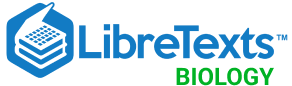
- school Campus Bookshelves
- menu_book Bookshelves
- perm_media Learning Objects
- login Login
- how_to_reg Request Instructor Account
- hub Instructor Commons

Margin Size
- Download Page (PDF)
- Download Full Book (PDF)
- Periodic Table
- Physics Constants
- Scientific Calculator
- Reference & Cite
- Tools expand_more
- Readability
selected template will load here
This action is not available.
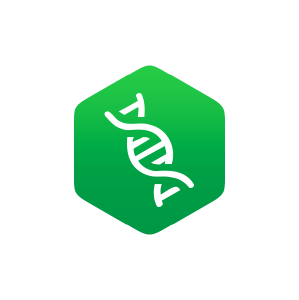
8: Introduction to Viruses
- Last updated
- Save as PDF
- Page ID 10660
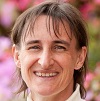
- Linda Bruslind
- Oregon State University via Open Oregon State
\( \newcommand{\vecs}[1]{\overset { \scriptstyle \rightharpoonup} {\mathbf{#1}} } \)
\( \newcommand{\vecd}[1]{\overset{-\!-\!\rightharpoonup}{\vphantom{a}\smash {#1}}} \)
\( \newcommand{\id}{\mathrm{id}}\) \( \newcommand{\Span}{\mathrm{span}}\)
( \newcommand{\kernel}{\mathrm{null}\,}\) \( \newcommand{\range}{\mathrm{range}\,}\)
\( \newcommand{\RealPart}{\mathrm{Re}}\) \( \newcommand{\ImaginaryPart}{\mathrm{Im}}\)
\( \newcommand{\Argument}{\mathrm{Arg}}\) \( \newcommand{\norm}[1]{\| #1 \|}\)
\( \newcommand{\inner}[2]{\langle #1, #2 \rangle}\)
\( \newcommand{\Span}{\mathrm{span}}\)
\( \newcommand{\id}{\mathrm{id}}\)
\( \newcommand{\kernel}{\mathrm{null}\,}\)
\( \newcommand{\range}{\mathrm{range}\,}\)
\( \newcommand{\RealPart}{\mathrm{Re}}\)
\( \newcommand{\ImaginaryPart}{\mathrm{Im}}\)
\( \newcommand{\Argument}{\mathrm{Arg}}\)
\( \newcommand{\norm}[1]{\| #1 \|}\)
\( \newcommand{\Span}{\mathrm{span}}\) \( \newcommand{\AA}{\unicode[.8,0]{x212B}}\)
\( \newcommand{\vectorA}[1]{\vec{#1}} % arrow\)
\( \newcommand{\vectorAt}[1]{\vec{\text{#1}}} % arrow\)
\( \newcommand{\vectorB}[1]{\overset { \scriptstyle \rightharpoonup} {\mathbf{#1}} } \)
\( \newcommand{\vectorC}[1]{\textbf{#1}} \)
\( \newcommand{\vectorD}[1]{\overrightarrow{#1}} \)
\( \newcommand{\vectorDt}[1]{\overrightarrow{\text{#1}}} \)
\( \newcommand{\vectE}[1]{\overset{-\!-\!\rightharpoonup}{\vphantom{a}\smash{\mathbf {#1}}}} \)
Viruses are typically described as obligate intracellular parasites , acellular infectious agents that require the presence of a host cell in order to multiply. Viruses that have been found to infect all types of cells – humans, animals, plants, bacteria, yeast, archaea, protozoa…some scientists even claim they have found a virus that infects other viruses! But that is not going to happen without some cellular help.
Virus Characteristics
Viruses can be extremely simple in design, consisting of nucleic acid surrounded by a protein coat known as a capsid . The capsid is composed of smaller protein components referred to as capsomers . The capsid+genome combination is called a nucleocapsid .
Viruses can also possess additional components, with the most common being an additional membranous layer that surrounds the nucleocapsid, called an envelope . The envelope is actually acquired from the nuclear or plasma membrane of the infected host cell, and then modified with viral proteins called peplomers . Some viruses contain viral enzymes that are necessary for infection of a host cell and coded for within the viral genome. A complete virus, with all the components needed for host cell infection, is referred to as a virion .
Virus Characteristics, Image created by Ben Taylor , Public Domain, Via Wikipedia commons
Virus Genome
While cells contain double-stranded DNA for their genome, viruses are not limited to this form. While there are dsDNA viruses, there are also viruses with single-stranded DNA ( ssDNA ), double-stranded RNA ( dsRNA ), and single-stranded RNA ( ssRNA ). In this last category, the ssRNA can either positive-sense ( +ssRNA , meaning it can transcribe a message, like mRNA) or it can be negative-sense ( -ssRNA , indicating that it is complementary to mRNA). Some viruses even start with one form of nucleic acid in the nucleocapsid and then convert it to a different form during replication.
Virus Structure
Viral nucleocapsids come in two basic shapes, although the overall appearance of a virus can be altered by the presence of an envelope, if present. Helical viruses have an elongated tube-like structure, with the capsomers arranged helically around the coiled genome. Icosahedral viruses have a spherical shape, with icosahedral symmetry consisting of 20 triangular faces. The simplest icosahedral capsid has 3 capsomers per triangular face, resulting in 60 capsomers for the entire virus. Some viruses do not neatly fit into either of the two previous categories because they are so unusual in design or components, so there is a third category known as complex viruses . Examples include the poxvirus with a brick-shaped exterior and a complicated internal structure, as well as bacteriophage with tail fibers attached to an icosahedral head.
Virus Replication Cycle
While the replication cycle of viruses can vary from virus to virus, there is a general pattern that can be described, consisting of five steps:
- Attachment – the virion attaches to the correct host cell.
- Penetration or Viral Entry – the virus or viral nucleic acid gains entrance into the cell.
- Synthesis – the viral proteins and nucleic acid copies are manufactured by the cells’ machinery.
- Assembly – viruses are produced from the viral components.
- Release – newly formed virions are released from the cell.
Outside of their host cell, viruses are inert or metabolically inactive. Therefore, the encounter of a virion to an appropriate host cell is a random event. The attachment itself is highly specific, between molecules on the outside of the virus and receptors on the host cell surface. This accounts for the specificity of viruses to only infect particular cell types or particular hosts.
Penetration or Viral Entry
Many unenveloped (or naked ) viruses inject their nucleic acid into the host cell, leaving an empty capsid on the outside. This process is termed penetration and is common with bacteriophage, the viruses that infect bacteria. With the eukaryotic viruses, it is more likely for the entire capsid to gain entrance into the cell, with the capsid being removed in the cytoplasm. An unenveloped eukaryotic virus often gains entry via endocytosis , where the host cell is compelled to engulf the capsid resulting in an endocytic vesicle. An enveloped eukaryotic virus gains entrance for its nucleocapsid when the viral envelope fuses with the host cell membrane, pushing the nucleocapsid past the cell membrane. If the entire nucleocapsid is brought into the cell then there is an uncoating process to strip away the capsid and release the viral genome.
The synthesis stage is largely dictated by the type of viral genome, since genomes that differ from the cell’s dsDNA genome can involve intricate viral strategies for genome replication and protein synthesis. Viral specific enzymes, such as RNA-dependent RNA polymerases, might be necessary for the replication process to proceed. Protein production is tightly controlled, to insure that components are made at the right time in viral development.
The complexity of viral assembly depends upon the virus being made. The simplest virus has a capsid composed of 3 different types of proteins, which self-assembles with little difficulty. The most complex virus is composed of over 60 different proteins, which must all come together in a specific order. These viruses often employ multiple assembly lines to create the different viral structures and then utilize scaffolding proteins to put all the viral components together in an organized fashion.
The majority of viruses lyse their host cell at the end of replication, allowing all the newly formed virions to be released to the environment. Another possibility, common for enveloped viruses, is budding , where one virus is released from the cell at a time. The cell membrane is modified by the insertion of viral proteins, with the nucleocapsid pushing out through this modified portion of the membrane, allowing it to acquire an envelope.
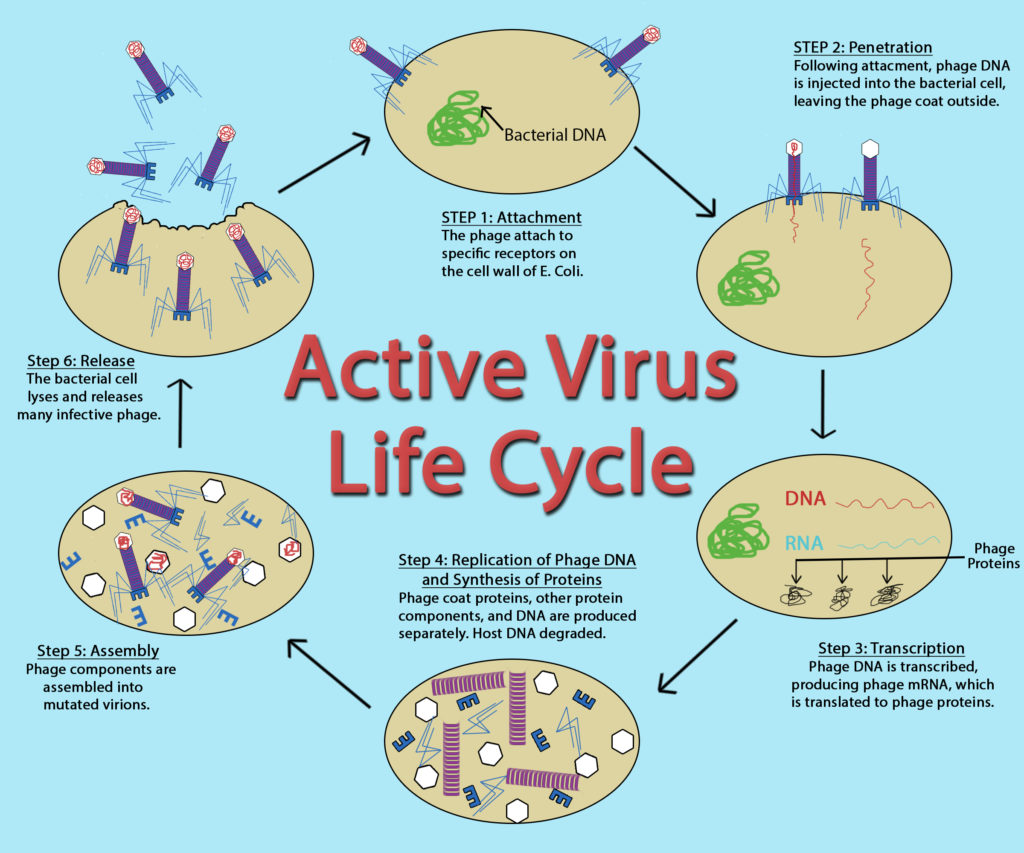
Active Virus Life Cycle by John Kellogg Via OER at Oregon State University
Bacteriophage
Viruses that infect bacteria are known as bacteriophage or phage . A virulent phage is one that always lyses the host cell at the end of replication, after following the five steps of replication described above. This is called the lytic cycle of replication.
There are also temperate phage , viruses that have two options regarding their replication. Option 1 is to mimic a virulent phage, following the five steps of replication and lysing the host cell at the end, referred to as the lytic cycle. But temperate phage differ from virulent phage in that they have another choice: Option 2, where they remain within the host cell without destroying it. This process is known as lysogeny or the lysogenic cycle of replication.
A phage employing lysogeny still undergoes the first two steps of a typical replication cycle, attachment and penetration. Once the viral DNA has been inserted into the cell it integrates with the host DNA, forming a prophage . The infected bacterium is referred to as a lysogen or lysogenic bacterium . In this state, the virus enjoys a stable relationship with its host, where it does not interfere with host cell metabolism or reproduction. The host cell enjoys immunity from reinfection from the same virus.
Exposure of the host cell to stressful conditions (i.e. UV irradiation) causes induction , where the viral DNA excises from the host cell DNA. This event triggers the remaining steps of the lytic cycle, synthesis, maturation, and release, leading to lysis of the host cell and release of newly formed virions.
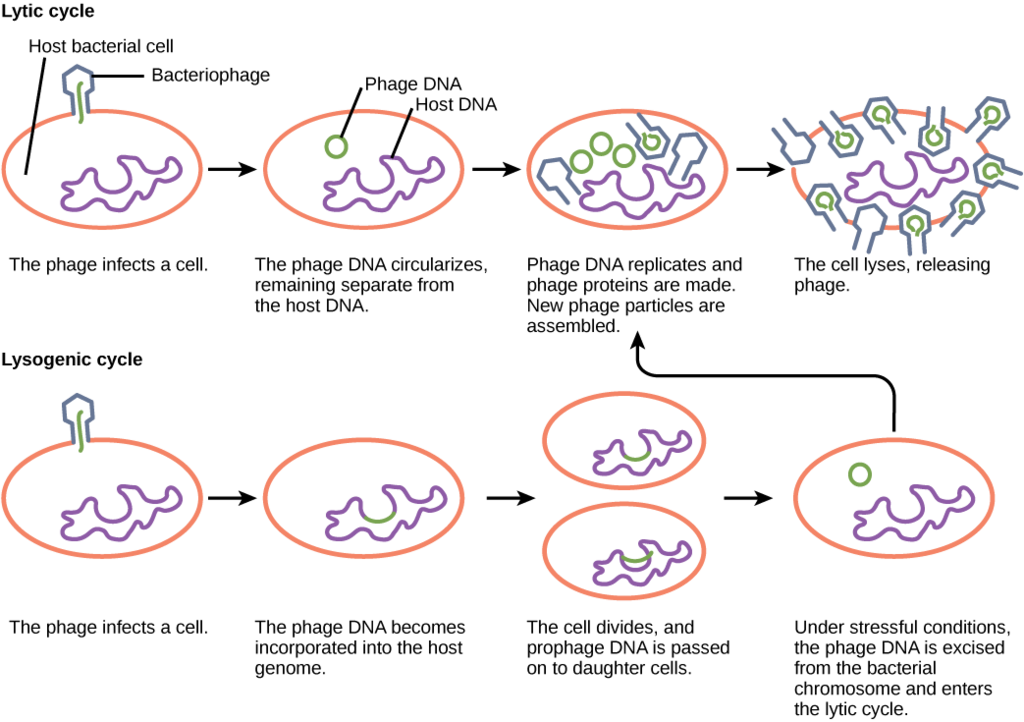
Lytic Cycle Versus Lysogenic Cycle of Replication. OpenStax, Virus Infections and Hosts. OpenStax CNX. Apr 11, 2013 http://cnx.org/contents/7cbd15ad-5bff-4678-a99f-85fd579e070c@3 .
So, what dictates the replication type that will be used by a temperate phage? If there are plenty of host cells around, it is likely that a temperate phage will engage in the lytic cycle of replication, leading to a large increase in viral production. If host cells are scarce, a temperate phage is more likely to enter lysogeny, allowing for viral survival until host cell numbers increase. The same is true if the number of phage in an environment greatly outnumber the host cells, since lysogeny would allow for host cells numbers to rebound, ensuring long term viral survival.
Lysogens can experience a benefit from lysogeny as well, since it can result in lysogenic conversion , a situation where the development of a prophage leads to a change in the host’s phenotype. One of the best examples of this is for the bacterium Corynebacterium diphtheriae , the causative agent of diphtheria. The diphtheria toxin that causes the disease is encoded within the phage genome, so only C. diphtheriae lysogens cause diphtheria.
Eukaryotic Viruses
Eukaryotic viruses can cause one of four different outcomes for their host cell. The most common outcome is host cell lysis, resulting from a virulent infection (essentially the lytic cycle of replication seen in phage). Some viruses can cause a latent infection , co-existing peacefully with their host cells for years (much like a temperate phage during lysogeny). Some enveloped eukaryotic viruses can also be released one at a time from an infected host cell, in a type of budding process, causing a persistent infection . Lastly, certain eukaryotic viruses can cause the host cell to transform into a malignant or cancerous cell, a mechanism known as transformation .
Viruses and Cancer
There are many different causes of cancer, or unregulated cell growth and reproduction. Some known causes include exposure to certain chemicals or UV light. There are also certain viruses that have a known associated with the development of cancer. Such viruses are referred to as oncoviruses . Oncoviruses can cause cancer by producing proteins that bind to host proteins known as tumor suppressor proteins , which function to regulate cell growth and to initiate programmed cell death, if needed. If the tumor suppressor proteins are inactivated by viral proteins then cells grow out of control, leading to the development of tumors and metastasis, where the cells spread throughout the body.
virus, obligate intracellular parasite, capsid, bacteriophage, capsomere, nucleocapsid, envelope, peplomer, virion, dsDNA, ssDNA, dsRNA, +ssRNA, -ssRNA, helical viruses, icosahedral viruses, complex viruses, attachment, penetration, viral entry, synthesis, assembly, release, naked virus, endocytosis, budding, bacteriophage, phage, virulent phage, lytic cycle, temperate phage, lysogeny, lysogenic cycle, prophage, lysogen, lysogenic bacterium, induction, lysogenic conversion, virulent infection, latent infection, persistent infection, transformation, oncovirus, tumor suppressor proteins.
Essential Questions/Objectives
- What are the general properties of a virus?
- What is the size range of viruses? How do they compare, size-wise, to bacteria?
- What is the general structure of viruses? What are the different components?
- What viral shapes exist?
- How do envelopes and enzymes relate to viruses?
- What types of viral genomes exist?
- What are the steps of viral multiplication? What is happening at each step? How do Bacterial/Archaeal viruses differ from eukaryotic viruses, in regards to multiplication?
- What are the 2 types of viral infection found in Bacteria/Archaea ? What are the specific terms associated with viral infection of bacterial/archaeal cells?
- What are the 4 types of viral infection found in eukaryotes?
- How do some viruses cause cancer?
Exploratory Questions (OPTIONAL)
- What is the largest bacterium or archaean ever discovered? What is the smallest eukaryote ever discovered?
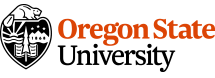
8 Introduction to Viruses
Viruses are typically described as obligate intracellular parasites , acellular infectious agents that require the presence of a host cell in order to multiply. Viruses that have been found to infect all types of cells – humans, animals, plants, bacteria, yeast, archaea, protozoa…some scientists even claim they have found a virus that infects other viruses! But that is not going to happen without some cellular help.
Virus Characteristics
Viruses can be extremely simple in design, consisting of nucleic acid surrounded by a protein coat known as a capsid . The capsid is composed of smaller protein components referred to as capsomers . The capsid+genome combination is called a nucleocapsid .
Viruses can also possess additional components, with the most common being an additional membranous layer that surrounds the nucleocapsid, called an envelope . The envelope is actually acquired from the nuclear or plasma membrane of the infected host cell, and then modified with viral proteins called peplomers . Some viruses contain viral enzymes that are necessary for infection of a host cell and coded for within the viral genome. A complete virus, with all the components needed for host cell infection, is referred to as a virion .
Virus Genome
While cells contain double-stranded DNA for their genome, viruses are not limited to this form. While there are dsDNA viruses, there are also viruses with single-stranded DNA ( ssDNA ), double-stranded RNA ( dsRNA ), and single-stranded RNA ( ssRNA ). In this last category, the ssRNA can either positive-sense ( +ssRNA , meaning it can transcribe a message, like mRNA) or it can be negative-sense ( -ssRNA , indicating that it is complementary to mRNA). Some viruses even start with one form of nucleic acid in the nucleocapsid and then convert it to a different form during replication.
Virus Structure
Viral nucleocapsids come in two basic shapes, although the overall appearance of a virus can be altered by the presence of an envelope, if present. Helical viruses have an elongated tube-like structure, with the capsomers arranged helically around the coiled genome. Icosahedral viruses have a spherical shape, with icosahedral symmetry consisting of 20 triangular faces. The simplest icosahedral capsid has 3 capsomers per triangular face, resulting in 60 capsomers for the entire virus. Some viruses do not neatly fit into either of the two previous categories because they are so unusual in design or components, so there is a third category known as complex viruses . Examples include the poxvirus with a brick-shaped exterior and a complicated internal structure, as well as bacteriophage with tail fibers attached to an icosahedral head.
Virus Replication Cycle
While the replication cycle of viruses can vary from virus to virus, there is a general pattern that can be described, consisting of five steps:
- Attachment – the virion attaches to the correct host cell.
- Penetration or Viral Entry – the virus or viral nucleic acid gains entrance into the cell.
- Synthesis – the viral proteins and nucleic acid copies are manufactured by the cells’ machinery.
- Assembly – viruses are produced from the viral components.
- Release – newly formed virions are released from the cell.
Outside of their host cell, viruses are inert or metabolically inactive. Therefore, the encounter of a virion to an appropriate host cell is a random event. The attachment itself is highly specific, between molecules on the outside of the virus and receptors on the host cell surface. This accounts for the specificity of viruses to only infect particular cell types or particular hosts.

Penetration or Viral Entry
Many unenveloped (or naked ) viruses inject their nucleic acid into the host cell, leaving an empty capsid on the outside. This process is termed penetration and is common with bacteriophage, the viruses that infect bacteria. With the eukaryotic viruses, it is more likely for the entire capsid to gain entrance into the cell, with the capsid being removed in the cytoplasm. An unenveloped eukaryotic virus often gains entry via endocytosis , where the host cell is compelled to engulf the capsid resulting in an endocytic vesicle, allowing the virus access to the cell contents. An enveloped eukaryotic virus gains entrance for its nucleocapsid through membrane fusion , where the viral envelope fuses with the host cell membrane, pushing the nucleocapsid past the cell membrane. If the entire nucleocapsid is brought into the cell then there is an uncoating process to strip away the capsid and release the viral genome.
The synthesis stage is largely dictated by the type of viral genome, since genomes that differ from the cell’s dsDNA genome can involve intricate viral strategies for genome replication and protein synthesis. Viral specific enzymes, such as RNA-dependent RNA polymerases, might be necessary for the replication process to proceed. Protein production is tightly controlled, to insure that components are made at the right time in viral development.
The complexity of viral assembly depends upon the virus being made. The simplest virus has a capsid composed of 3 different types of proteins, which self-assembles with little difficulty. The most complex virus is composed of over 60 different proteins, which must all come together in a specific order. These viruses often employ multiple assembly lines to create the different viral structures and then utilize scaffolding proteins to put all the viral components together in an organized fashion.
The majority of viruses lyse their host cell at the end of replication, allowing all the newly formed virions to be released to the environment. Another possibility, common for enveloped viruses, is budding , where one virus is released from the cell at a time. The cell membrane is modified by the insertion of viral proteins, with the nucleocapsid pushing out through this modified portion of the membrane, allowing it to acquire an envelope.
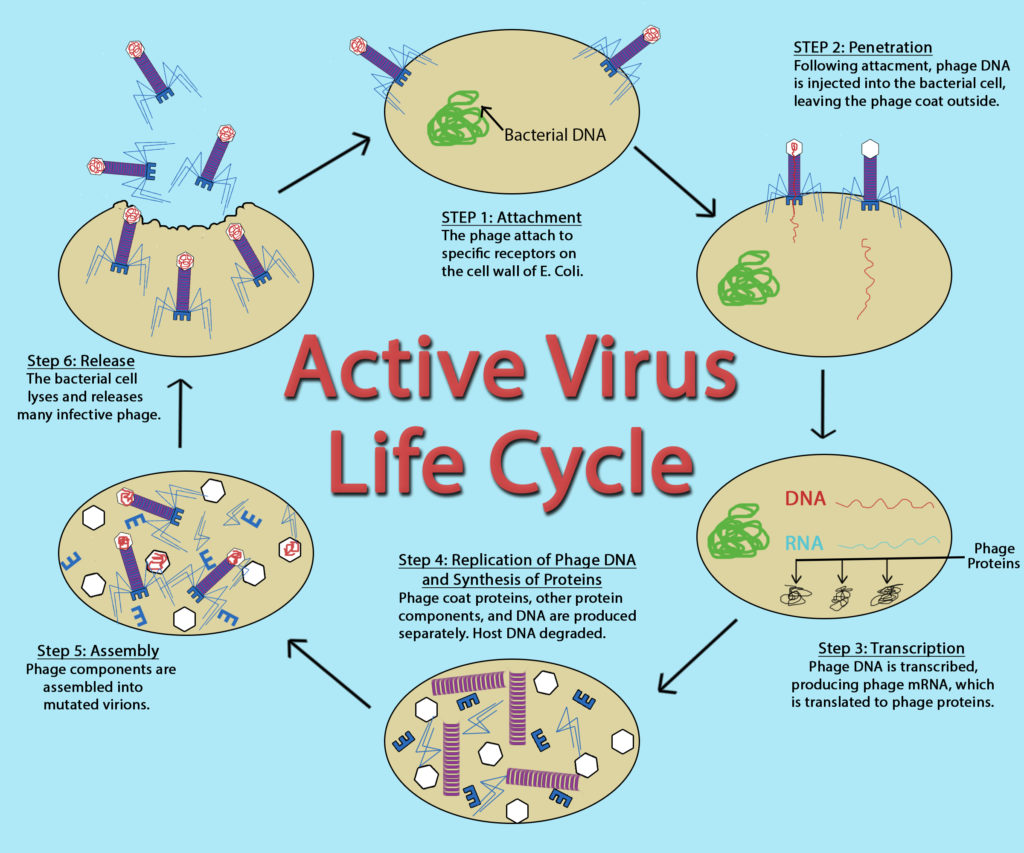
Bacteriophage
Viruses that infect bacteria are known as bacteriophage or phage . A virulent phage is one that always lyses the host cell at the end of replication, after following the five steps of replication described above. This is called the lytic cycle of replication.
There are also temperate phage , viruses that have two options regarding their replication. Option 1 is to mimic a virulent phage, following the five steps of replication and lysing the host cell at the end, referred to as the lytic cycle. But temperate phage differ from virulent phage in that they have another choice: Option 2, where they remain within the host cell without destroying it. This process is known as lysogeny or the lysogenic cycle of replication.
A phage employing lysogeny still undergoes the first two steps of a typical replication cycle, attachment and penetration. Once the viral DNA has been inserted into the cell it integrates with the host DNA, forming a prophage . The infected bacterium is referred to as a lysogen or lysogenic bacterium . In this state, the virus enjoys a stable relationship with its host, where it does not interfere with host cell metabolism or reproduction. The host cell enjoys immunity from reinfection from the same virus.
Exposure of the host cell to stressful conditions (i.e. UV irradiation) causes induction , where the viral DNA excises from the host cell DNA. This event triggers the remaining steps of the lytic cycle, synthesis, maturation, and release, leading to lysis of the host cell and release of newly formed virions.
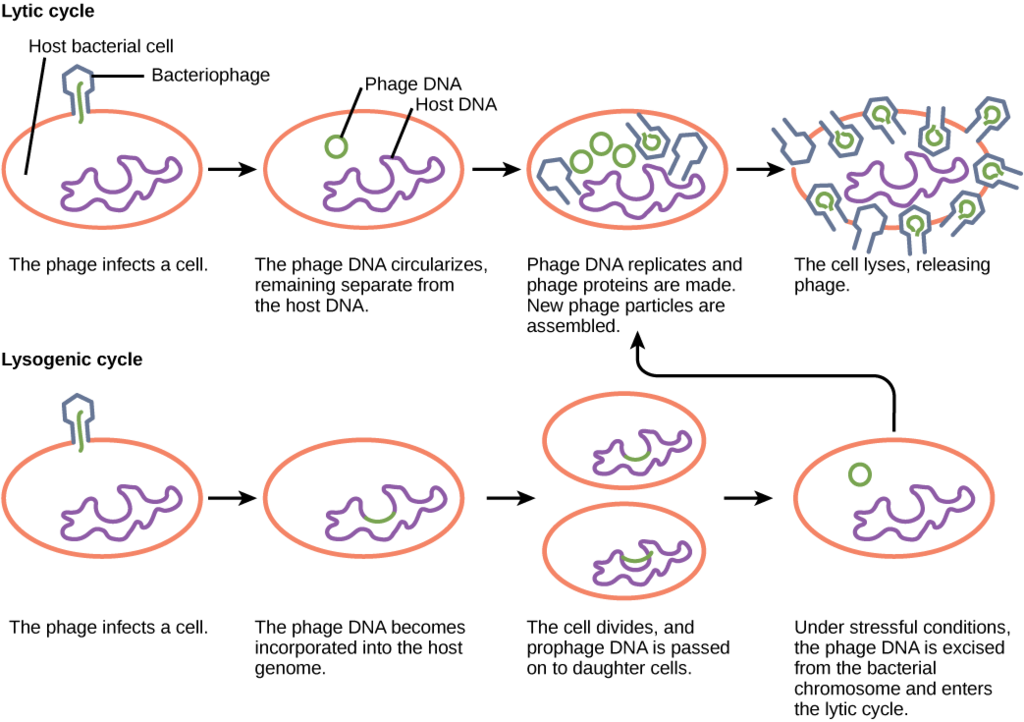
So, what dictates the replication type that will be used by a temperate phage? If there are plenty of host cells around, it is likely that a temperate phage will engage in the lytic cycle of replication, leading to a large increase in viral production. If host cells are scarce, a temperate phage is more likely to enter lysogeny, allowing for viral survival until host cell numbers increase. The same is true if the number of phage in an environment greatly outnumber the host cells, since lysogeny would allow for host cells numbers to rebound, ensuring long term viral survival.
Lysogens can experience a benefit from lysogeny as well, since it can result in lysogenic conversion , a situation where the development of a prophage leads to a change in the host’s phenotype. One of the best examples of this is for the bacterium Corynebacterium diphtheriae , the causative agent of diphtheria. The diphtheria toxin that causes the disease is encoded within the phage genome, so only C. diphtheriae lysogens cause diphtheria.
Eukaryotic Viruses
Eukaryotic viruses can cause one of four different outcomes for their host cell. The most common outcome is host cell lysis, resulting from a virulent infection (essentially the lytic cycle of replication seen in phage). Some viruses can cause a latent infection , inserting their viral DNA into the host cell genome, allowing them to co-exist peacefully with their host cells for long periods of time (much like a temperate phage during lysogeny). Some enveloped eukaryotic viruses can also be released one at a time from an infected host cell, in a type of budding process, causing a persistent infection . Lastly, certain eukaryotic viruses can cause the host cell to transform into a malignant or cancerous cell, a mechanism known as transformation .
Viruses and Cancer
There are many different causes of cancer, or unregulated cell growth and reproduction. Some known causes include exposure to certain chemicals or UV light. There are also certain viruses that have a known associated with the development of cancer. Such viruses are referred to as oncoviruses . Oncoviruses can cause cancer by producing proteins that bind to host proteins known as tumor suppressor proteins , which function to regulate cell growth and to initiate programmed cell death, if needed. If the tumor suppressor proteins are inactivated by viral proteins then cells grow out of control, leading to the development of tumors and metastasis, where the cells spread throughout the body.
virus, obligate intracellular parasite, capsid, bacteriophage, capsomere, nucleocapsid, envelope, peplomer, virion, dsDNA, ssDNA, dsRNA, +ssRNA, -ssRNA, helical viruses, icosahedral viruses, complex viruses, attachment, penetration, viral entry, synthesis, assembly, release, naked virus, endocytosis, membrane fusion, budding, bacteriophage, phage, virulent phage, lytic cycle, temperate phage, lysogeny, lysogenic cycle, prophage, lysogen, lysogenic bacterium, induction, lysogenic conversion, virulent infection, latent infection, persistent infection, transformation, oncovirus, tumor suppressor proteins.
Study Questions
- What are the general properties of a virus?
- What is the size range of viruses? How do they compare, size-wise, to bacteria?
- What is the general structure of viruses? What are the different components?
- What viral shapes exist?
- How do envelopes and enzymes relate to viruses?
- What types of viral genomes exist?
- What are the 5 basic steps of viral replication? What happens at each step? How do bacterial/archaeal viruses differ from eukaryotic viruses, in regards to replication details?
- What are the 2 types of viral infection found in Bacteria/Archaea ? What are the specific terms associated with viral infection of bacterial/archaeal cells?
- What are the 4 types of viral infection found in eukaryotes?
- How do some viruses cause cancer?
Exploratory Questions (OPTIONAL)
- What is the largest bacterium or archaean ever discovered? What is the smallest eukaryote ever discovered?
General Microbiology Copyright © 2019 by Linda Bruslind is licensed under a Creative Commons Attribution-NonCommercial 4.0 International License , except where otherwise noted.

An official website of the United States government
The .gov means it’s official. Federal government websites often end in .gov or .mil. Before sharing sensitive information, make sure you’re on a federal government site.
The site is secure. The https:// ensures that you are connecting to the official website and that any information you provide is encrypted and transmitted securely.
- Publications
- Account settings
Preview improvements coming to the PMC website in October 2024. Learn More or Try it out now .
- Advanced Search
- Journal List

Virus classification – where do you draw the line?
Peter simmonds.
1 Nuffield Department of Medicine, University of Oxford, Peter Medawar Building, South Parks Road, Oxford, OX1 3SY UK
Pakorn Aiewsakun
2 Department of Microbiology, Faculty of Science, Mahidol University, Bangkok, 10400 Thailand
High-throughput sequencing (HTS) and its use in recovering and assembling novel virus sequences from environmental, human clinical, veterinary and plant samples has unearthed a vast new catalogue of viruses. Their classification, known by their sequences alone, sets a major challenge to traditional virus taxonomy, especially at the family and species levels, which have been historically based largely on descriptive taxon definitions. These typically entail some knowledge of their phenotypic properties, including replication strategies, virion structure and clinical and epidemiological features, such as host range, geographical distribution and disease outcomes. Little to no information on these attributes is available, however, for viruses identified in metagenomic datasets. If such viruses are to be included in virus taxonomy, their assignments will have to be guided largely or entirely by metrics of genetic relatedness. The immediate problem here is that the International Committee on Taxonomy of Viruses (ICTV), an organisation that authorises the taxonomic classification of viruses, provides little or no guidance on how similar or how divergent viruses must be in order to be considered members of new species or new families. We have recently developed a method for scoring genomic (dis)similarity between viruses (Genome Relationships Applied to Virus Taxonomy – GRAViTy) among the eukaryotic and prokaryotic viruses currently classified by the ICTV. At the family and genus levels, we found large-scale consistency between genetic relationships and their taxonomic assignments for eukaryotic viruses of all genome configurations and genome sizes. Family assignments of prokaryotic viruses have, however, been made at a quite different genetic level, and groupings currently classified as sub-families are a much better match to the eukaryotic virus family level. These findings support the ongoing reorganisation of bacteriophage taxonomy by the ICTV Phage Study Group. A rapid and objective means to explore metagenomic viral diversity and make evidence-based assignments for such viruses at each taxonomic layer is essential. Analysis of sequences by GRAViTy provides evidence that family (and genus) assignments of currently classified viruses are largely underpinned by genomic relatedness, and these features could serve as a guide towards an evidence-based classification of metagenomic viruses in the future.
The diversity and classification of viruses
Virus taxonomy is an essential element in the description of viruses and acts as a unified catalogue of their vast diversity and genetic interrelationships. Viruses are assigned in a hierarchy of taxonomic levels by the International Committee on Taxonomy of Viruses (ICTV; https://talk.ictvonline.org/ ). Viral diversity is, however, far greater than that of other organisms, with major differences in their genetic material (RNA or DNA) and configurations (double or single stranded), as well as the orientation of their encoded genes. Viral genomes may, furthermore, be distributed across several segments, sometimes packaged together in a virion, or often in separate virus particles, all of which are needed to infect a cell for replication to occur. Viral genomes come in various sizes, reflecting their diverse replication mechanisms and cellular interactions, as well as the varying structural complexity of their virions. The smallest virus genomes range from less than 2,000 bases, containing two genes, to 2.5 million base pairs, containing over 2,500 genes [ 1 ]. Similarly, there is extraordinary variability in virus particle morphology and size; some virus particles show icosahedral or more complex symmetry, while others may form filamentous, rectangular, bullet, or even bottle-shaped nucleocapsids. Viruses infecting bacteria typically possess “tails” and “spikes”, structural complexes that attach to and pierce the otherwise impermeable bacterial cell wall and injects viral DNA into the cytoplasm.
The taxonomy of cellular organisms, including microorganisms such as bacteria and unicellular fungi, is built on a common evolutionary framework – ultimately all eukaryotes share a last common ancestor, distinct from those of bacteria and archaea representing the other domains of life. These deeper relationships are largely recoverable from their genetic relationships; the phylogeny of core genes, such as those for ribosomal proteins, provides a reasonable representation of their evolutionary origin and divergence many billions of years ago. Unfortunately, the diversity of viruses prevents such a reconstruction of virus evolutionary histories – they lack any equivalent set of universally conserved genes on which to construct a phylogeny [ 9 , 11 , 19 ]. Viruses appear to have appeared on several occasions as parasitic companions of the various prokaryotic and eukaryotic life forms that they infect [ 13 ].
The current taxonomy of viruses has itself gradually evolved since the formation of what is now the ICTV in 1966. The vastly and rapidly expanding knowledge of virus diversity since that time and the advent of molecular methods for virus discovery and genetic characterisation have greatly increased the number of taxa assigned, with the current totals being 9 orders, 131 families, 46 subfamilies, 803 genera and 4,853 species, following the ratification vote after the 49 th ICTV Executive Committee meeting [ 18 ]. Incorporating the hugely diverse collection of evolutionarily related (and perhaps unrelated) groups of viruses into a single, overarching framework represents a considerable and ongoing organisational achievement, and the resources and descriptive catalogues of viruses (9 th and 10 th Report - https://talk.ictvonline.org/ictv-reports/ ) are vital resources underpinning the whole virus classification field.
Nevertheless, you might discover on closer inspection that the unified virus taxonomy is a rather ramshackle construction, with taxonomic assignment rules often being based on quite different and inconsistent criteria between virus groups. For example, the assignment of viruses to the order Herpesvirales is based on their morphology, and is independent of genomic relationships, which do not place their members into a coherent genetic group. Contrastingly, membership of the order Tymovirales is based upon possession of genetically related RNA polymerase genes, irrespective of the highly variable virion structures of their members.
Species-level classification
The species definition is particularly variable between virus groups. This taxonomic level was originally used as the primary division of viruses showing distinct disease patterns, epidemiology and host range. Yellow fever virus , Carnation mottle virus and Salmon pancreas disease virus are typical examples of literally hundreds of such disease-focussed designations. As nucleotide sequence information on classified species gradually accrued in the 1980s-1990s, it became clear that species were typically genetically distinct from each other, but there was no fixed sequence divergence threshold that defined members of the same and different species. As an example of what typifies large numbers of other species assignments throughout the ICTV taxonomy, members of different flavivirus species show pairwise distances in the RNA-dependent RNA polymerase (RdRp) gene of only 1.5% (between the species Israel turkey meningoencephalomyelitis virus and Bagaza virus ), while members of San Perlita virus and Ilheus virus differ by 44%. As an example of even greater discrepancy, members of the species Louping ill virus, which infect grouse and sheep in upland Britain, lie entirely within the clade of the separate species Tick-borne encephalitis virus . The two were defined as separate species based on their marked differences in geographical range, host associations, and pathogenicity, and clearly not their genetic relationships in this case.
It has been entirely possible to maintain what is essentially a phenotypically based system of species assignments for many decades – these assignments do, after all, divide viruses into groups that differ in important medical, veterinary and agricultural properties that serve a major purpose for classification. Flexibility in what defines species is implicit in the polythetic species definition [ 27 , 28 ] developed by Marc Van Regenmortel, in which constellations of properties, none of which would be individually essential for species inclusion or exclusion, are formulated to produce a highly intuitive and effective descriptive definition. Polythetic species definitions, indeed, have provided the basis for ICTV taxonomy assignments for nearly two decades. The difficulty that has arisen from this approach is that, increasingly, viruses are discovered by molecular methods and, most recently, by deep sequencing of environmental and other samples using high-throughput sequencing (HTS) methods. These techniques often simply provide a virus sequence but none of the typical sets of properties that might constitute a polythetic definition.
As HTS methods become increasingly used, and the catalogue of viruses known only by their sequence data continues to expand almost exponentially, genomics-based species assignments are clearly required to accommodate these into the ICTV taxonomy [ 10 , 12 , 21 , 22 , 31 ]. The difficulty is how to assign what often are purely sequence-based species thresholds in a manner that is not purely arbitrary and which is consistent with the taxonomy of other viruses whose phenotypic properties are known. Many opinions and proposals have been put forward on this crucial procedural issue over many years [ 7 , 14 , 29 , 30 ], recently reviewed in reference [ 26 ]. However, in practical terms, all of these boil down to a central and seemingly irreconcilable dilemma – you can’t use descriptive species definitions for viruses where there is nothing to describe except its nucleotide sequence. On the other hand, disallowing sequence-only assignments produces an ICTV taxonomy that fails in its function as a proper catalogue of viral diversity. Sequence-only viruses are still viruses. It is simply that information on their properties has not been collected (yet) – hardly a reason for their permanent exclusion. We suggest that those involved in previous discussions and those expressing new views on the subject take the time to discuss future species definitions for viruses, to critically evaluate the various biological species definitions currently in use and to decide which concepts are most suitable for viruses in the future – the polythetic species is, after all, just one of the over 20-30 species definitions used or proposed in wider biology.
Virologists should not be daunted by the scale of the task ahead in making species assignment to the vast number of viruses identified by deep-sequencing methods. Recent descriptions of potentially tens or hundreds of new species, genus and family assignments for viruses isolated from arthropod and lower vertebrate host taxa [ 20 , 23 , 24 ] are really no different taxonomically from, and are indeed simpler in practical terms than classification tasks elsewhere in biology. A (possibly extreme) example is the still ongoing cataloguing of beetle species, with more than 400,000 species already assigned, each bearing a binomial, Latinised name and perhaps more than half a million to go.
Family-level classification
While the species level of taxonomy is one that best describes what we would consider individual viruses in a broad sense, the family level is the fundamental taxonomic level by which viruses of different kinds are organised. Eukaryotic viruses are currently assigned into a total of 111 families, with distinct genome configurations, virion morphologies, replication strategies and host interactions. Many of the groupings first described in early ICTV reports, generally based on electron microscopy appearance and genomic material, have stood the test of time – herpesviruses, poxviruses and rhabdoviruses are instantly and recognisably distinct morphologically, and their separateness has been verified by detailed analysis of their genome structures and replication mechanisms.
The ICTV taxonomy, however, provides little information that might guide decisions on what is needed to justify the creation of new virus families or orders. The ICTV Code provides this as a definition of a virus family:
A family is a group of genera (whether or not these are organized into subfamilies) sharing certain common characters
building on:
A genus is a group of species sharing certain common characters
which, frankly, is equally uninformative. Although new families have continued to be assigned in the last three decades, there is no real systematic information or guidance on what virological, structural or genomic attributes would support the family-level assignments that have been made, and what may be used in the future. For example, family members must presumably share homologous genes, but how many and how similar? Must they have related genome organisations? Should they look similar by electron microscopy? Most crucially in this “metagenomic” era, are there any family-defining attributes recoverable from a virus sequence alone – or is it essential to visualise particle morphology and to determine some of the physical attributes of the virus, such as host range, cell tropism and pathogenicity, as part of an extended family descriptive definition? The ICTV offers no guidance. Perhaps as a direct result of this uncertainty, picorna-like, flavi-like, circo-like and a vast range of other (family)–like virus descriptions are to be found in literally hundreds of papers indexed by PubMed. In many cases, it is as if their authors, too, lacked the information or confidence to assign new families for newly discovered viruses. Guidance on this issue and, ideally, a better-defined set of criteria for family assignments seems long overdue – this is indeed required if the ICTV is going to embrace the growing dataset of virus genome sequences from metagenomic datasets.
Development of GRAViTy
It was with this background that we set out to examine whether there were any consistent genomic attributes of eukaryotic virus families that correlated with their current family-level ICTV taxonomy relationships [ 5 ]. Investigating this involved extracting and analysing a wide range of genetic metrics from the set of currently classified viruses, including organisational features (gene complements and gene orders), possession of homologous genes and their amino acid sequence identity. These features were then systematically evaluated for their ability to recover the taxonomy of all currently classified eukaryotic viruses in the ICTV Master Species List [ 5 ]. The logic of this exercise was based on the premise that genome features that were informative and predictive of taxonomic relationships could then be extracted from currently unassigned viruses from their sequences alone (including the vast number of “-like” viruses), and informed decisions on their assignments could be made. While this represents an entirely bioinformatic approach, it ensures that any family or other-level assignments of sequence-only viruses are broadly consistent with existing taxonomic placements of viruses classified by other means.
This method, “ G enome R elationships A pplied to Vi rus T axonom y ” (GRAViTy), was applied to the complete list of 3,854 classified eukaryotic viruses with complete genome sequence data. Information such as gene contents, orientations and protein profiles were extracted from each genome sequence and systematically compared through the calculation of a composite generalised Jaccard (CGJ) distance, a metric that captures and weights the contributions of different genome features between pairs of viruses. This use of multiple features extracted from viral sequences contrasts with traditional phylogenetic methods, in which virus relationships are often calculated from small, highly conserved portions of their genomes, such as the catalytic core of the RdRp gene.
Pairwise distances between members of particular virus groups, such as dsDNA viruses (Baltimore group I), for example, can be visualised as a heat map (Fig. 1 ) or as dendrograms (Fig. 2 ). The highly encouraging finding from this analysis was the close concordance between sequence groupings and their current ICTV assignments into families for viruses of all configurations (dsDNA, ssDNA, dsRNA, (+)ssRNA, (-)ssRNA and retro-transcribing viruses). GRAViTy showed 95.7%-100% (mean, 99.1%) sensitivity and 99.3%-100% (mean, 99.8%) specificity for the virus assignments with the current virus taxonomy. These associations are all the more striking for being derived from genome features without pre-selection for which ones might be considered to be informative, and furthermore being generated without having to construct multiple sequence alignments with its attendant but often hidden assumptions and problems. The analysis revealed a primary division of viruses at the family level that, with very few exceptions, was readily identifiable as tight clusters with ≥70% bootstrap support in the dendrogram (Fig. 2 ). The small number of exceptions in other Baltimore groups were in themselves often highly informative – the separation of rubella virus (genus Rubivirus ) from the rest of the family Togaviridae concurs with a variety of other evidence that it should be re-classified into a separate family. Some virus families turned out to be far more diverse and often polyphyletic, including Rhabdoviridae and Reoviridae, and these represent future candidates for reorganisation of their taxonomy.

Heat maps of CGJ distances between dsDNA. Pairwise CGJ distances were computed between each sequence plotted as a heat map using colour-coded points (see scale on the left of the figure). The light grey solid lines indicate boundaries between each virus taxonomic group. Annotations for each virus family and order are shown on the axes. This figure has been reproduced from reference [ 5 ]

Virus dendrograms based on CGJ distances. UPGMA dendrograms were constructed from pairwise distance matrices, and the tips are labelled with current ICTV family and genus assignments. Virus taxonomy at the order level is also shown to the right of the dendrograms. The scale bar for the CGJ distance is shown at the bottom. Bootstrap clade support values of ≥ 30% are shown on the branches. Values ≥ 70% are in black, otherwise are in grey. Bootstrap support values for collapsed clades are shown regardless of the values. This figure has been modified from Fig. 2 in reference [ 5 ]
With this newly developed ability to predict family memberships from genomic sequences alone, we next analysed large datasets of virus sequences derived from metagenomic datasets to determine the extent to which they might be classified as new potential families, or as members of existing ones. Using the same metrics that differentiated virus families in each Baltimore group, we were able to provisionally assign viruses in this combined metagenomic dataset as four new families of ssDNA viruses, four dsRNA virus families, potentially as many as 101 new family-level groupings of (+)ssRNA viruses and 16 new (-)ssRNA families. Clearly, these results are preliminary, and conclusions about such a large number of new families require corroboration with other methods, including RdRp phylogenies in the case of future RNA viruses, as is the extension of classification to genus and species levels. What we can say, however, is that future assignments will certainly approximate to relationships that GRAViTy has shown. Furthermore, the new frameworks for each Baltimore group depict sets of virus relationships that are consistent with and extend virus taxonomy in a manner that effectively follows the rules used in the current classification.
Bacterial and archaeal virus (phage) taxonomy
Viruses infecting bacteria and archaea are extraordinarily abundant and diverse. Almost all of the currently classified bacterial viruses are, however, assigned to just three families of tailed phages, namely the Myoviridae , Podoviridae and Siphoviridae [ 2 ], a division based upon their distinct morphologies (myo-: long contractile, sipho-: long non-contractile and podo-: short tails). While it has been appreciated for many years that members of these families are highly diverse genetically, there has been little to no attempt to match these family assignment categories to those used for eukaryotic viruses. There is, consequently, no real idea of the extent to which bacteriophage assignments are taxonomically equivalent or not. This may not have mattered too much when different and largely independent scientific communities were engaged in their separate phage and eukaryotic virus research programmes, but the advent of metagenomic virus sequence data challenges this division. Bacterial, archaeal, and eukaryotic viruses abound in environmental samples that typically generate the largest metagenomic datasets – how can there be different rules for their classification when their hosts are not necessarily known?
We addressed this question directly through analysis of sequence datasets by GRAViTy that incorporated both eukaryotic and prokaryotic viruses in Baltimore groups I-IV (dsDNA, ssDNA, dsRNA and (+)ssRNA) [ 4 ]. This analysis revealed quite different sequence relationships, particularly between those of members of the tailed phage families Siphoviridae, Myoviridae and Podoviridae (assigned to the order Caudovirales ) and those of eukaryotic viruses. Members of the same phage family were generally far more divergent from each other and, despite their morphological similarities, frequently possessed genes that showed no detectable homology with each other (Fig. 3 ). If we were to apply the same metric of genetic relatedness observed between eukaryotic virus families, we might split the Caudovirales into as many as 70 family-level groupings and a further 37 groups comprising currently unclassified bacteriophage sequences available in public databases. These totals are, nevertheless, far more consistent with their own genetic diversity and that of the vast range of bacterial hosts they infect.

UPGMA dendrogram of classified and unclassified dsDNA viruses (Baltimore group I) based on CGJ distances. The dendrogram is divided into six separate lines to represent the 139 clades present in the dataset. Tips are labelled with genus for members of the Caudovirales ; abbreviated as S: Siphoviridae , M: Myoviridae , and P: Podoviridae , and by family/genus for other bacterial, eukaryotic and archaeal viruses, or by accession number codes for unclassified viruses. The scale bar for CGJ distance is shown at the left of each line, and the 0.8 threshold that corresponds to eukaryotic family groupings is shown as a grey dotted line. Bootstrap re-sampling was performed with pruned signature tables as described previously [ 5 ]. Clades were coloured based on host origin according to the key; those containing both classified and unclassified (U) sequences are shown in a lighter shade. The 39 new candidate unassigned taxonomic units (UTUs) arising from the inclusion of current unclassified viruses are shaded in light blue. This figure has been reproduced from reference [ 4 ]
Examining sequence relationships a little further, it was apparent that the subfamily-level groupings among tailed phages were generally more consistent with family assignments of eukaryotic viruses where these have been made (Fig. 4 ). These findings fully support ongoing reclassifications by the ICTV of subfamilies, such as Spounavirinae and Vi1virus taxa, as new virus families based on genome relationships rather than phonotypic properties, such as virion appearance and presumed host [ 6 , 15 , 16 ]. The traditional morphology-based classifications of prokaryotic viruses are, indeed, increasingly untenable even in principle, since the bulk of new phage sequences to be classified derive from metagenomic datasets, where few or no phenotypic attributes are available.

Dendrogram of members of the order Caudovirales that have subfamily assignments. Taxa are colour coded for family (see key). Minor discrepancies between genus assignments and phylogeny are shown in red circles. This figure has been reproduced from reference [ 4 ]
Concluding remarks
The ICTV is committed to the incorporation of viruses known only by their genome sequence into the current taxonomy [ 3 , 25 ]. This is a logical step if the ITCV taxonomy is to remain relevant as a guide to viral diversity; viral sequences reconstructed from HTS data represent viruses as much as those whose phenotypic properties are known (at least in part). However, as reviewed previously [ 26 ], classifications built on sequences alone directly challenge many of the concepts and assumptions of polythetic and other descriptive definitions of virus species.
They also reveal gaping holes in how the various taxonomic levels are defined – the ICTV code provides no indication of how different viruses have to be, either in terms of their phenotypic properties or in their genetic relatedness, to be considered members of the same or different species, genus or family. Given the importance of the family level in virus taxonomy, it is remarkable that there is no information about what features might define a family and enable new families to be assigned in a consistent way. The application of bioinformatic programs like GRAViTy, vContact and others [ 4 , 5 , 8 , 17 ] will be important in documenting those genomic features that underlie this and other taxonomic levels of currently classified eukaryotic and prokaryotic viruses. Such approaches are essential if future sequence-only assignments are to be made from a strong evidence base. While we can all foresee a future with a vastly expanded taxonomy of viruses, we think we should also preserve and respect as much of the current taxonomy as we can – the delineation of how current taxonomy works at a genomic level is an important initial step in this process.
Acknowledgements
The authors would like to thank members of the ICTV Executive Committee for valuable discussion of classification challenges and the need for bioinformatic solutions.
This review was funded by a Wellcome Trust Bioresource Grant (WT108418AIA).
Compliance with ethical standards
Conflict of interest.
The authors declare that they have no competing interests.
Contributor Information
Peter Simmonds, Email: [email protected] .
Pakorn Aiewsakun, Email: [email protected] .

IMAGES
VIDEO
COMMENTS
Virus Characteristics. Viruses can be extremely simple in design, consisting of nucleic acid surrounded by a protein coat known as a capsid.The capsid is composed of smaller protein components referred to as capsomers.The capsid+genome combination is called a nucleocapsid.. Viruses can also possess additional components, with the most common being an additional membranous layer that surrounds ...
-Poliovirus, rabies virus • The type of disease -Murine leukemia virus • Geographic locations -Sendai virus, Coxsackie virus • Their discovers -Epstein-Barr virus • How they were originally thought to be contracted -Dengue virus ("evil spirit"), Influenza virus (the "influence" of bad air) • Combinations of the above
Components of viruses -. A virion is an infectious virus particle - not all virus particles are infectious. Viruses are composed of a nucleic acid, RNA or DNA - never both. All viruses have a protein coat or shell that surrounds and protects the nucleic acid core. Some viruses have a lipid envelope or membrane surrounding a nucleocapsid core.
Some viruses, for example, HIV and herpes simplex virus (HSV), cause both lytic and latent infections (Chapter 5). Some viruses infect bacteria, and the occurrence of such viral infections has led to considerations of viruses as therapeutic agents. For example, bacteriophages, viruses that speci cally infect bacteria, have
Viruses are small obligate intracellular parasites, which by definition contain either a RNA or DNA genome surrounded. by a protective, virus-coded prot ein coat. Viruses may be viewed as mobile ...
Growth of virus on embryonated eggs. This method is used for the cultivation of more than 30 species of viruses. The material containing the virus is inoculated into the amnion, allantois, yolk sac and others. After inoculation of the chick embryo, it is incubated and examined daily for virus growth.
1. Viruses may cause chronic, persistent infection with continuous viral replication in the face of an immune response. Examples include HIV, hepatitis B virus and hepatitis C virus. Some viruses may demonstrate persistent infection in immune compromised hosts. These include the herpesviruses, human papillomavirus and rubella virus, among ...
discovery of viruses. • Organize and represent data showing the differences between viruses and bacteria. • Identify who discovered viruses. DOK 1 - 3 OVERVIEW In this lesson, students learn about the differences between viruses and bacteria. The aim is for students to understand (a) that disease arises from different kinds of agents and (b)
Click & Learn Educator Materials. OVERVIEW. SARS-CoV-2 is a virus that, starting in 2020, has caused the largest global pandemic in recent history. The disease caused by this virus, called COVID-19, has affected millions of people worldwide. Biology of SARS-CoV-2 is a four-part animation series that explores the biology of the virus, including ...
These are mediated primarily through interactions between structured domains (64.1%) and involve 7,463 viral proteins from 990 viruses and 5,749 human proteins. The majority (76.8%) of predicted viral- human PPIs mediated by domain-domain contacts are based on experimentally solved interaction complexes that do not involveaviralprotein ...
Reading assignment: Chap. 15. Virus particles self-assemble from coat monomers membrane proteins themselves or with an inner symmetric protein layer. Non- ... viruses are mainly responsible for the shape and size of the virus particles and are able to form five-fold, three-fold and two-fold contacts. When multiples of
current infection (presence of virus) during the acute phase of disease. NAAT test time is between 13 minutes and 3 hours and require specific instrumentation. Antibody detection tests detect IgG and IgM antibodies against the virus. Not useful for diagnosis as an antibody response is not detectable in the first week of illness. Test time is
8. Introduction to Viruses. Viruses are typically described as obligate intracellular parasites, acellular infectious agents that require the presence of a host cell in order to multiply. Viruses that have been found to infect all types of cells - humans, animals, plants, bacteria, yeast, archaea, protozoa…some scientists even claim they ...
virus, its genomic sequence would show a mix of known elements. This is not the case. Another coronavirus, SARS-CoV-1, the cause of the Severe Acute Respiratory Syndrome (SARS) outbreak in 2003, was also closely related to other coronaviruses isolated from bats. These close genetic relations of SARS-CoV-1, SARS-
The virus family concept is fundamentally important in understanding the biologic classification of viruses. 1 By specifying the family to which a virus belongs, much can be inferred about its physical, chemical, and biologic properties and its evolutionary relationships and modes of gene expression. 2 Virus families are designated with the suffix -viridae. 3 Families are distinguished largely ...
current infection (presence of virus) during the acute phase of disease. NAAT test time is between 13 minutes and 3 hours and require specific instrumentation. Antibody detection tests detect IgG and IgM antibodies against the virus. Not useful for diagnosis as an antibody response is not detectable in the first week of illness. Test time is
Computer Viruses: an Introduction - University of Wollongong
A virus is a tiny, infectious particle that can reproduce only by infecting a host cell. Viruses "commandeer" the host cell and use its resources to make more viruses, basically reprogramming it to become a virus factory. Because they can't reproduce by themselves (without a host), viruses are not considered living.Nor do viruses have cells: they're very small, much smaller than the cells of ...
The second section requires students to answer 10 more questions about viruses. FOUR IMAGES HOMEWORK ASSIGNMENT. For the homework assignment, students will find or sketch four images that show how a virus passes from person to person. ... If you choose to administer the lesson pages to your students via PDF, you will need to save a new file ...
Nevertheless, you might discover on closer inspection that the unified virus taxonomy is a rather ramshackle construction, with taxonomic assignment rules often being based on quite different and inconsistent criteria between virus groups. For example, the assignment of viruses to the order Herpesvirales is based on their morphology, and is ...
A virus is a piece of computer code that. attaches itself to a program or file so it can. spread from computer to computer. It infects. as it travels. Viruses can damage your. software, your ...
or copy data from computer to computer. viruses can be transmitted via computer. syste ms, an inte rnal network or the. internet. Once a computer system gets. infected with a virus, the data ...
technician, pharmacist (emergency assignment), and pharmacy technician (emergency assignment)) is authorized to prescribe oseltamivir or Paxlovid. ... Treatments for Respiratory Viruses in Ontario Pharmacies) dated December 12, 2023. 2 For Paxlovid, refer to Paxlovid - What Pharmacists and Prescribers Need to Know (with Appendix)
Assignment of virus and antimicrobial resistance genes to microbial hosts in a complex microbial community by combined long-read assembly and proximity ligation August 2019 Genome Biology 20(1)