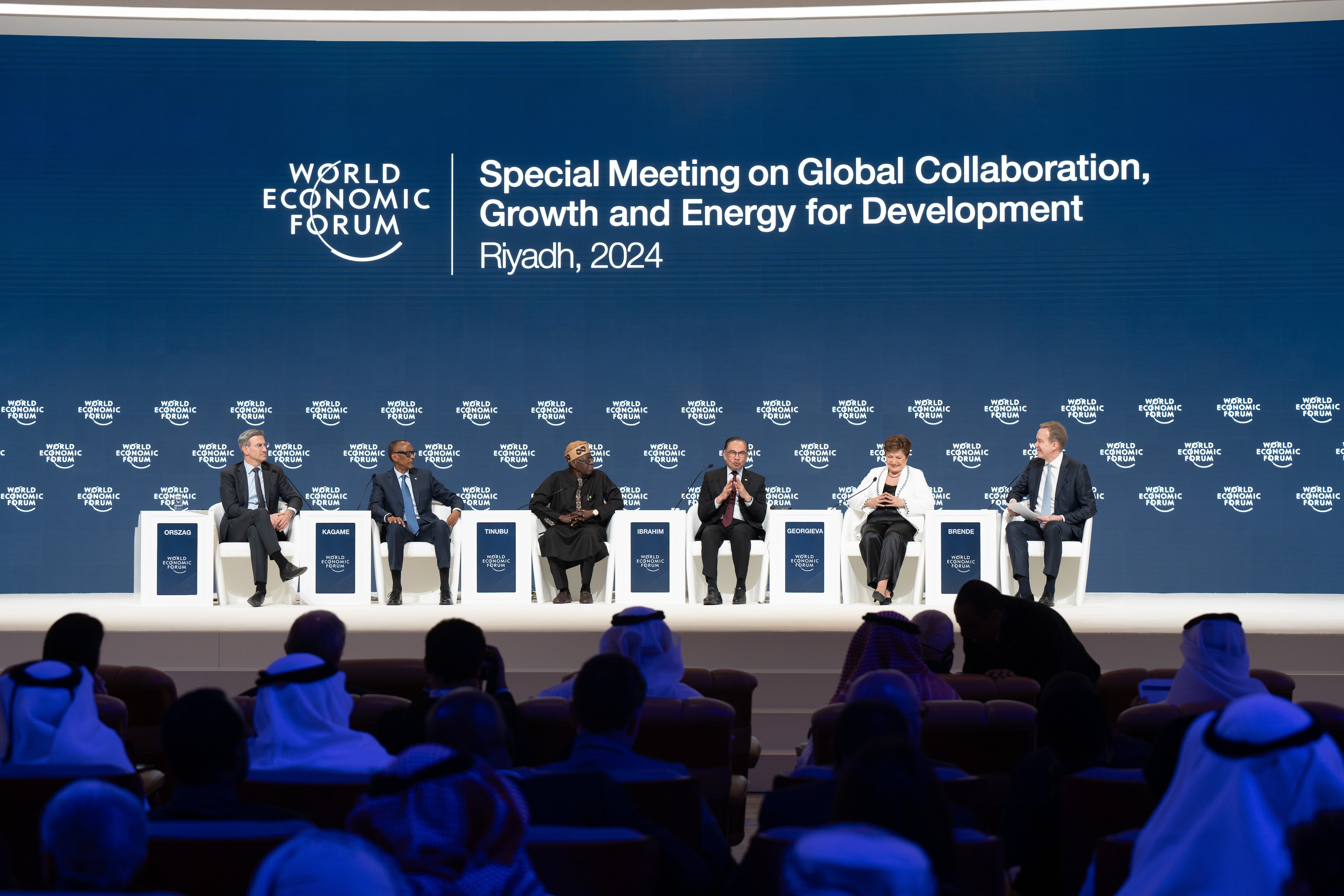
Day 1 #SpecialMeeting24: Key insights and what just happened
April 27, 2024
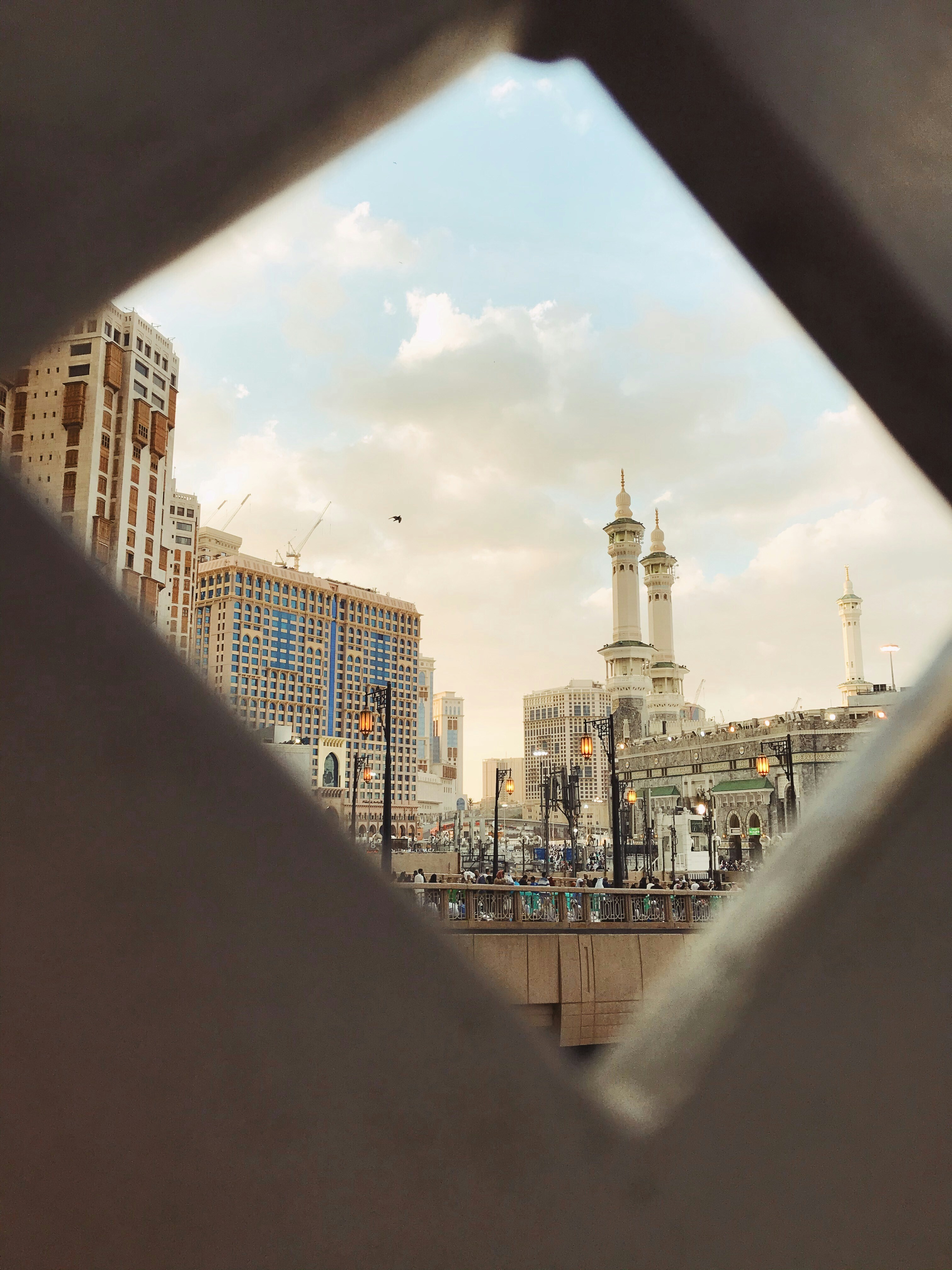
#SpecialMeeting24: What to know about the programme and who's coming
Mirek Dušek and Maroun Kairouz
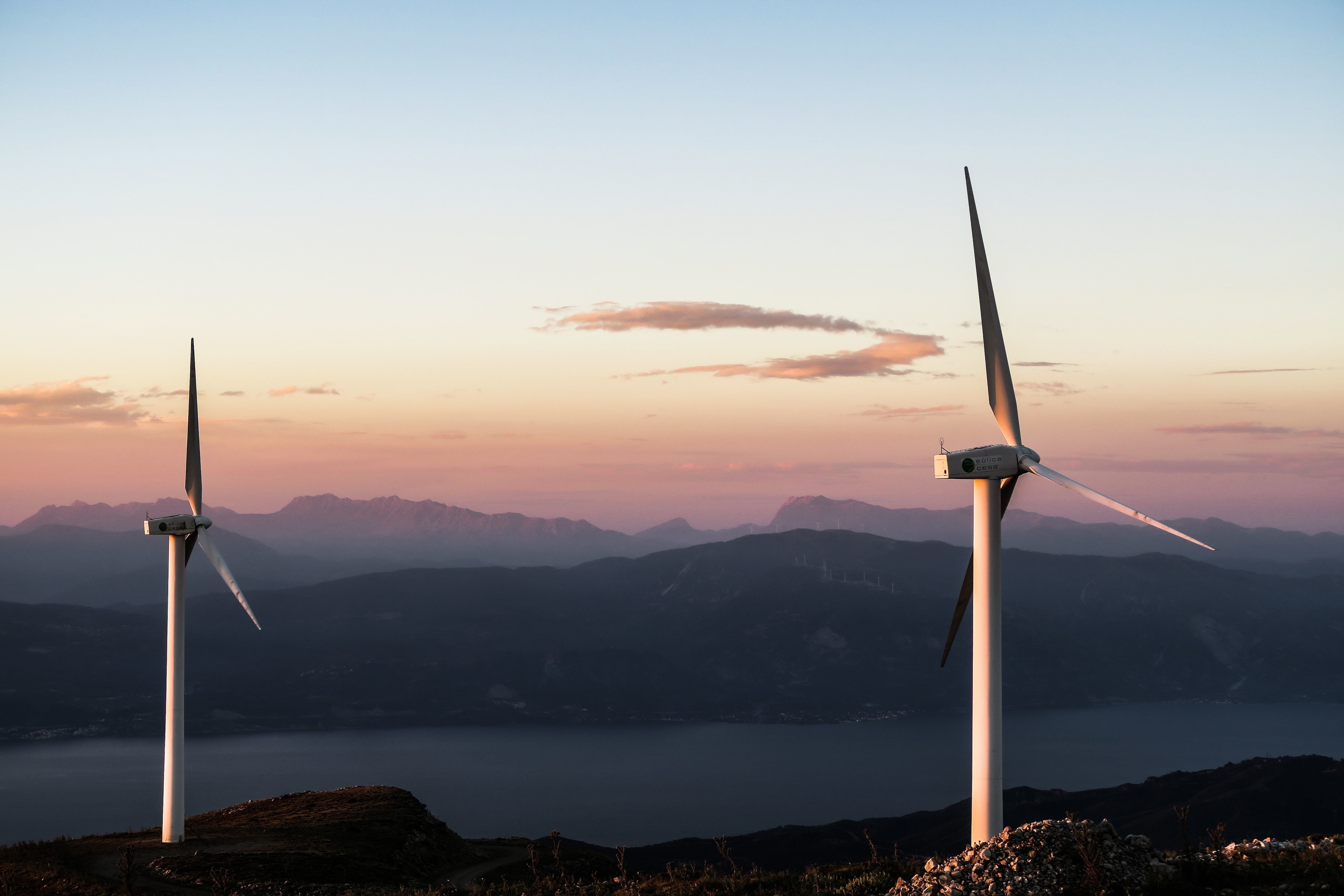
Climate finance: What are debt-for-nature swaps and how can they help countries?
Kate Whiting
April 26, 2024
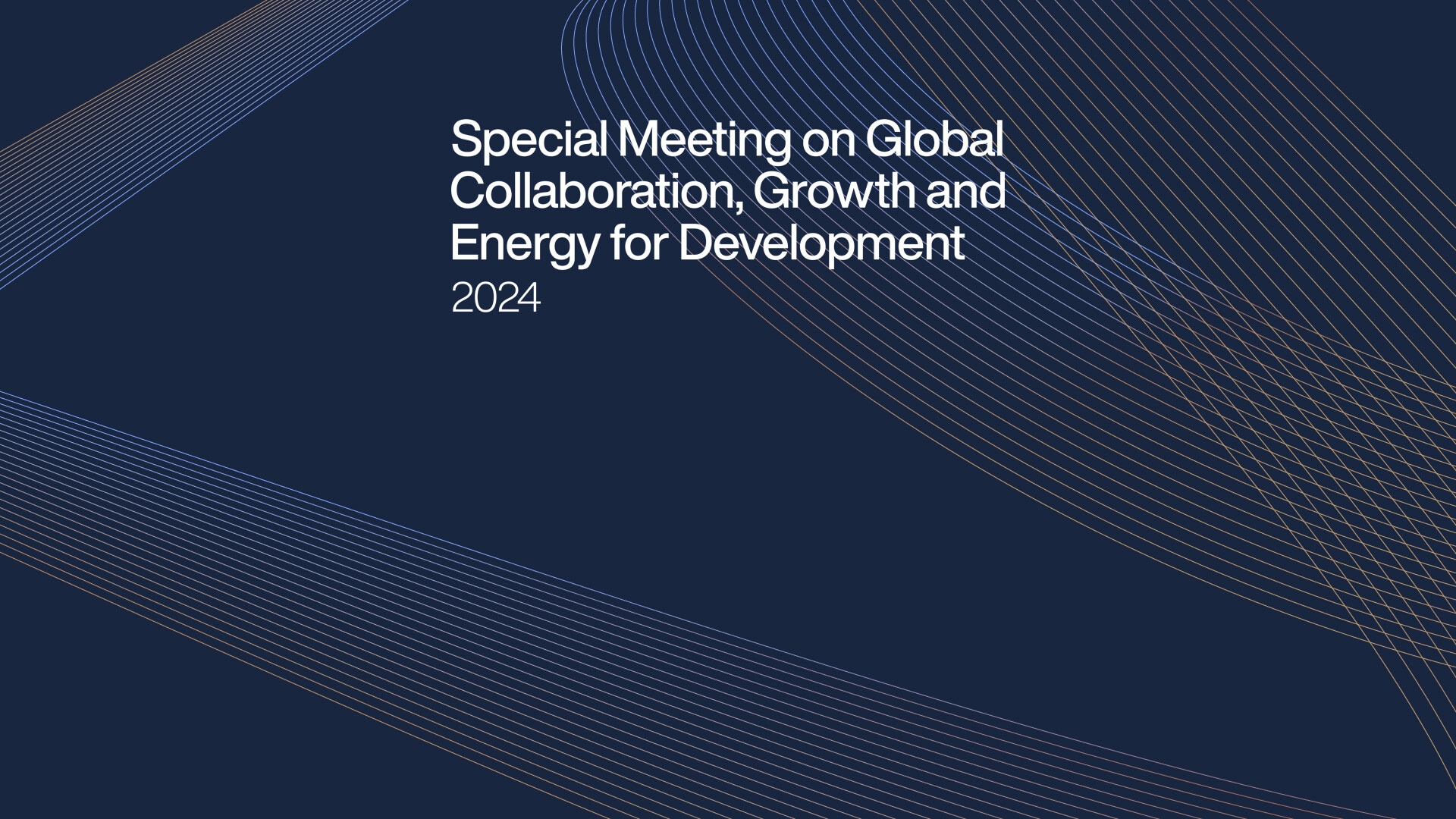
What to expect at the Special Meeting on Global Collaboration, Growth and Energy for Development
Spencer Feingold and Gayle Markovitz
April 19, 2024
- Search News and Events
- News overview
- Media relations
Wind’s impact on asbestos depressurization
Anjali Radhakrishnan Jayakumari defended her PhD thesis at the Department of Built Environment on May 15th.
![[Translate to English:] [Translate to English:]](https://assets.w3.tue.nl/w/fileadmin/_processed_/0/1/csm_Radhakrishnan%20banner%20image_82dc703b64.jpg)
Asbestos abatement is a process fraught with risks, as it can release harmful fibers into the air, posing a significant threat to public health and workers' safety. Governments worldwide have set guidelines for this process, one of which is to maintain the pressure inside the asbestos-containing zones lower than the outdoor environment. However, these guidelines overlook a crucial factor - the impact of wind on the pressure difference between the indoor and outdoor environment. For her PhD research, Anjali Radhakrishnan Jayakumari delved into this unexplored territory, investigating the effects of wind on internal depressurization during asbestos abatement.
Research indicates that wind can create high suction pressure on building facades and roofs, potentially leading to the release of asbestos fibers through leakages in the envelope of the asbestos-containing zone. Therefore, understanding the impact of wind on asbestos abatement is crucial. Anjali Radhakrishnan Jayakumari’s PhD thesis sought to investigate how atmospheric winds affect the depressurization established using a mechanical ventilation system during asbestos removal.
Wind-tunnel tests
Radhakrishnan Jayakumari employed wind-tunnel tests and numerical simulations to study a building with asbestos containment and a mechanical ventilation system. The goal was to investigate the adequacy of the standard depressurization set by government authorities and implemented by industries.
Wind-tunnel tests were conducted on a scaled building and ventilation system. By measuring internal and external pressures at various wind speeds and directions, Radhakrishnan Jayakumari found that atmospheric winds significantly affect depressurization.
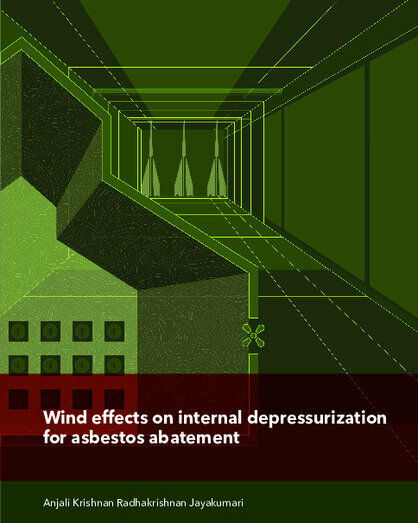
Network model validation
The analyzed depressurization value of -20 Pa, typically used by industries, becomes inadequate, leading to a breach in containment even at lower wind speeds for the building considered. The higher the wind speed, the higher the possibility of a containment breach. The breach probability also depends on wind direction relative to the building’s geometry.
Radhakrishnan Jayakumari 's study validates a ventilation network model named SYLVIA, which can accurately predict internal pressure and containment breach probability. Additionally, the study uses computational fluid dynamics (CFD) simulations to provide input for SYLVIA.
Results show good agreement between CFD and wind-tunnel data, suggesting that CFD simulations can reliably predict external wind pressures for use as input in SYLVIA.
Overall, Radhakrishnan Jayakumari 's research underscores the importance of considering wind effects in asbestos removal processes. By understanding how wind influences depressurization, we can better protect public health and workers' safety during asbestos abatement.
Title of PhD thesis: Wind effects on internal depressurization for asbestos abatement . Supervisors: Twan van Hooff, Stefanie Gillmeier, Romain Guichard.
Media contact

IMAGES
VIDEO
COMMENTS
Metadata. This thesis explores opportunities for extending the life of a building and its components through Design for Disassembly (DfD). Our current way of constructing the built environment is "fixed" and difficult to change. This means that most buildings, once a critical function or building system fails, will be demolished.
Recent intergovernmental panel on climate change reports have once again emphasised the effective measures to reduce greenhouse gas emissions and the importance of the built environment. Historically, passive and active solutions are known for their potential to make the built environment more environmentally friendly. Recently, a significant number of studies covered the effectiveness of such ...
This dissertation focuses on new emergent forms of intelligent architecture in which networked technologies are challenging the way architects reconstruct the built environment. It also explores ...
A Thesis submitted to the Faculty of the Graduate School of Arts and Sciences of Georgetown University in partial fulfillment of the requirements for the degree of Master of Public Policy By Baesung Kim, B.A. ... Built environment can also be important from a policy perspective. That's because policy
Birds and the Built Environment: The Impacts of Architecture, Structures, and Green Spaces on Avian Populations in the United States . Judy Bowes . A thesis . ... architecture, this thesis seeks to provide designers and architects with knowledge about how birds interact with the built environment, a critical assessment of design ...
Developments in the Built Environment (DIBE) is a new peer-reviewed gold open access (OA) journal whereby upon acceptance all articles are permanently and freely available. DIBE publishes original papers and short communications resulting from research in civil engineering and the built …. View full aims & scope. $2840. Article publishing charge.
environment as a whole. Also, since we spend as much as 90% of our time in buildings, we are significantly affected by our interior living and working environments. 2 It will be the goal of this thesis to address the issues of sustainable development in our built environment. This thesis will attempt to answer questions on whether the new
This thesis explores the fundamental aspects of interior architecture regarding the existing built environment. It focuses on the necessity of restoration, preservation and adaptive reuse as possible solutions when approaching a new project concerning an existing building.
The built environment and its users are a direct source of knowledge for design. ... a revised version of the author's 2007 doctoral thesis, is an important contribution to the study of Roman ...
CLIMATE CHANGE AND THE BUILT ENVIRONMENT: AN EVALUATION OF SUSTAINABLE REFURBISHMENT OPTIONS FOR HIGHER EDUCATION BUILDINGS IN THE UK By YAZAN ABU AISHEH A thesis submitted to the University of Birmingham for the degree of DOCTOR OF PHILOSOPHY School of Civil Engineering College of Engineering and Physical Sciences
This master's thesis explores the role of multi-sensory user experience in the built environment. Traditionally, the practice of design has focused heavily on visual stimuli, although these ...
The built environment provides a habitat for the most sophisticated mammal in our universe, the human being. Developments in science and technology are forcing us to reconsider the priority of human needs in current theories of architecture and the built environment. Newly developed theories and methodologies in neuroscience have allowed us to ...
This research article presents a bibliometric analysis, followed by a systematic literature review and, finally, a detailed analysis of the development of theory in the context of CE in the built-environment. 2.1. Bibliometric analysis. Bibliometric analysis is a method to analyze, estimate, and visualize the development of scientific fields.
This thesis focuses both on understanding the impact that the urban built environment has on holistic well-being across a variety of factors as well as understanding how architecture and design can support well-being in changing urban environments. By clearly defining well-being, assessing current standards for well-being, analyzing a variety ...
This anthology is the proceedings publication from the 2018 NAF Symposium "Built Environment and Architecture as a Resource". Focusing its discussions on a research interest shared by The Nordic Association of Architectural Research and its collaborators from Tampere University of Technology in Finland, the book is dedicated to reflections on how cities, neighbourhoods, buildings, and ...
This is the capstone module for the MSc in the Built Environment Regulations. Candidates will be invited to conduct research to a master's degree level in a topic area of their choosing, within the broad field of the Built Environment Regulations. Candidates will learn through their research journey the skills required to write a mini-thesis ...
Report | Pathways to Decarbonizing the Built Environment Towards a Circular Building Industry in Berlin - Emerging Concepts and from the Circular Economy Table of Contents List of figures 5 List of tables 6 Introduction 8 I. Research Design 11 1. Aims 11 2. Methods 12 II. The Role of the Built Environment 14 1. The limits of Resource ...
There is a selection of Built Environment related theses in the library. They are shelved in subject and date order in cabinets in the library. ... EThOS is the UK's national thesis service which aims to maximise the visibility and availability of the UK's doctoral research theses. It demonstrates the quality of UK research, and supports ...
ScholarWorks@UMass Amherst
In the engineering and social sciences, the term 'built environment', or built world, refers to the human-made environment that provides the setting for human activity, ranging in scale from buildings to cities and beyond. It has been defined as "the human-made space in which people live, work and recreate on a day-to-day basis." The built ...
Green roofs cool cities, clean the air, provide habitat, and manage stormwater. They are compelling tools to teach interconnected human-ecological systems. This study included the design, pilot, and evaluation of a fourth-grade science unit entitled "Raising the Green Roof", exploring these connections. Five classrooms in two Midwestern U.S. public elementary schools participated, and 4th ...
Request PDF | On May 14, 2019, Shamil G. Naoum published Dissertation Research and Writing for Built Environment Students | Find, read and cite all the research you need on ResearchGate
Cutting emissions from the built environment is urgently needed if we are to meet our climate goals. Buildings account for around 40% of total global energy use and emissions. From transportation to new-build and retrofit - here are 10 priority areas for public and private sector decision-makers to focus on.
Wind's impact on asbestos depressurization. May 16, 2024. Anjali Radhakrishnan Jayakumari defended her PhD thesis at the Department of Built Environment on May 15th. Asbestos abatement is a process fraught with risks, as it can release harmful fibers into the air, posing a significant threat to public health and workers' safety.