100+ Great Chemistry Research Topics

Table of contents
- 1 5 Tips for Writing Chemistry Research Papers
- 2 Chemical Engineering Research Topics
- 3 Organic Сhemistry Research Topics
- 4 Іnorganic Сhemistry Research Topics
- 5 Biomolecular Сhemistry Research Topics
- 6 Analytical Chemistry Research Topics
- 7 Computational Chemistry Research Topics
- 8 Physical Chemistry Research Topics
- 9 Innovative Chemistry Research Topics
- 10 Environmental Chemistry Research Topics
- 11 Green Chemistry Research Topics
- 12.1 Conclusion
Do you need a topic for your chemistry research paper? Are you unsure of where to start? Don’t worry – we’re here to help. In this post, we’ll go over a series of the best chemistry research paper topics as well as Tips for Writing Chemistry Research Papers on different topics. By the time you finish reading this post, you’ll have plenty of ideas to get started on your next research project!
There are many different subfields of chemistry, so it can be tough to find interesting chemistry topics to write about. If you’re struggling to narrow down your topic, we’ll go over lists of topics in multiple fields of study.
Doing research is important to help scientists learn more about the world around us. By researching different compounds and elements, we can learn more about how they interact with one another and how they can be used to create new products or improve existing ones.
There are many different topics that you can choose to research in chemistry. Here are just a few examples:
- The history of chemistry and how it has evolved over time
- How different chemicals react with one another
- How to create new compounds or improve existing ones
- The role of chemistry in the environment
- The health effects of different chemicals

5 Tips for Writing Chemistry Research Papers
Once you have chosen a topic for your research paper , it is important to follow some tips to ensure that your paper is well-written and accurate. Here are a few tips to get you started:
- Start by doing some background research on your topic. This will help you understand the basics of the topic and give you a good foundation to build your paper on.
- Make sure to cite all of the sources that you use in your paper. This will help to show where you got your information and will also help to add credibility to your work.
- Be sure to proofread your paper before you submit it. This will ensure that there are no errors and that your paper is clear and concise.
- Get help from a tutor or friend if you are struggling with your paper. They may be able to offer helpful advice or feedback.
- Take your time when writing your research paper . This is not a race, and it is important to make sure that you do a good job on your research.
By following these tips, you can be sure that your chemistry research paper will be a success! So what are you waiting for? Let’s go over some of the best research paper topics out there.
Chemical Engineering Research Topics
Chemical Engineering is a branch of engineering that deals with the design and application of chemical processes. If you’re wondering how to choose a paper topic, here are some ideas to inspire you:
- How to create new alloy compounds or improve existing ones
- The health effects of the food industry chemicals
- Chemical engineering and sustainable development
- The future of chemical engineering
- Chemical engineering and the food industry
- Chemical engineering and the pharmaceutical industry
- Chemical engineering and the cosmetics industry
- Chemical engineering and the petrochemical industry
- Biocompatible materials for drug delivery systems
- Membrane technology in water treatment
- Development of synthetic fibers for industrial use
These are just a few examples – there are many more possibilities out there! So get started on your research today. Who knows what you might discover!
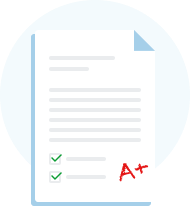
Organic Сhemistry Research Topics
Organic chemistry is the study of carbon-containing molecules. There are many different organic chemistry research topics that a student could choose to focus on and here are just a few examples of possible research projects in organic chemistry:
- Investigating new methods for synthesizing chiral molecules
- Studying the structure and reactivity of carbon nanotubes
- Investigating metal complexes with organometallic ligands
- Designing benzene derivatives with improved thermal stability
- Exploring new ways to control the stereochemistry of chemical reactions
- Studying the role of enzymes in organic synthesis
- Investigating new strategies for combating drug resistance
- Developing new methods for detecting explosives residues
- Studying the photochemistry of organic molecules
- Studying the behavior of organometallic compounds in biological systems
- Synthetic routes for biodegradable plastics
- Catalysis in organic synthesis
- Development of non-toxic solvents
Іnorganic Сhemistry Research Topics
Inorganic Chemistry is the study of the chemistry of materials that do not contain carbon. Unlike other chemistry research topics, these include elements such as metals, minerals, and inorganic compounds. If you are looking for inorganic chemistry research topics on inorganic chemistry, here are some ideas to get you started:
- How different metals react with one another
- How to create new alloys or improve existing ones
- The role of inorganic chemistry in the environment
- Rare earth elements and their applications in electronics
- Inorganic polymers in construction materials
- Photoluminescent materials for energy conversion
- Inorganic chemistry and sustainable development
- The future of inorganic chemistry
- Inorganic chemistry and the food industry
- Inorganic chemistry and the pharmaceutical industry
- Atomic structure progressive scale grading
- Inorganiс Сhemistry and the cosmetics industry
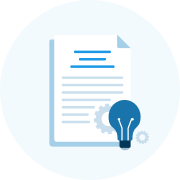
Biomolecular Сhemistry Research Topics
Biomolecular chemistry is the study of molecules that are important for life. These molecules can be found in all living things, from tiny bacteria to the largest animals. Researchers who work in this field use a variety of techniques to learn more about how these molecules function and how they interact with each other.
If you are looking for essential biomolecular chemistry research topics, here are some ideas to get you started:
- The structure and function of DNA
- Lipidomics and its applications in disease diagnostics
- The structure and function of proteins
- The role of carbohydrates in the body
- The role of lipids in the body
- How enzymes work
- Protein engineering for therapeutic applications
- The role of biochemistry in heart disease
- Cyanides and their effect on the body
- The role of biochemistry in cancer treatment
- The role of biochemistry in Parkison’s disease treatment
- The role of biochemistry in the immune system
- Carbohydrate-based vaccines
The possibilities are endless for someone willing to dedicate some time to research.
Analytical Chemistry Research Topics
Analytical Chemistry is a type of chemistry that helps scientists figure out what something is made of. This can be done through a variety of methods, such as spectroscopy or chromatography. If you are looking for research topics, here are some ideas to get you started:
- How food chemicals react with one another
- Mass spectrometry
- Microplastics detection in marine environments
- Development of sensors for heavy metal detection in water
- Analytical aspects of gas and liquid chromatography
- Analytical chemistry and sustainable development
- Atomic absorption spectroscopy methods and best practices
- Analytical chemistry and the pharmaceutical industry in Ibuprofen consumption
- Analytical chemistry and the cosmetics industry in UV protectors
- High-throughput screening methods in pharmaceutical analysis
- Dispersive X-ray analysis of damaged tissues
Analytical chemistry is considered by many a complex science and there is a lot yet to be discovered in the field.
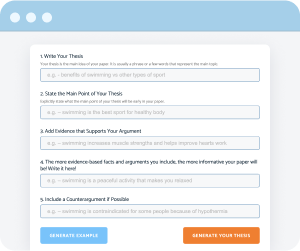
Computational Chemistry Research Topics
Computational chemistry is a way to use computers to help chemists understand chemical reactions. This can be done by simulating reactions or by designing new molecules. If you are looking for essential chemistry research topics in computational chemistry, here are some ideas to get you started:
- Molecular mechanics simulation
- Machine learning applications in predicting molecular properties
- Reaction rates of complex chemical reactions
- Designing new molecules: how can simulation help
- The role of computers in the study of quantum mechanics
- How to use computers to predict chemical reactions
- Using computers to understand organic chemistry
- The future of computational Chemistry in organic reactions
- The impacts of simulation on the development of new medications
- Combustion reaction simulation impact on engine development
- Quantum-chemistry simulation review
- Simulation of protein folding and misfolding in diseases
- Development of algorithms for chemical synthesis planning
- Applications of Metal-Organic Frameworks in water sequestration and catalysis
Computers are cutting-edge technology in chemical research and this relatively new field of study has a ton yet to be explored.
Physical Chemistry Research Topics
Physical chemistry is the study of how matter behaves. It looks at the physical and chemical properties of atoms and molecules and how they interact with each other. If you are looking for physical chemistry research topics, here are some ideas to get you started:
- Standardization of pH scales
- Structure of atom on a quantum scale
- Bonding across atoms and molecules
- The effect of temperature on chemical reactions
- The role of light in in-body chemical reactions
- Chemical kinetics
- Molecular dynamics in confined spaces
- Quantum computing for solving chemical problems
- Studies on non-Newtonian fluids in industrial processes
- Surface tension and its effects on mixtures
- The role of pressure in chemical reactions
- Rates of diffusion in gases and liquids
- The role of entropy in chemical reactions
Here are just a few samples, but there are plenty more options! Start your research right now!
Innovative Chemistry Research Topics
Innovative chemistry is all about coming up with new ideas and ways to do things. This can be anything from creating new materials to finding new ways to make existing products. If you are looking for ground-breaking chemistry research topics, here are some ideas to get you started:
- Amino acids side chain effects in protein folding
- Chemistry in the production of nanomaterials
- The role of enzymes in chemical reactions
- Photocatalysis in 3D printing
- Avoiding pesticides in agriculture
- Combining chemical and biological processes
- Gene modification in medicinal chemistry
- The role of quantum mechanics in chemical reactions
- Astrochemical research on extraterrestrial molecules
- Spectroscopy signatures of pressurized organic components
- Development of smart materials with responsive properties
- Chemistry in space: studying chemical reactions in microgravity
- Utilization of CO2 in chemical synthesis
- Use of black soldier fly carcasses for bioplastic production using extracted chitin
- Bioorthogonal chemistry for molecule synthesis inside living systems
If you need a hand, there are several sites that also offer research papers for sale and can be a great asset as you work to create your own research papers.
Whatever route you decide to take, good luck! And remember – the sky’s the limit when it comes to research! So get started today and see where your studies may take you. Who knows, you might just make a breakthrough discovery!
Environmental Chemistry Research Topics
Environmental Chemistry is the study of how chemicals interact with the environment. This can include anything from the air we breathe to the water we drink. If you are looking for environmental chemistry research topics, here are some ideas to get you started:
- Plastic effects on ocean life
- Urban ecology
- The role of carbon in climate change
- Air pollution and its effects
- Water pollution and its effects
- Chemicals in food and their effect on the body
- The effect of chemicals on plant life
- Earth temperature prediction models
- Effects of pharmaceuticals in aquatic environments
- Atmospheric chemistry and urban air quality
- Bioremediation techniques for oil spill cleanup
- Regulatory and environmental impact of Per- and Polyfluoroalkyl (PFA) substances
- Comparison of chemical regulation impacts like PFA with historical cases such as lead in fuel
A lot of research on the environment is being conducted at the moment because the environment is in danger. There are a lot of environmental problems that need to be solved, and research is the key to solving them.
Green Chemistry Research Topics
Green chemistry is the study of how to make products and processes that are environmentally friendly. This can include anything from finding new ways to recycle materials to developing new products that are biodegradable. If you are looking for green chemistry research topics, here are some ideas to get you started:
- Recycling and reuse of materials
- Developing biodegradable materials
- Improving existing recycling processes
- Green chemistry and sustainable development
- The future of green chemistry
- Green chemistry and the food industry
- Lifecycle assessment of chemical processes
- Green chemistry and the pharmaceutical industry
- Development of catalysts for green chemistry
- Green chemistry and the cosmetics industry
- Alternative energy sources for chemical synthesis
A more environmentally friendly world is something we all aspire for and a lot of research has been conducted on how we can achieve this, making this one of the most promising areas of study. The results have been varied, but there are a few key things we can do to make a difference.
Controversial Chemistry Research Topics
Controversial chemistry is all about hot-button topics that people are passionate about. This can include anything from the use of chemicals in warfare to the health effects of different chemicals. If you are looking for controversial topics to write about , here are some ideas to get you started:
- The use of chemicals in warfare
- Gene modification in human babies
- Bioengineering
- How fast food chemicals affect the human brain
- The role of the government in regulating chemicals
- Evolution of cigarette chemicals over time
- Chemical effects of CBD oils
- Ethical issues in genetic modification of organisms
- Nuclear energy: risks and benefits
- Use of chemicals in electronic waste recycling
- Antidepressant chemical reactions
- Synthetic molecule replication methods
- Gene analysis
Controversial research papers often appear in the media before it has been peer-reviewed and published in a scientific journal. The reason for this is that the media is interested in stories that are new, exciting, and generate a lot of debate.
Chemistry is an incredibly diverse and interesting field, with many controversial topics to write about. If you are looking for a research topic, consider the examples listed in this article. With a little bit of effort, you are sure to find a topic that is both interesting and within your skillset.
In order to be a good researcher, it is important to be able to think critically and solve problems. However, innovation in chemistry research can be challenging. When thinking about how to innovate, it is important to consider both the practical and theoretical aspects of your research. Additionally, try to build on the work of others in order to create something new and unique. With a little bit of effort, you are sure to be able to find a topic that is both interesting and within your skillset.
Happy writing!
Readers also enjoyed
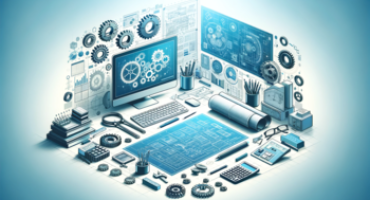
WHY WAIT? PLACE AN ORDER RIGHT NOW!
Just fill out the form, press the button, and have no worries!
We use cookies to give you the best experience possible. By continuing we’ll assume you board with our cookie policy.
- Privacy Policy

Home » 300+ Chemistry Research Topics
300+ Chemistry Research Topics
Table of Contents

Chemistry is a fascinating and complex field that explores the composition, properties, and behavior of matter at the molecular and atomic level. As a result, there are numerous chemistry research topics that can be explored, ranging from the development of new materials and drugs to the study of natural compounds and the environment. In this rapidly evolving field, researchers are constantly uncovering new insights and pushing the boundaries of our understanding of chemistry. Whether you are a student, a professional researcher, or simply curious about the world around you, there is always something new to discover in the field of chemistry. In this post, we will explore some of the exciting and important research topics in chemistry today.
Chemistry Research Topics
Chemistry Research Topics are as follows:
Organic Chemistry Research Topics
Organic Chemistry Research Topics are as follows:
- Development of novel synthetic routes for the production of biologically active natural products
- Investigation of reaction mechanisms and kinetics for organic transformations
- Design and synthesis of new catalysts for asymmetric organic reactions
- Synthesis and characterization of chiral compounds for pharmaceutical applications
- Development of sustainable methods for the synthesis of organic molecules using renewable resources
- Discovery of new reaction pathways for the conversion of biomass into high-value chemicals
- Study of molecular recognition and host-guest interactions for drug design
- Design and synthesis of new materials for energy storage and conversion
- Development of efficient and selective methods for C-H functionalization reactions
- Exploration of the reactivity of reactive intermediates such as radicals and carbenes
- Study of supramolecular chemistry and self-assembly of organic molecules
- Development of new methods for the synthesis of heterocyclic compounds
- Investigation of the biological activities and mechanisms of action of natural products
- Synthesis of polymeric materials with controlled architecture and functionality
- Development of new synthetic methodologies for the preparation of bioconjugates
- Investigation of the mechanisms of enzyme catalysis and the design of enzyme inhibitors
- Synthesis and characterization of novel fluorescent probes for biological imaging
- Development of new synthetic strategies for the preparation of carbohydrates and glycoconjugates
- Study of the properties and reactivity of carbon nanomaterials
- Design and synthesis of novel drugs for the treatment of diseases such as cancer, diabetes, and Alzheimer’s disease.
Inorganic Chemistry Research Topics
Inorganic Chemistry Research Topics are as follows:
- Synthesis and characterization of new metal-organic frameworks (MOFs) for gas storage and separation applications
- Development of new catalysts for sustainable chemical synthesis reactions
- Investigation of the electronic and magnetic properties of transition metal complexes for spintronics applications
- Synthesis and characterization of novel nanomaterials for energy storage applications
- Development of new ligands for metal coordination complexes with potential medical applications
- Investigation of the mechanism of metal-catalyzed reactions using advanced spectroscopic techniques
- Synthesis and characterization of new inorganic materials for photocatalytic water splitting
- Development of new materials for electrochemical carbon dioxide reduction reactions
- Investigation of the properties of transition metal oxides for energy storage and conversion applications
- Synthesis and characterization of new metal chalcogenides for optoelectronic applications
- Development of new methods for the preparation of inorganic nanoparticles with controlled size and shape
- Investigation of the reactivity and catalytic properties of metal clusters
- Synthesis and characterization of new metal-organic polyhedra (MOPs) for gas storage and separation applications
- Development of new methods for the synthesis of metal nanoparticles using environmentally friendly reducing agents
- Investigation of the properties of metal-organic frameworks for gas sensing applications
- Synthesis and characterization of new coordination polymers with potential magnetic and electronic properties
- Development of new materials for electrocatalytic water oxidation reactions
- Investigation of the properties of metal-organic frameworks for carbon capture and storage applications
- Synthesis and characterization of new metal-containing polymers with potential applications in electronics and energy storage
- Development of new methods for the synthesis of metal-organic frameworks using green solvents and renewable resources.
Physical Chemistry Research Topics
Physical Chemistry Research Topics are as follows:
- Investigation of the properties and interactions of ionic liquids in aqueous and non-aqueous solutions.
- Development of advanced analytical techniques for the study of protein structure and dynamics.
- Investigation of the thermodynamic properties of supercritical fluids for use in industrial applications.
- Development of novel nanomaterials for energy storage applications.
- Studies of the surface chemistry of catalysts for the optimization of their performance in chemical reactions.
- Development of new methods for the synthesis of complex organic molecules with improved yields and selectivity.
- Investigation of the molecular mechanisms involved in the catalysis of biochemical reactions.
- Development of new strategies for the controlled release of drugs and other bioactive molecules.
- Studies of the interaction of nanoparticles with biological systems for biomedical applications.
- Investigation of the thermodynamic properties of materials under extreme conditions of temperature and pressure.
- Development of new methods for the characterization of materials at the nanoscale.
- Investigation of the electronic and magnetic properties of materials for use in spintronics.
- Development of new materials for energy conversion and storage.
- Studies of the kinetics and thermodynamics of adsorption processes on surfaces.
- Investigation of the transport properties of ionic liquids for use in energy storage and conversion devices.
- Development of new materials for the capture and sequestration of greenhouse gases.
- Studies of the structure and properties of biomolecules for use in drug design and development.
- Investigation of the dynamics of chemical reactions in solution using time-resolved spectroscopic techniques.
- Development of new approaches for the synthesis of metallic and semiconductor nanoparticles with controlled size and shape.
- Studies of the structure and properties of materials for use in electrochemical energy storage devices.
Analytical Chemistry Research Topics
Analytical Chemistry Research Topics are as follows:
- Development and optimization of analytical techniques for the quantification of trace elements in food and environmental samples.
- Design and synthesis of novel analytical probes for the detection of biomolecules in complex matrices.
- Investigation of the fundamental mechanisms involved in the separation and detection of complex mixtures using chromatographic techniques.
- Development of sensors and biosensors for the detection of chemical and biological species in real-time.
- Investigation of the chemical and structural properties of nanomaterials and their applications in analytical chemistry.
- Development and validation of analytical methods for the quantification of contaminants and pollutants in water, air, and soil.
- Investigation of the molecular mechanisms underlying drug metabolism and toxicity using mass spectrometry.
- Development of analytical tools for the identification and quantification of drugs of abuse in biological matrices.
- Investigation of the chemical composition and properties of natural products and their applications in medicine and food science.
- Development of advanced analytical techniques for the characterization of proteins and peptides.
- Investigation of the chemistry and mechanism of action of antioxidants in foods and their impact on human health.
- Development of analytical methods for the detection and quantification of microorganisms in food and environmental samples.
- Investigation of the molecular mechanisms involved in the biosynthesis and degradation of important biomolecules such as proteins, carbohydrates, and lipids.
- Development of analytical methods for the detection and quantification of environmental toxins and their impact on human health.
- Investigation of the structure and properties of biological membranes and their role in drug delivery and disease.
- Development of analytical techniques for the characterization of complex mixtures such as petroleum and crude oil.
- Investigation of the chemistry and mechanism of action of natural and synthetic dyes.
- Development of analytical techniques for the detection and quantification of pharmaceuticals and personal care products in water and wastewater.
- Investigation of the chemical composition and properties of biopolymers and their applications in biomedicine and biomaterials.
- Development of analytical methods for the identification and quantification of essential nutrients and vitamins in food and dietary supplements.
Biochemistry Research Topics
Biochemistry Research Topics are as follows:
- The role of enzymes in metabolic pathways
- The biochemistry of DNA replication and repair
- Protein folding and misfolding diseases
- Lipid metabolism and the pathogenesis of atherosclerosis
- The role of vitamins and minerals in human metabolism
- Biochemistry of cancer and the development of targeted therapies
- The biochemistry of signal transduction pathways and their regulation
- The mechanisms of antibiotic resistance in bacteria
- The biochemistry of neurotransmitters and their roles in behavior and disease
- The role of oxidative stress in aging and age-related diseases
- The biochemistry of microbial fermentation and its applications in industry
- The biochemistry of the immune system and its response to pathogens
- The biochemistry of plant metabolism and its regulation
- The molecular basis of genetic diseases and gene therapy
- The biochemistry of membrane transport and its role in cell function
- The biochemistry of muscle contraction and its regulation
- The role of lipids in membrane structure and function
- The biochemistry of photosynthesis and its regulation
- The biochemistry of RNA splicing and alternative splicing events
- The biochemistry of epigenetics and its regulation in gene expression.
Environmental Chemistry Research Topics
Environmental Chemistry Research Topics are as follows:
- Investigating the effects of microplastics on aquatic ecosystems and their potential impact on human health.
- Examining the impact of climate change on soil quality and nutrient availability in agricultural systems.
- Developing methods to improve the removal of heavy metals from contaminated soils and waterways.
- Assessing the effectiveness of natural and synthetic antioxidants in mitigating the effects of air pollution on human health.
- Investigating the potential for using algae and other microorganisms to sequester carbon dioxide from the atmosphere.
- Studying the role of biodegradable plastics in reducing plastic waste and their impact on the environment.
- Examining the impact of pesticides and other agricultural chemicals on water quality and the health of aquatic organisms.
- Investigating the effects of ocean acidification on marine organisms and ecosystems.
- Developing new materials and technologies to reduce carbon emissions from industrial processes.
- Evaluating the effectiveness of phytoremediation in cleaning up contaminated soils and waterways.
- Studying the impact of microplastics on terrestrial ecosystems and their potential to enter the food chain.
- Developing sustainable methods for managing and recycling electronic waste.
- Investigating the role of natural processes, such as weathering and erosion, in regulating atmospheric carbon dioxide levels.
- Assessing the impact of urbanization on air quality and developing strategies to mitigate pollution in cities.
- Examining the effects of climate change on the distribution and abundance of species in different ecosystems.
- Investigating the impact of ocean currents on the distribution of pollutants and other environmental contaminants.
- Developing new materials and technologies for renewable energy generation and storage.
- Studying the effects of deforestation on soil quality, water availability, and biodiversity.
- Assessing the potential for using waste materials, such as agricultural residues and municipal solid waste, as sources of renewable energy.
- Investigating the role of natural and synthetic chemicals in regulating ecosystem functions, such as nutrient cycling and carbon sequestration.
Polymer Chemistry Research Topics
Polymer Chemistry Research Topics are as follows:
- Development of new monomers for high-performance polymers
- Synthesis and characterization of biodegradable polymers for sustainable packaging
- Design of stimuli-responsive polymers for drug delivery applications
- Investigation of the properties and applications of conductive polymers
- Development of new catalysts for controlled/living polymerization
- Synthesis of polymers with tailored mechanical properties
- Characterization of the structure-property relationship in polymer nanocomposites
- Study of the impact of polymer architecture on material properties
- Design and synthesis of new polymeric materials for energy storage
- Development of high-throughput methods for polymer synthesis and characterization
- Exploration of new strategies for polymer recycling and upcycling
- Synthesis and characterization of responsive polymer networks for smart textiles
- Design of advanced polymer coatings with self-healing properties
- Investigation of the impact of processing conditions on the morphology and properties of polymer materials
- Study of the interactions between polymers and biological systems
- Development of biocompatible polymers for tissue engineering applications
- Synthesis and characterization of block copolymers for advanced membrane applications
- Exploration of the potential of polymer-based sensors and actuators
- Design of novel polymer electrolytes for advanced batteries and fuel cells
- Study of the behavior of polymers under extreme conditions, such as high pressure or temperature.
Materials Chemistry Research Topics
Materials Chemistry Research Topics are as follows:
- Development of new advanced materials for energy storage and conversion
- Synthesis and characterization of nanomaterials for environmental remediation
- Design and fabrication of stimuli-responsive materials for drug delivery
- Investigation of electrocatalytic materials for fuel cells and electrolysis
- Fabrication of flexible and stretchable electronic materials for wearable devices
- Development of novel materials for high-performance electronic devices
- Exploration of organic-inorganic hybrid materials for optoelectronic applications
- Study of corrosion-resistant coatings for metallic materials
- Investigation of biomaterials for tissue engineering and regenerative medicine
- Synthesis and characterization of metal-organic frameworks for gas storage and separation
- Design and fabrication of new materials for water purification
- Investigation of carbon-based materials for supercapacitors and batteries
- Synthesis and characterization of self-healing materials for structural applications
- Development of new materials for catalysis and chemical reactions
- Exploration of magnetic materials for spintronic devices
- Investigation of thermoelectric materials for energy conversion
- Study of 2D materials for electronic and optoelectronic applications
- Development of sustainable and eco-friendly materials for packaging
- Fabrication of advanced materials for sensors and actuators
- Investigation of materials for high-temperature applications such as aerospace and nuclear industries.
Nuclear Chemistry Research Topics
Nuclear Chemistry Research Topics are as follows:
- Nuclear fission and fusion reactions
- Nuclear power plant safety and radiation protection
- Radioactive waste management and disposal
- Nuclear fuel cycle and waste reprocessing
- Nuclear energy and its impact on climate change
- Radiation therapy for cancer treatment
- Radiopharmaceuticals for medical imaging
- Nuclear medicine and its role in diagnostics
- Nuclear forensics and nuclear security
- Isotopic analysis in environmental monitoring and pollution control
- Nuclear magnetic resonance (NMR) spectroscopy
- Nuclear magnetic resonance imaging (MRI)
- Radiation damage in materials and radiation effects on electronic devices
- Nuclear data evaluation and validation
- Nuclear reactors design and optimization
- Nuclear fuel performance and irradiation behavior
- Nuclear energy systems integration and optimization
- Neutron and gamma-ray detection and measurement techniques
- Nuclear astrophysics and cosmology
- Nuclear weapons proliferation and disarmament.
Medicinal Chemistry Research Topics
Medicinal Chemistry Research Topics are as follows:
- Drug discovery and development
- Design and synthesis of novel drugs
- Medicinal chemistry of natural products
- Structure-activity relationships (SAR) of drugs
- Rational drug design using computational methods
- Target identification and validation
- Drug metabolism and pharmacokinetics (DMPK)
- Drug delivery systems
- Development of new antibiotics
- Design of drugs for the treatment of cancer
- Development of drugs for the treatment of neurological disorders
- Medicinal chemistry of peptides and proteins
- Development of drugs for the treatment of infectious diseases
- Discovery of new antiviral agents
- Design of drugs for the treatment of cardiovascular diseases
- Medicinal chemistry of enzyme inhibitors
- Development of drugs for the treatment of inflammatory diseases
- Design of drugs for the treatment of metabolic disorders
- Medicinal chemistry of anti-cancer agents
- Development of drugs for the treatment of rare diseases.
Food Chemistry Research Topics
Food Chemistry Research Topics are as follows:
- Investigating the effect of cooking methods on the nutritional value of food.
- Analyzing the role of antioxidants in preventing food spoilage and degradation.
- Examining the effect of food processing techniques on the nutritional value of fruits and vegetables.
- Studying the chemistry of food additives and their impact on human health.
- Evaluating the role of enzymes in food digestion and processing.
- Investigating the chemical properties and functional uses of food proteins.
- Analyzing the effect of food packaging materials on the quality and safety of food products.
- Examining the chemistry of food flavorings and the impact of flavor on consumer acceptance.
- Studying the role of carbohydrates in food texture and structure.
- Investigating the chemistry of food lipids and their impact on human health.
- Analyzing the chemical properties and functional uses of food gums and emulsifiers.
- Examining the effect of processing on the flavor and aroma of food products.
- Studying the chemistry of food preservatives and their impact on food safety.
- Investigating the chemical properties and functional uses of food fibers.
- Analyzing the effect of food processing on the bioavailability of nutrients.
- Examining the chemistry of food colorants and their impact on consumer acceptance.
- Studying the role of vitamins and minerals in food and their impact on human health.
- Investigating the chemical properties and functional uses of food hydrocolloids.
- Analyzing the effect of food processing on the allergenicity of food products.
- Examining the chemistry of food sweeteners and their impact on human health.
Industrial Chemistry Research Topics
Industrial Chemistry Research Topics are as follows:
- Development of catalysts for selective hydrogenation reactions in the petrochemical industry.
- Green chemistry approaches for the synthesis of biodegradable polymers from renewable sources.
- Optimization of solvent extraction processes for the separation of rare earth elements from ores.
- Development of novel materials for energy storage applications, such as lithium-ion batteries.
- Production of biofuels from non-food sources, such as algae or waste biomass.
- Application of computational chemistry to optimize the design of new catalysts and materials.
- Design and optimization of continuous flow processes for large-scale chemical production.
- Development of new synthetic routes for the production of pharmaceutical intermediates.
- Investigation of the environmental impact of industrial processes and development of sustainable alternatives.
- Development of innovative water treatment technologies for industrial wastewater.
- Synthesis of functionalized nanoparticles for use in drug delivery and other biomedical applications.
- Optimization of processes for the production of high-performance polymers, such as polyamides or polyesters.
- Design and optimization of process control strategies for efficient and safe chemical production.
- Development of new methods for the detection and removal of heavy metal ions from industrial effluents.
- Investigation of the behavior of surfactants in complex mixtures, such as crude oil or food products.
- Development of new materials for catalytic oxidation reactions, such as the removal of volatile organic compounds from air.
- Investigation of the properties and behavior of materials under extreme conditions, such as high pressure or high temperature.
- Development of new processes for the production of chemicals from renewable resources, such as bio-based building blocks.
- Study of the kinetics and mechanism of chemical reactions in complex systems, such as multi-phase reactors.
- Optimization of the production of fine chemicals, such as flavors and fragrances, using biocatalytic processes.
Computational Chemistry Research Topics
Computational Chemistry Research Topics are as follows:
- Development and application of machine learning algorithms for predicting chemical reactions and properties.
- Investigation of the role of solvents in chemical reactions using molecular dynamics simulations.
- Modeling and simulation of protein-ligand interactions to aid drug design.
- Study of the electronic structure and reactivity of catalysts for sustainable energy production.
- Analysis of the thermodynamics and kinetics of complex chemical reactions using quantum chemistry methods.
- Exploration of the mechanism and kinetics of enzyme-catalyzed reactions using molecular dynamics simulations.
- Investigation of the properties and behavior of nanoparticles using computational modeling.
- Development of computational tools for the prediction of chemical toxicity and environmental impact.
- Study of the electronic properties of graphene and other 2D materials for applications in electronics and energy storage.
- Investigation of the mechanisms of protein folding and aggregation using molecular dynamics simulations.
- Development and optimization of computational methods for calculating thermodynamic properties of liquids and solids.
- Study of the properties of supercritical fluids for applications in separation and extraction processes.
- Development of new methods for the calculation of electron transfer rates in complex systems.
- Investigation of the electronic and mechanical properties of carbon nanotubes for applications in nanoelectronics and nanocomposites.
- Development of new approaches for modeling the interaction of biomolecules with biological membranes.
- Study of the mechanisms of charge transfer in molecular and hybrid solar cells.
- Analysis of the structural and mechanical properties of materials under extreme conditions using molecular dynamics simulations.
- Development of new approaches for the calculation of free energy differences in complex systems.
- Investigation of the reaction mechanisms of metalloenzymes using quantum mechanics/molecular mechanics (QM/MM) methods.
- Study of the properties of ionic liquids for applications in catalysis and energy storage.
Theoretical Chemistry Research Topics
Theoretical Chemistry Research Topics are as follows:
- Quantum Chemical Studies of Excited State Processes in Organic Molecules
- Theoretical Investigation of Structure and Reactivity of Metal-Organic Frameworks
- Computational Modeling of Reaction Mechanisms and Kinetics in Enzyme Catalysis
- Theoretical Investigation of Non-Covalent Interactions in Supramolecular Chemistry
- Quantum Chemical Studies of Photochemical Processes in Organic Molecules
- Theoretical Analysis of Charge Transport in Organic and Inorganic Materials
- Computational Modeling of Protein Folding and Dynamics
- Quantum Chemical Investigations of Electron Transfer Processes in Complex Systems
- Theoretical Studies of Surface Chemistry and Catalysis
- Computational Design of Novel Materials for Energy Storage Applications
- Theoretical Analysis of Chemical Bonding and Molecular Orbital Theory
- Quantum Chemical Investigations of Magnetic Properties of Complex Systems
- Computational Modeling of Biological Membranes and Transport Processes
- Theoretical Studies of Nonlinear Optical Properties of Molecules and Materials
- Quantum Chemical Studies of Spectroscopic Properties of Molecules
- Theoretical Investigations of Reaction Mechanisms in Organometallic Chemistry
- Computational Modeling of Heterogeneous Catalysis
- Quantum Chemical Studies of Excited State Dynamics in Photosynthesis
- Theoretical Analysis of Chemical Reaction Networks
- Computational Design of Nanomaterials for Biomedical Applications
Astrochemistry Research Topics
Astrochemistry Research Topics are as follows:
- Investigating the chemical composition of protoplanetary disks and its implications for planet formation
- Examining the role of magnetic fields in the formation of complex organic molecules in space
- Studying the effects of interstellar radiation on the chemical evolution of molecular clouds
- Exploring the chemistry of comets and asteroids to better understand the early solar system
- Investigating the origin and evolution of interstellar dust and its relationship to organic molecules
- Examining the formation and destruction of interstellar molecules in shocked gas
- Studying the chemical processes that occur in the atmospheres of planets and moons in our solar system
- Exploring the possibility of life on other planets through astrobiology and astrochemistry
- Investigating the chemistry of planetary nebulae and their role in the evolution of stars
- Studying the chemical properties of exoplanets and their potential habitability
- Examining the chemical reactions that occur in the interstellar medium
- Investigating the chemical composition of supernova remnants and their impact on the evolution of galaxies
- Studying the chemical composition of interstellar grains and their role in the formation of stars and planets
- Exploring the chemistry of astrocytes and their role in the evolution of galaxies
- Investigating the formation of interstellar ice and its implications for the origin of life
- Examining the chemistry of molecular clouds and its relationship to star formation
- Studying the chemical composition of the interstellar medium in different galaxies and how it varies
- Investigating the role of cosmic rays in the formation of complex organic molecules in space
- Exploring the chemical properties of interstellar filaments and their relationship to star formation
- Studying the chemistry of protostars and the role of turbulence in the formation of stars.
Geochemistry Research Topics
Geochemistry Research Topics are as follows:
- Understanding the role of mineralogical and geochemical factors on metal mobility in contaminated soils
- Investigating the sources and fate of dissolved organic matter in aquatic systems
- Exploring the geochemical signatures of ancient sedimentary rocks to reconstruct Earth’s past atmospheric conditions
- Studying the impacts of land-use change on soil organic matter content and quality
- Investigating the impact of acid mine drainage on water quality and ecosystem health
- Examining the processes controlling the behavior and fate of emerging contaminants in the environment
- Characterizing the organic matter composition of shale gas formations to better understand hydrocarbon storage and migration
- Evaluating the potential for carbon capture and storage in geologic formations
- Investigating the geochemical processes controlling the formation and evolution of ore deposits
- Studying the geochemistry of geothermal systems to better understand energy production potential and environmental impacts
- Exploring the impacts of climate change on the biogeochemistry of terrestrial ecosystems
- Investigating the geochemical cycling of nutrients in coastal marine environments
- Characterizing the isotopic composition of minerals and fluids to understand Earth’s evolution
- Developing new analytical techniques to better understand the chemistry of natural waters
- Studying the impact of anthropogenic activities on the geochemistry of urban soils
- Investigating the role of microbial processes in geochemical cycling of elements in soils and sediments
- Examining the impact of wildfires on soil and water chemistry
- Characterizing the geochemistry of mineral dust and its impact on climate and biogeochemical cycles
- Investigating the geochemical factors controlling the release and transport of contaminants from mine tailings
- Exploring the biogeochemistry of wetlands and their role in carbon sequestration and nutrient cycling.
Electrochemistry Research Topics
Electrochemistry Research Topics are as follows:
- Development of high-performance electrocatalysts for efficient electrochemical conversion of CO2 to fuels and chemicals
- Investigation of electrode-electrolyte interfaces in lithium-ion batteries for enhanced battery performance and durability
- Design and synthesis of novel electrolytes for high-energy-density and stable lithium-sulfur batteries
- Development of advanced electrochemical sensors for the detection of trace-levels of analytes in biological and environmental samples
- Analysis of the electrochemical behavior of new materials and their electrocatalytic properties in fuel cells
- Study of the kinetics of electrochemical reactions and their effect on the efficiency and selectivity of electrochemical processes
- Development of novel strategies for the electrochemical synthesis of value-added chemicals from biomass and waste materials
- Analysis of the electrochemical properties of metal-organic frameworks (MOFs) for energy storage and conversion applications
- Investigation of the electrochemical degradation mechanisms of polymer electrolyte membranes in fuel cells
- Study of the electrochemical properties of 2D materials and their applications in energy storage and conversion devices
- Development of efficient electrochemical systems for desalination and water treatment applications
- Investigation of the electrochemical properties of metal-oxide nanoparticles for energy storage and conversion applications
- Analysis of the electrochemical behavior of redox-active organic molecules and their application in energy storage and conversion devices
- Study of the electrochemical behavior of metal-organic frameworks (MOFs) for the catalytic conversion of CO2 to value-added chemicals
- Development of novel electrode materials for electrochemical capacitors with high energy density and fast charge/discharge rates
- Investigation of the electrochemical properties of perovskite materials for energy storage and conversion applications
- Study of the electrochemical behavior of enzymes and their application in bioelectrochemical systems
- Development of advanced electrochemical techniques for the characterization of interfacial processes in electrochemical systems
- Analysis of the electrochemical behavior of nanocarbons and their application in electrochemical energy storage devices
- Investigation of the electrochemical properties of ionic liquids for energy storage and conversion applications.
Surface Chemistry Research Topics
Surface Chemistry Research Topics are as follows:
- Surface modification of nanoparticles for enhanced catalytic activity
- Investigating the effect of surface roughness on the wetting behavior of materials
- Development of new materials for solar cell applications through surface chemistry techniques
- Surface chemistry of graphene and its applications in electronic devices
- Surface functionalization of biomaterials for biomedical applications
- Characterization of surface defects and their effect on material properties
- Surface modification of carbon nanotubes for energy storage applications
- Developing surface coatings for corrosion protection of metals
- Synthesis of self-assembled monolayers on surfaces for sensor applications
- Surface chemistry of metal-organic frameworks for gas storage and separation
- Investigating the role of surface charge in protein adsorption
- Developing surfaces with superhydrophobic or superoleophobic properties for self-cleaning applications
- Surface functionalization of nanoparticles for drug delivery applications
- Surface chemistry of semiconductors and its effect on photovoltaic properties
- Development of surface-enhanced Raman scattering (SERS) substrates for trace analyte detection
- Surface functionalization of graphene oxide for water purification applications
- Investigating the role of surface tension in emulsion formation and stabilization
- Surface modification of membranes for water desalination and purification
- Synthesis and characterization of metal nanoparticles for catalytic applications
- Development of surfaces with controlled wettability for microfluidic applications.
Atmospheric Chemistry Research Topics
Atmospheric Chemistry Research Topics are as follows:
- The impact of wildfires on atmospheric chemistry
- The role of aerosols in atmospheric chemistry
- The chemistry and physics of ozone depletion in the stratosphere
- The chemistry and dynamics of the upper atmosphere
- The impact of anthropogenic emissions on atmospheric chemistry
- The role of clouds in atmospheric chemistry
- The chemistry of atmospheric particulate matter
- The impact of nitrogen oxides on atmospheric chemistry and air quality
- The effects of climate change on atmospheric chemistry
- The impact of atmospheric chemistry on climate change
- The chemistry and physics of atmospheric mercury cycling
- The impact of volcanic eruptions on atmospheric chemistry
- The chemistry and physics of acid rain formation and effects
- The role of halogen chemistry in the atmosphere
- The chemistry of atmospheric radicals and their impact on air quality and health
- The impact of urbanization on atmospheric chemistry
- The chemistry and physics of stratospheric polar vortex dynamics
- The role of natural sources (e.g. ocean, plants) in atmospheric chemistry
- The impact of atmospheric chemistry on the biosphere
- The chemistry and dynamics of the ozone hole over Antarctica.
Photochemistry Research Topics
Photochemistry Research Topics are as follows:
- Investigating the mechanisms of photoinduced electron transfer reactions in organic photovoltaic materials.
- Developing novel photoredox catalysts for photochemical reactions.
- Understanding the effects of light on DNA and RNA stability and replication.
- Studying the photochemistry of atmospheric pollutants and their impact on air quality.
- Designing new photoresponsive materials for advanced photonic and electronic devices.
- Exploring the photochemistry of metalloporphyrins for potential applications in catalysis.
- Investigating the photochemistry of transition metal complexes and their use as photodynamic therapy agents.
- Developing new photocatalytic systems for sustainable energy production.
- Studying the photochemistry of natural products and their potential pharmaceutical applications.
- Investigating the role of light in the formation and degradation of environmental contaminants.
- Designing new photochromic materials for smart windows and displays.
- Exploring the photochemistry of carbon nanomaterials for energy storage and conversion.
- Developing new light-driven molecular machines for nanotechnology applications.
- Investigating the photochemistry of organic dyes for potential applications in dye-sensitized solar cells.
- Studying the effects of light on the behavior of biological macromolecules.
- Designing new photoresponsive hydrogels for drug delivery applications.
- Exploring the photochemistry of semiconductor nanoparticles for potential applications in quantum computing.
- Investigating the mechanisms of photochemical reactions in ionic liquids.
- Developing new photonic sensors for chemical and biological detection.
- Studying the photochemistry of transition metal complexes for potential applications in water splitting and hydrogen production.
About the author
Muhammad Hassan
Researcher, Academic Writer, Web developer
You may also like

200+ Funny Research Topics

500+ Sports Research Topics

300+ American History Research Paper Topics

500+ Cyber Security Research Topics

500+ Environmental Research Topics

500+ Economics Research Topics
This website uses cookies to improve your user experience. By continuing to use the site, you are accepting our use of cookies. Read the ACS privacy policy.
- ACS Publications
28 Must-Read Topics in Chemistry
- Mar 4, 2021
- 14 min read
ACS Publications regularly produces collections of the most important chemistry research topics. These Virtual Collections of the most important chemistry research topics bring together the most important ideas in the field in a variety of ways, including Special Issues and ACS Selects from across the portfolio journals. These collections reflect the most important chemistry research […]
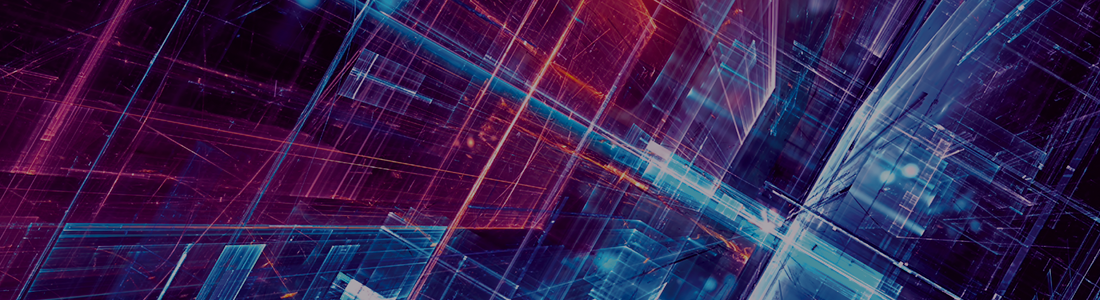
ACS Publications regularly produces collections of the most important chemistry research topics. These Virtual Collections of the most important chemistry research topics bring together the most important ideas in the field in a variety of ways, including Special Issues and ACS Selects from across the portfolio journals. These collections reflect the most important chemistry research topics of current scientific interest and are designed for experienced investigators and educators alike.
Browse 28 of the most important, engaging topics in chemists with Virtual Collections released by ACS Publications journals in Q4 2020:
Crystalline molecular materials: from structure to function.
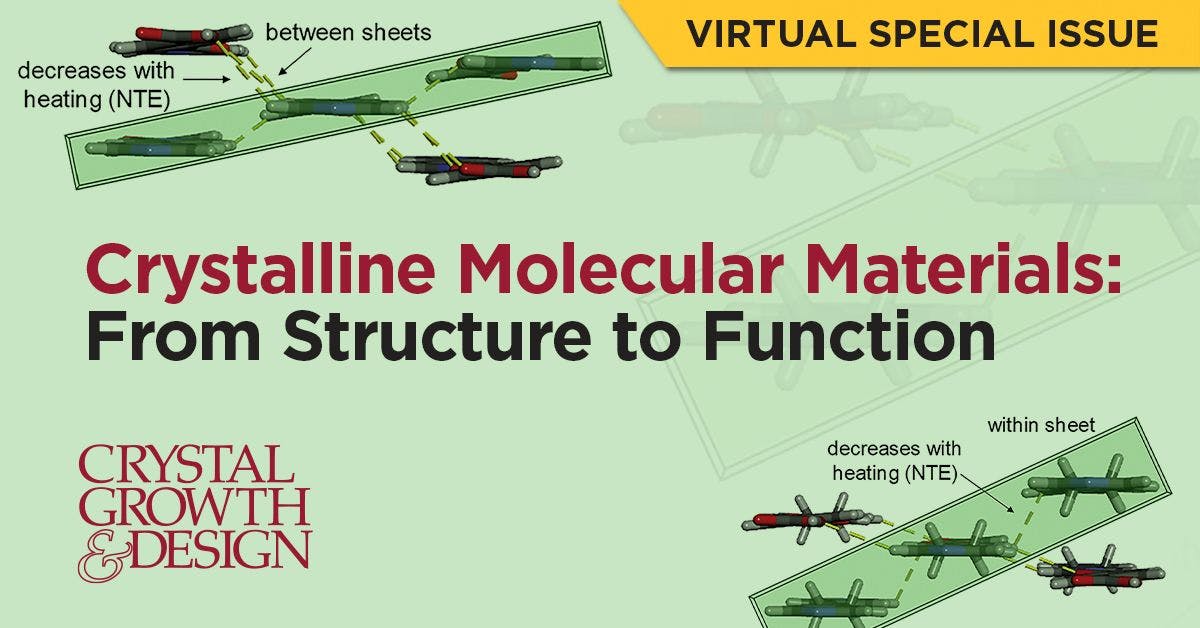
This Virtual Special Issue focuses on the design and study of materials wherein the target properties arise from, or are enhanced by, the three-dimensional assembly of molecules in a solid phase. The “structure−function” relationship transcends the nature of the individual molecule, and supramolecular organization is a key component in the material’s properties. The goal of this issue is to assemble contributions from a broad community of scientists with similar research interests, as defined by the need to understand and manipulate the bulk assembly of molecules. Placing emphasis on a common interest in supramolecular architecture, this issue showcases work in apparently disparate fields, including molecule-based magnetism, rare zero thermal expansion properties, and catalytic activity.
Read the Issue. ***
Materials for Thermoelectric Energy Conversion
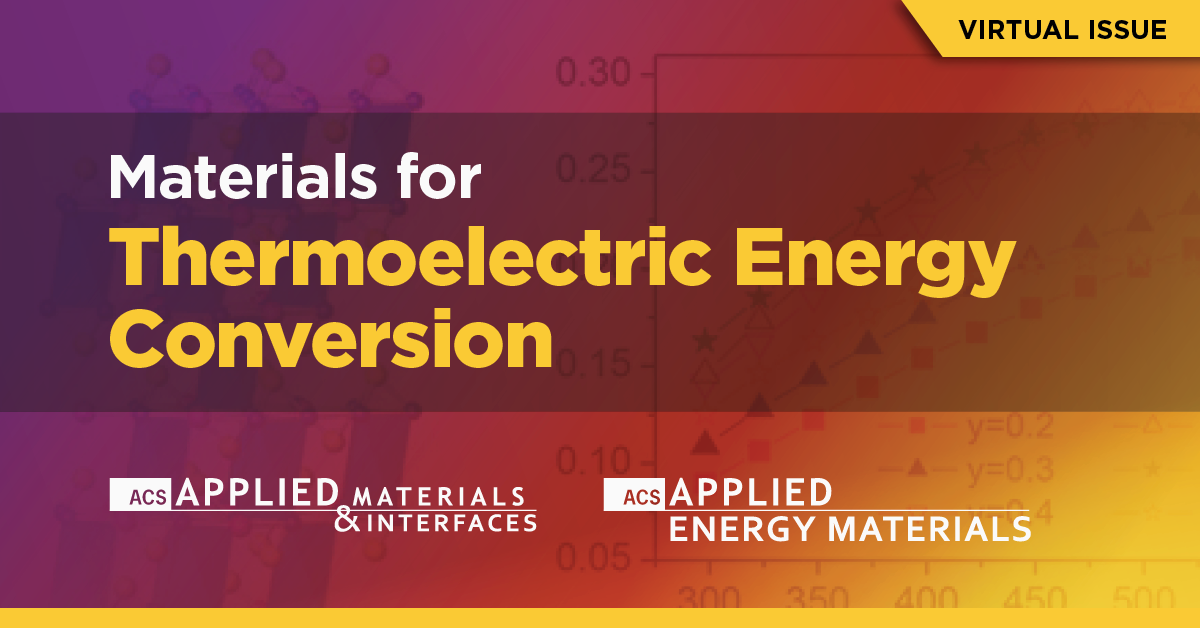
This virtual issue of ACS Applied Materials & Interfaces and ACS Applied Energy Materials presents cutting edge articles in the field of Thermoelectric Energy Conversion. Thermoelectric materials and devices are central for energy conversion and management as they convert waste heat into electricity. Given the ubiquitous nature of heat, thermoelectric materials provide total-package solutions to mitigate environmental crisis and energy needs. The realization of this has caused a surge in the development of high-performance, environmentally benign, robust, and earth-abundant inorganic materials, which can be used in heat to electrical energy generations in power plants, space, automobiles, households, battery technology, and data centers. Interestingly, flexible thermoelectric materials, mainly based on organic/polymer materials, have successfully been integrated into body-worn fabrics and watches, which simply utilize body heat to generate electricity. Furthermore, using the Peltier effect, thermoelectric coolers are developed and are one of the mainstays in the consumer market for refrigeration purposes, especially for portable applications. Hence, thermoelectricity is foreseen as a potential frontrunner in energy management for the near future.
Interfacialscience Developments at the Chinese Academy of Sciences
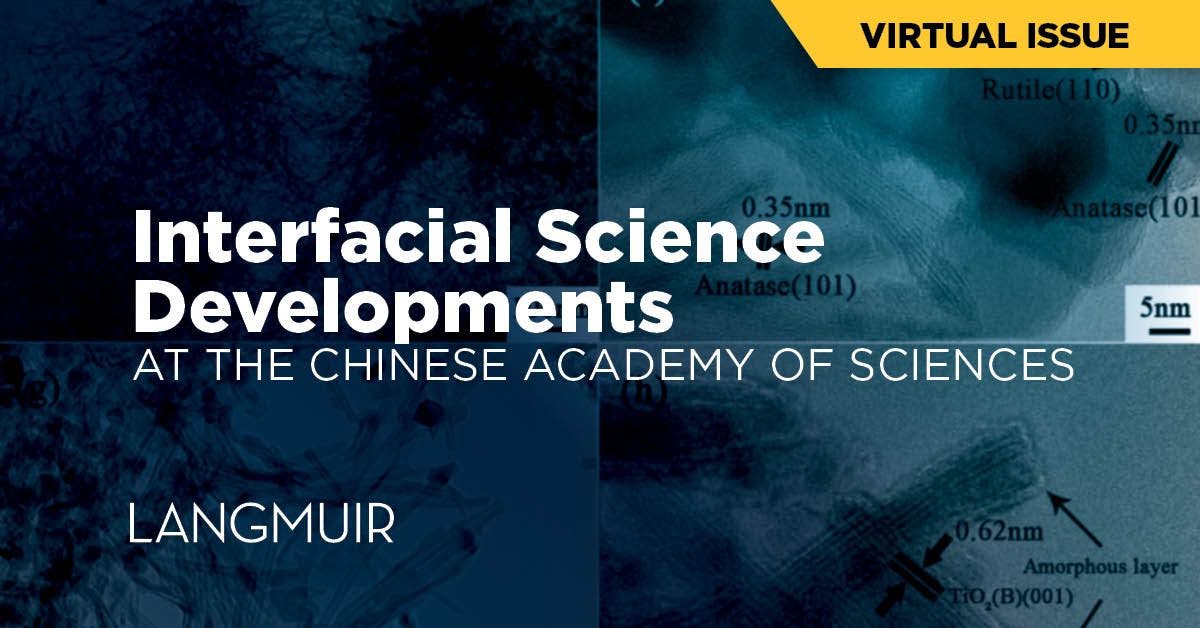
This virtual issue is a sampling of some of the most recent work from the Chinese Academy of Sciences, with an emphasis on work from this year (2020) so far. The 46 articles in this virtual issue cover a broad range of research topics, examples of which include Janus particle engineering and interfacial assembly, surface modification of colloid particles, stability of water monolayer in mineral under high pressure, nano-bubbles adsorption on surface, switching of underwater superhydrophilicity and superoleophobicity, nanostructured de-icing surface, lithium ion battery anode binder, bio-inspired smart liquid directional transport control, corrosion resistance of alloys, behavior of polymers at solid/liquid interface, and effect of polymer conformation on protein resistance.
Celebrating 90% Completion of the Human Proteome
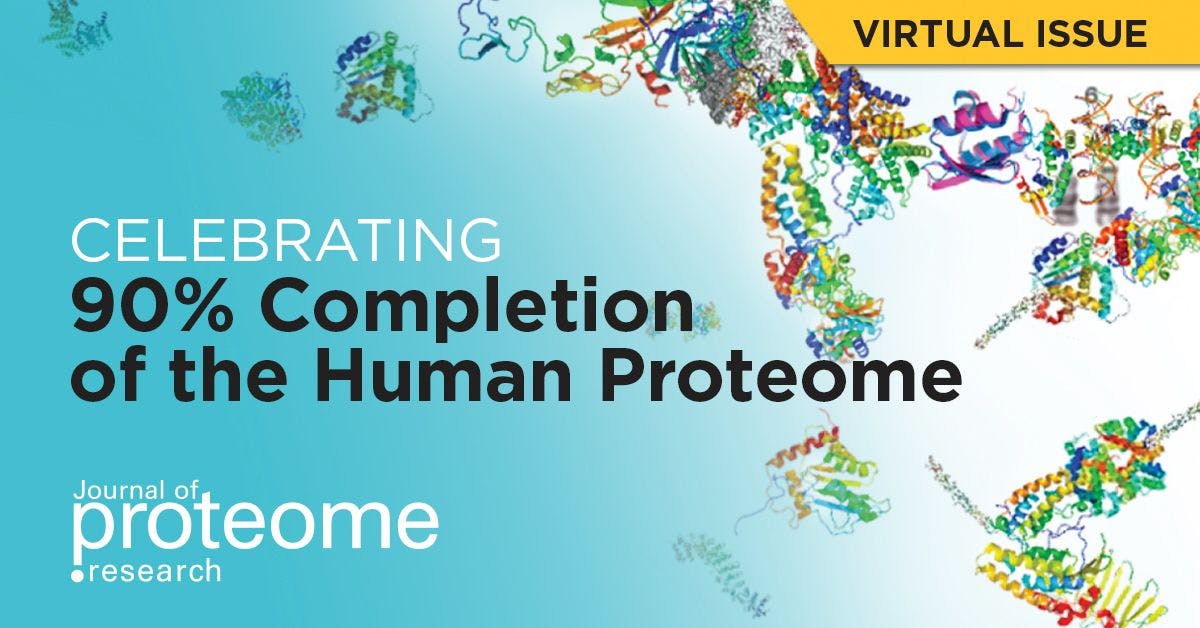
Twenty years after the establishment of the international Human Proteome Organization (HUPO) and ten years after its launch of the Human Proteome Project (HPP), researchers have much to celebrate. Today, HUPO will release the draft human proteome at the 19th Human Proteome Organization World Congress, connecting virtually, with this Virtual Issue published in the Journal of Proteome Research.
Read the Issue . ***
Nanomaterials-based Membranes for Chemical Separations
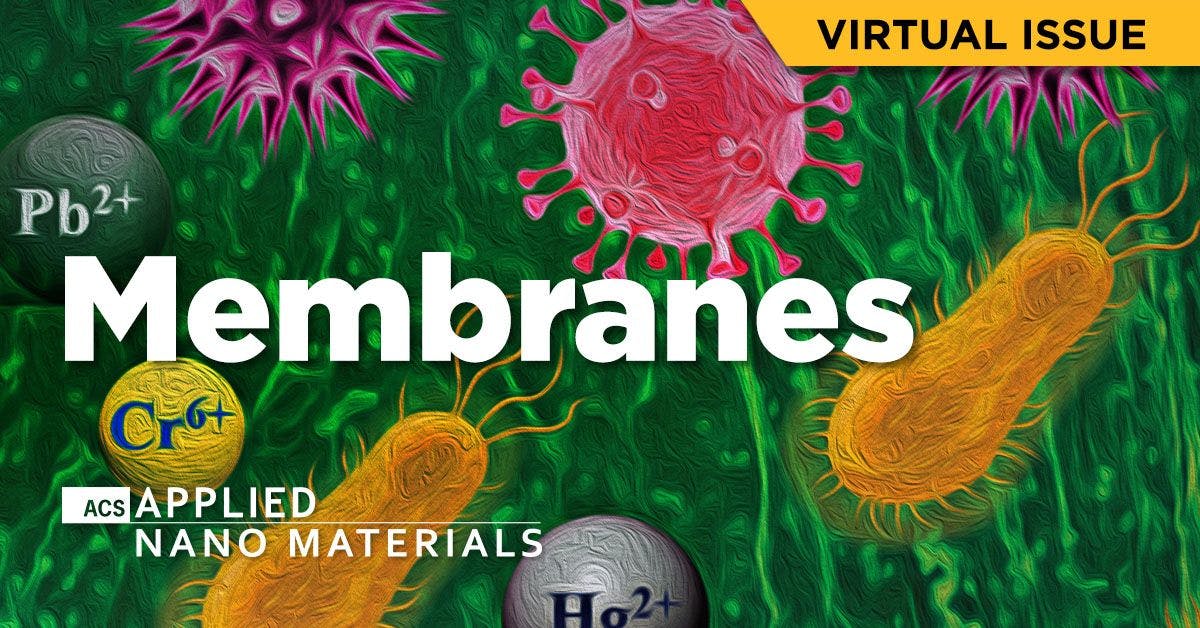
Membranes are a critical area of research in academia and have been used in industrial applications for decades. Membrane-based separations are desired in industry because they can be highly energy efficient and up to an order of magnitude less expensive than other techniques such as distillation. In addition, these separations are easily scaled to industrial levels so that advances in the laboratory can be translated to real applications. The key challenges in this field are how to separate chemicals with similar sizes by having a high flux for only one chemical through a membrane. This difference in flux should translate into a high selectivity for one chemical over one or more other chemicals present in a mixture. An unfortunate trade-off in membrane-based separations is that as the permeation of a chemical increases, the selectivity of the membrane will often decrease. To address these challenges, scientists often use cross-linked polymers with ill-defined pores, hard materials such as zeolites with well-defined pores, 2D materials, coated nanofibers, carbon nanotubes, metal nanoparticles, or other nanomaterials.
Organic Chemistry in China: Synthetic Methodology, Natural Products, and More
During the past 20 years, China has become a powerhouse in chemistry research, now leading globally in submissions of research articles to chemical journals. In recognizing these developments, Organic Letters presents a Virtual Issue that includes a collection of 25 research articles contributed by Chinese chemists during 2019-2020, selected from among the more than 1,000 articles published in the journal from China over this period.
Advances in Microfluidics Research
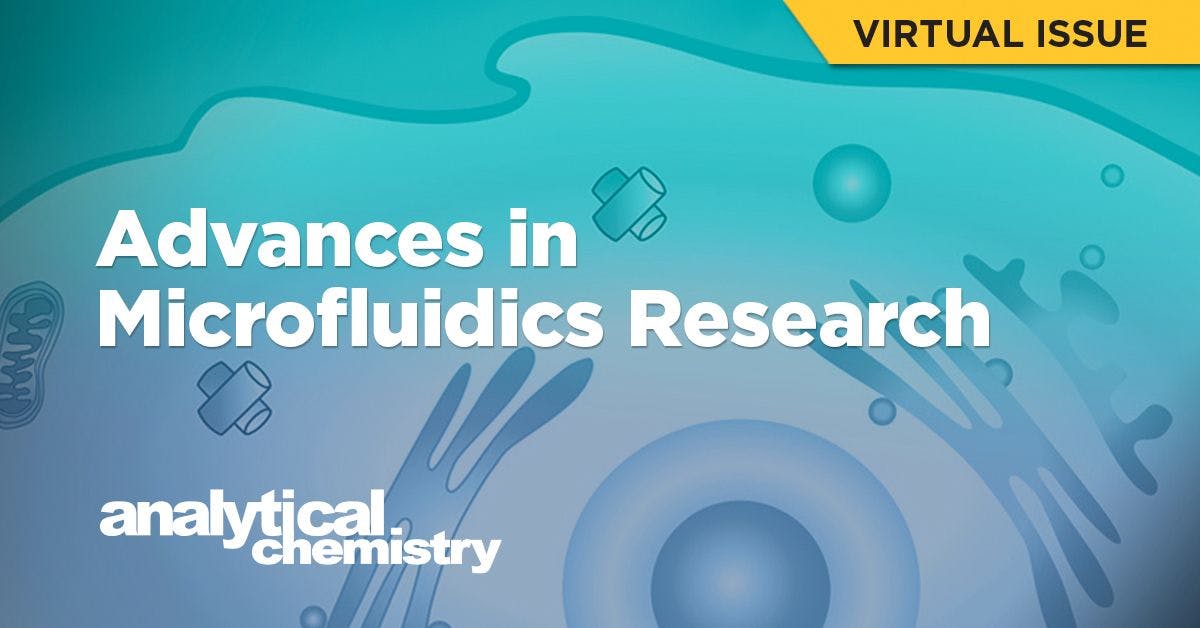
This Virtual Issue highlights articles published in Analytical Chemistry that showcase advances in microfluidics research over the past several years. The articles below are separated by sub-field and span research on virus detection to cell manipulation to 3D-printing, and are all at the cutting edge of microfluidics technologies. The thirty articles included in this collection were selected by Associate Editor Yoshinobu Baba and include previous winners of the Chemical & Biological Microsystems Society (CBMS)/ Analytical Chemistry co-sponsored Young Innovator Award.
Chemistry in Korea: IBS and Beyond
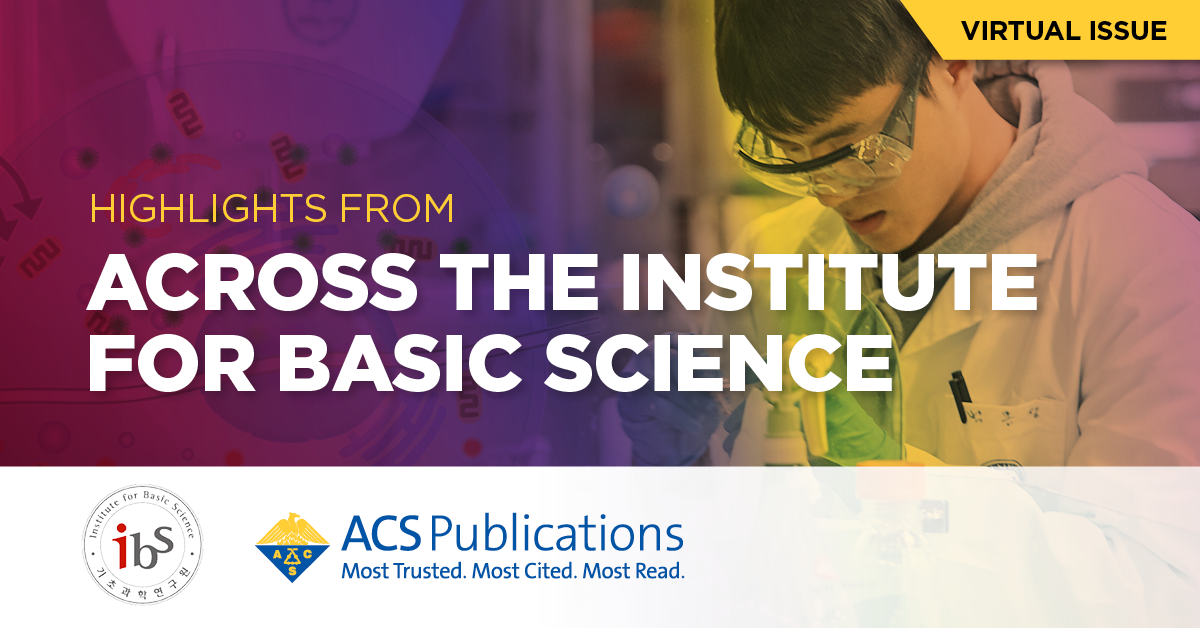
This virtual issue of “Chemistry in Korea: IBS and Beyond” highlights the latest contributions from eight IBS centers along with exciting advances from other emerging scientists in South Korea. Topics encompass a wide range of chemistry and its cross-boundary researches from theory and simulations, nanomaterials, molecular synthesis, catalysts, spectroscopy, supramolecular chemistry, soft materials to nanomedicine.
Highlighting Analytical Chemistry 2020 Advisory Board Members
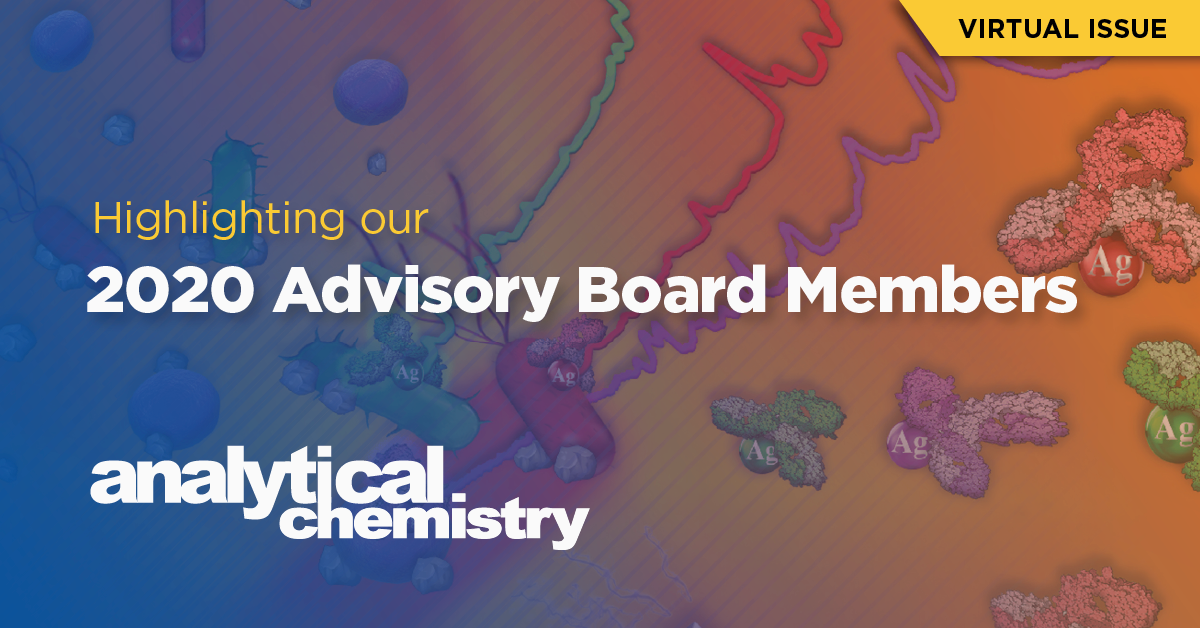
The members of Analytical Chemistry ‘s Editorial Advisory Board (EAB) and Early Career Board (ECB) panels devote substantial voluntary time and energy to support Analytical Chemistry and deserve special recognition for their contributions. In recognition of their service, this new virtual issue is dedicated to the members of both the journal’s EAB and ECB, with each selecting one of their recent Analytical Chemistry articles to highlight.
A Bright New World of Ferroelectrics: Magic of Spontaneous Polarization
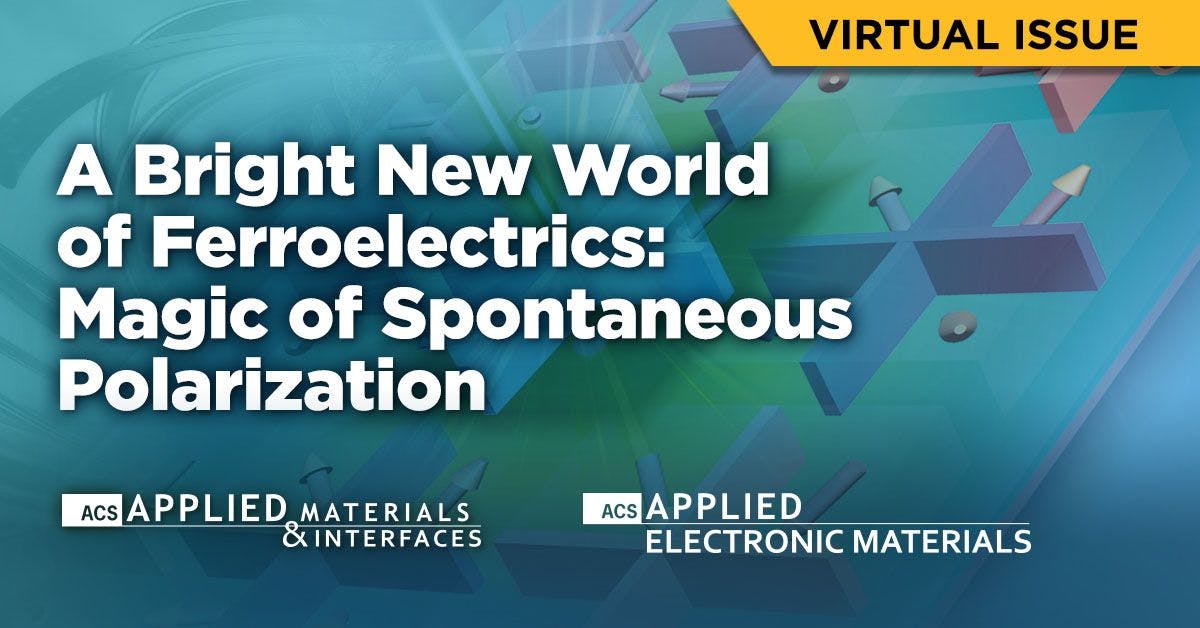
Ferroelectric materials featured with spontaneous polarization have experienced a century of vigorous development. The permanent electric dipole moment makes ferroelectric an outstanding multifunctional material for a wide range of applications. Ferroelectrics with unique coupling effects among electric, optical, mechanical, thermal, and magnetic orders, have been developed for a wide range of functional devices and triggered a new world-wide wave of ferroelectric research. This virtual issue highlights some of the key state-of-the-art findings in ferroelectrics published in ACS Applied Materials & Interfaces and ACS Applied Electronic Materials , and the editorial attempts to reflect the rapid development and provide a perspective in this field.
Peter J. Rossky Festschrift
This Virtual Special Issue honors Professor Peter J. Rossky and his contributions to the field of physical chemistry.
Computational and Experimental Advances in Biomembranes
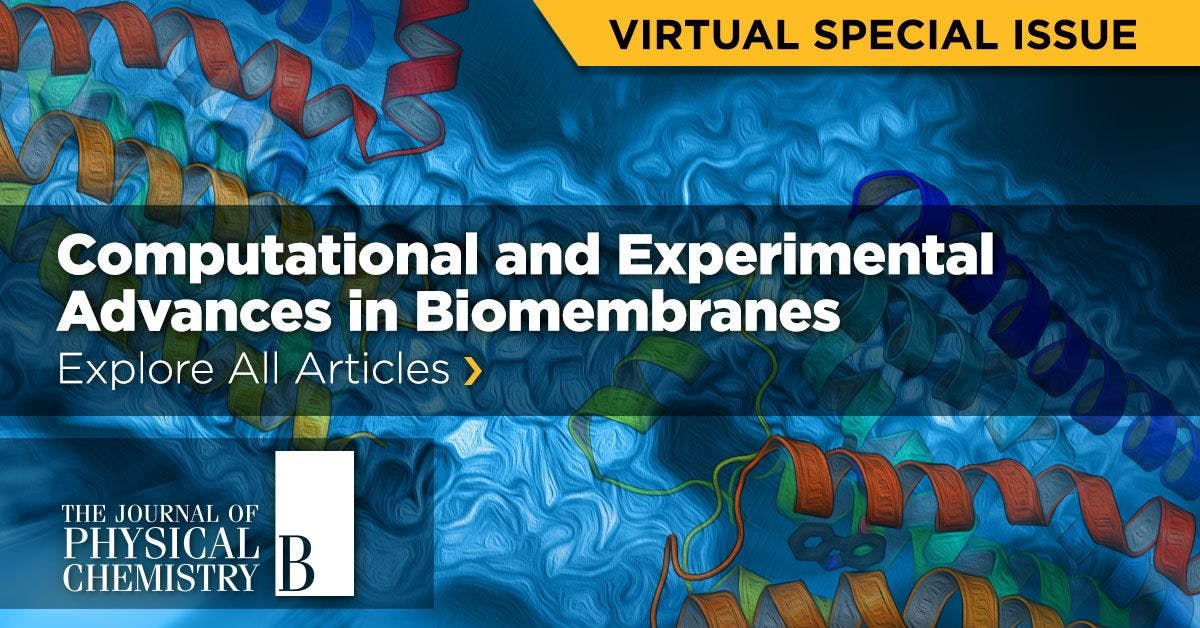
As an integral component of cellular architecture and signalling, cell membranes are central to cell physiology. Comprising a vastly heterogeneous mixture of proteins and lipids, cell membranes are constantly adapting their structural organization to regulate cellular processes. Malfunction at the level of lipid-protein interaction is implicated in numerous diseases, and hence, understanding cell membrane organization at the molecular level is of critical importance. The collection of articles in this Virtual Special Issue from The Journal of Physical Chemistry B provides a survey of the advances in both computational and experimental characterization of the complex processes underlying the behavior of cellular membranes.
Sensors and Industry
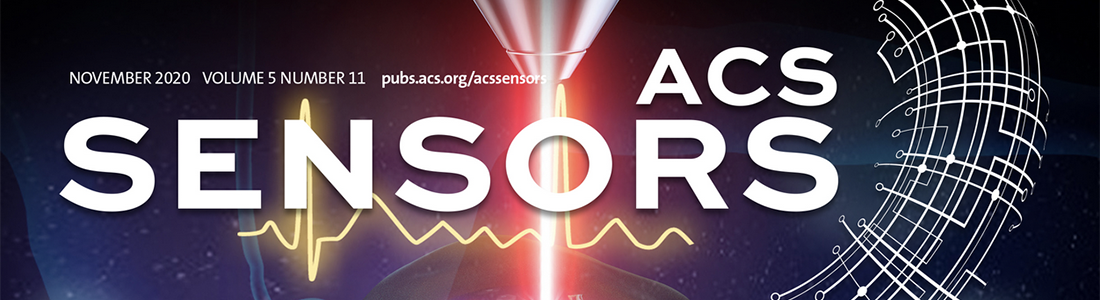
In this virtual issue, ACS Sensors and Analytical Chemistry highlight 30 of these outstanding industrial co-authored papers recently published in the two journals. The breadth of the articles in this collection emphasizes the incredible research on diagnostic methods being performed in both universities and industries, and highlights the benefits of collaboration between the two. Read the Issue . ***
Machine Learning in Physical Chemistry
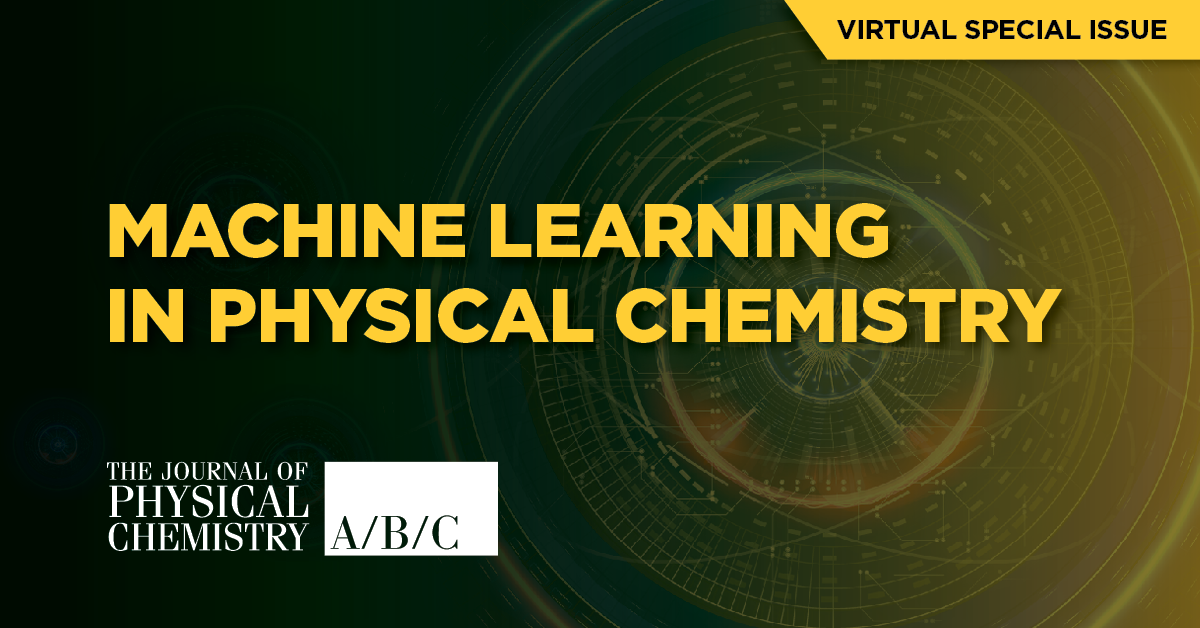
Physical chemistry stands today at an exciting transition state where the integration of machine learning and data science tools into all corners of the field is poised to do nothing short of revolutionizing the discipline. These powerful techniques – when appropriately combined with domain knowledge, tools, and expertise – have led to new physical insights, better understanding, accelerated discovery, rational design, and inverse engineering that transcend traditional approaches to materials, molecular, and chemical science and engineering. This collection of nearly 150 manuscripts from The Journal of Physical Chemistry A / B / C and The Journal of Physical Chemistry Letters reflects the relevance and popularity of this topic in physical chemistry by both the depth and breadth of excellent articles in this exciting collection.
Self-Healing Materials
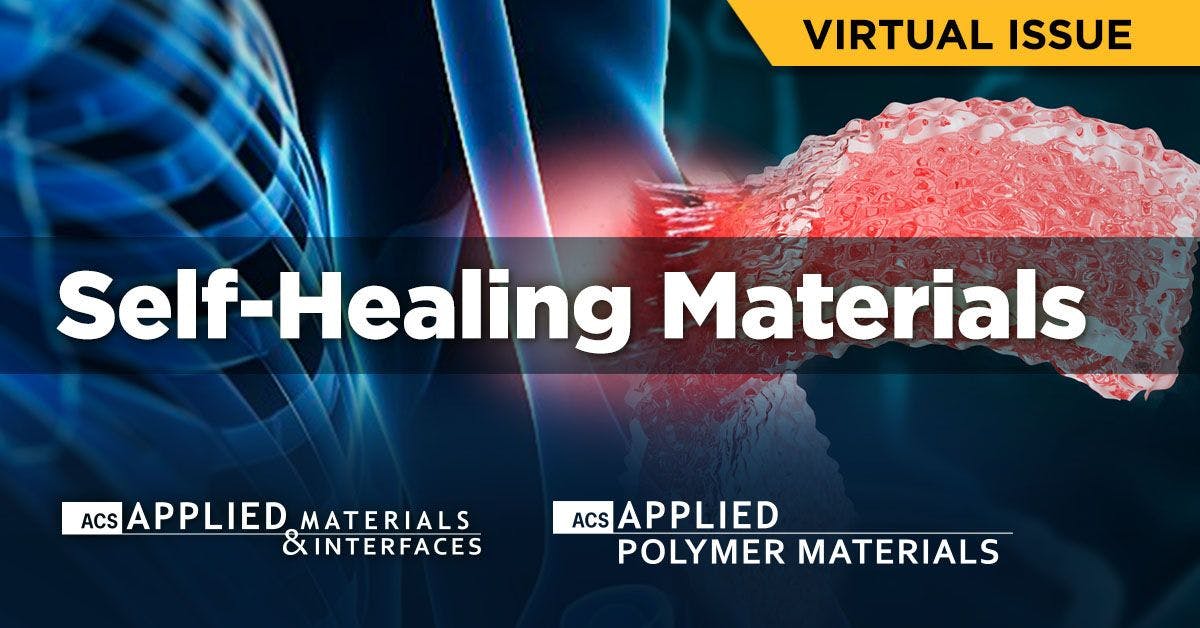
This is a spotlight on applications discusses developments made over the last six years that have enabled the fabrication of increasingly high-performance spray-coated perovskite solar cells. In particular, the various approaches adopted to spray-cast perovskite films (one-step vs two-step processes) ware charted and the development of sophisticated techniques used to control thin-film crystallinity is described. Finally, remaining research challenges are discussed that, once solved, may allow the mass deployment of low-cost solar energy.
Women in Mass Spectrometry
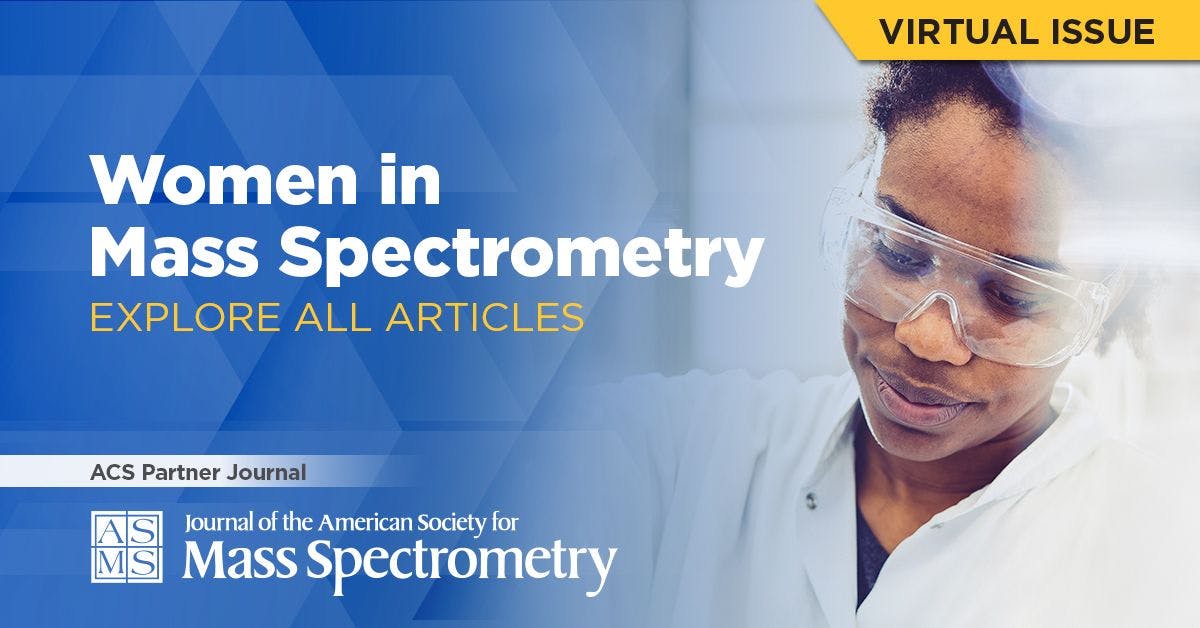
This virtual issue was assembled to feature talented women mass spectrometrists who publish in JASMS as the corresponding author. The articles compiled are among the most highly cited that were published in the journal in the last 5 years, regardless of gender, and are representative of the best mass spectrometry science reported in JASMS .
In Memory of Mario Molina (1943-2020)
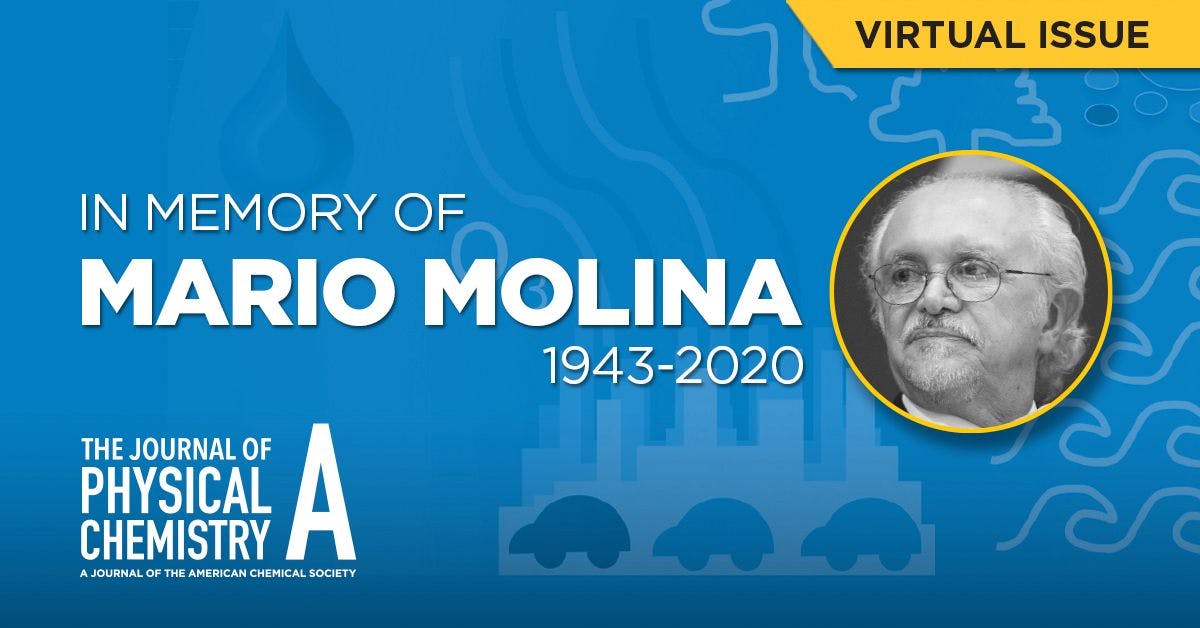
Mario Molina was a Mexican chemist who shared the 1995 Nobel Prize in chemistry with the late F. Sherwood Rowland of UC Irvine and Paul Crutzen of the Max Planck Institute for Chemistry in Mainz “for their work in atmospheric chemistry particularly concerning the formation and decomposition of ozone.” Molina passed away in his birth city of Mexico City at age 77 on 7 October 2020. A physical chemist at heart, Molina published about 80 papers in The Journal of Physical Chemistry . His mentees remember him by celebrating 30 of them. His indelible legacy lives on through his publications, his collaborators, the scholars that he trained, the innovations in experimental design he made, the thousands who were inspired and informed by his science communication, and the millions whose quality of life improved thanks to his work on stratospheric ozone depletion and air quality in megacities.
Women Scientists at the Forefront of Energy Research: A Virtual Issue, Part 3
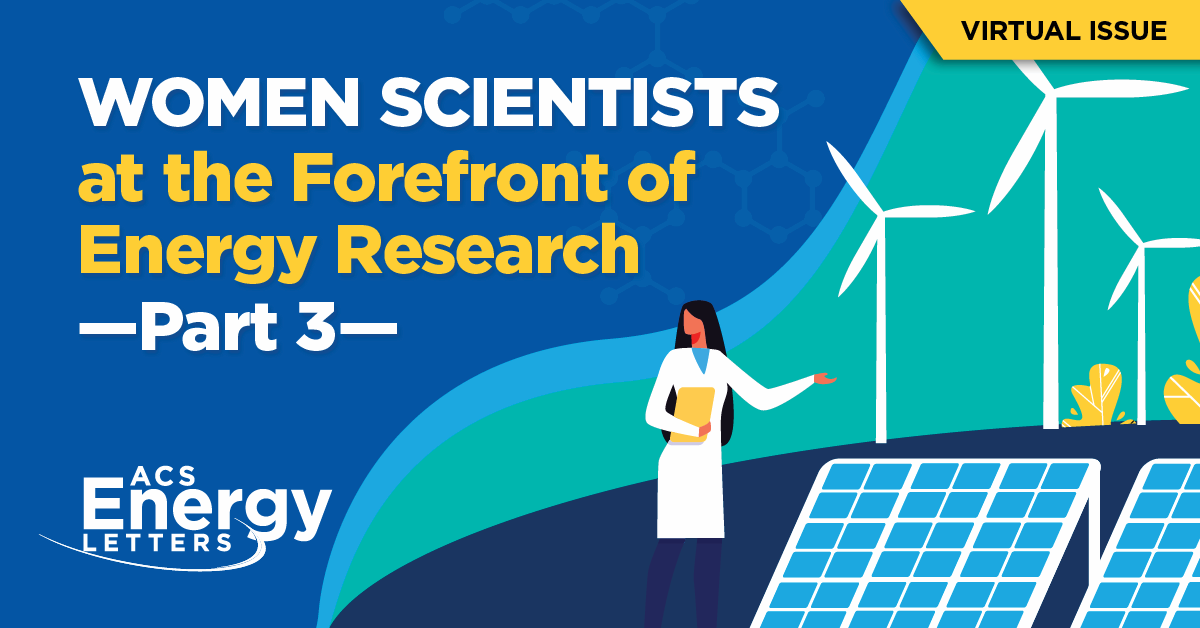
This is the third part of a series that recognizes women energy researchers who have published new advances from their laboratories in ACS Energy Letters . The inspirational stories and advice to newcomers in the field contained in this issue should provide motivation to advance the scientific research in energy conversion and storage. Through their personal reflections, these researchers discuss the successful career paths they have taken to become leaders in the scientific community.
Scalable Organic Chemistry: A Virtual Issue to highlight Organic Process Research & Development
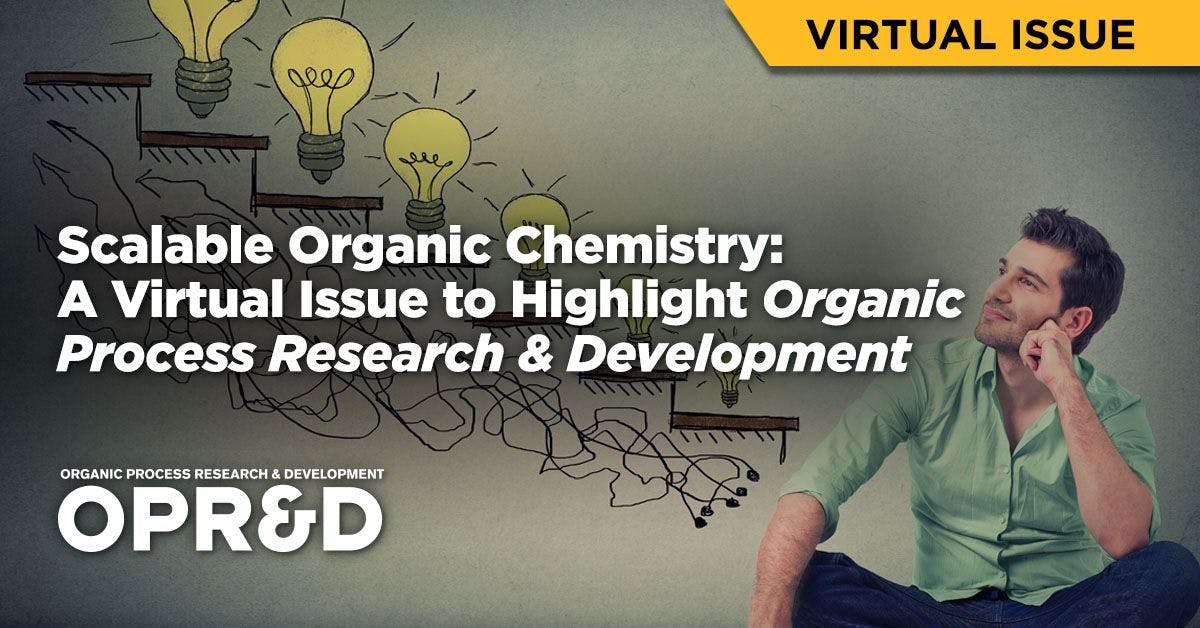
From small-scale use in academic research to large-scale application in industrial processes, only select chemistries make the cut to be relevant throughout the scale-up process. This virtual issue showcases a collection of innovative and industrially-relevant papers on key topics from academic and industrial chemists in Organic Process Research & Development .
Virtual Issue in Memoriam of Dr. Alan Poland (1940-2020)
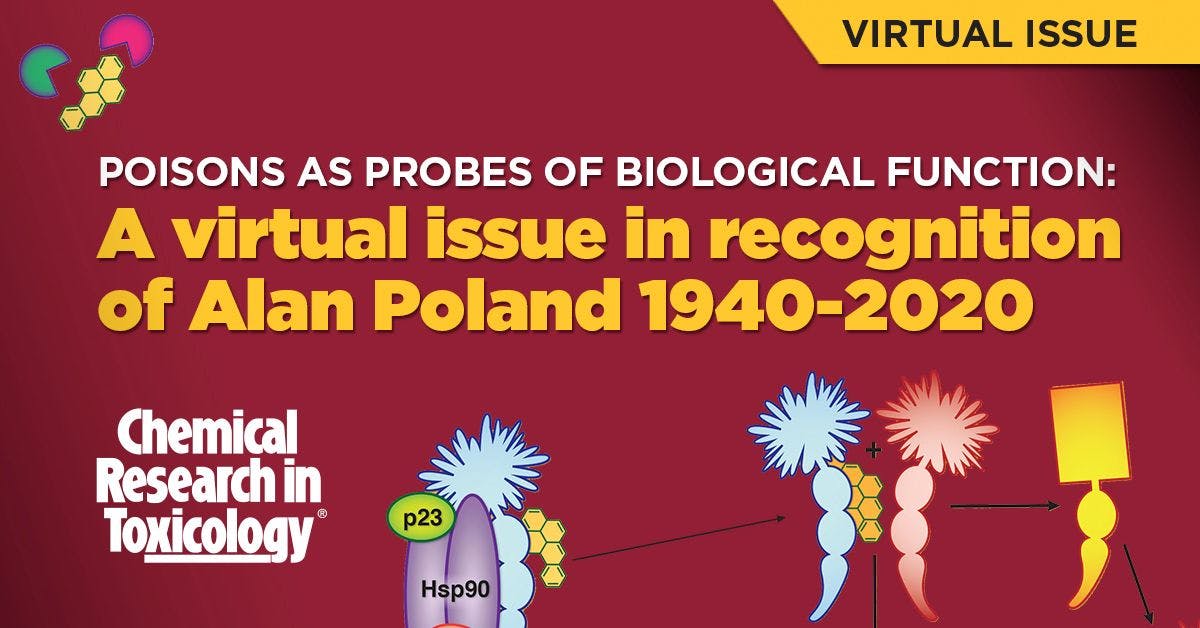
Dr. Alan Poland was a major influence on the development of modern molecular toxicology and the understanding of how chemicals cause cancer. He is most widely known for his groundbreaking work to explain the adverse effects of dioxins, chemicals and related environmental pollutants.
Deep Eutectic Solvents
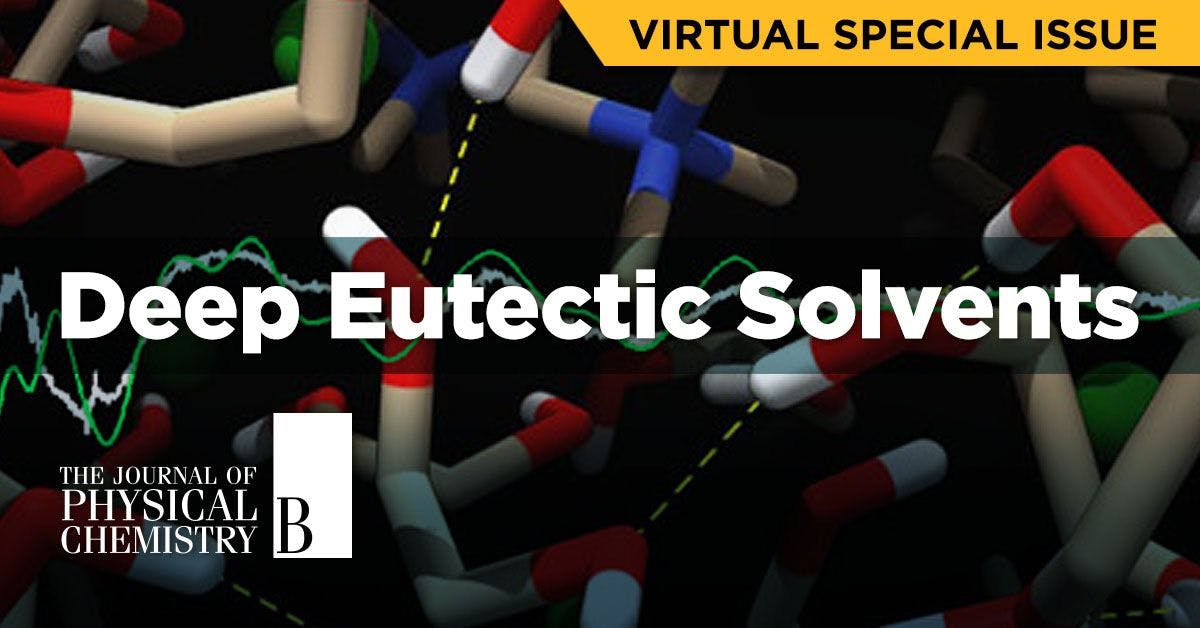
This virtual issue focuses on scientific and engineering advances related to Deep Eutectic Solvents. It includes papers that have appeared in the last two years in ACS Sustainable Chemistry & Engineering , Industrial & Engineering Chemistry Research , Journal of Chemical & Engineering Data , and Journal of Physical Chemistry B and C .
Celebrating ACS Sensors ‘ Editorial Advisory Board
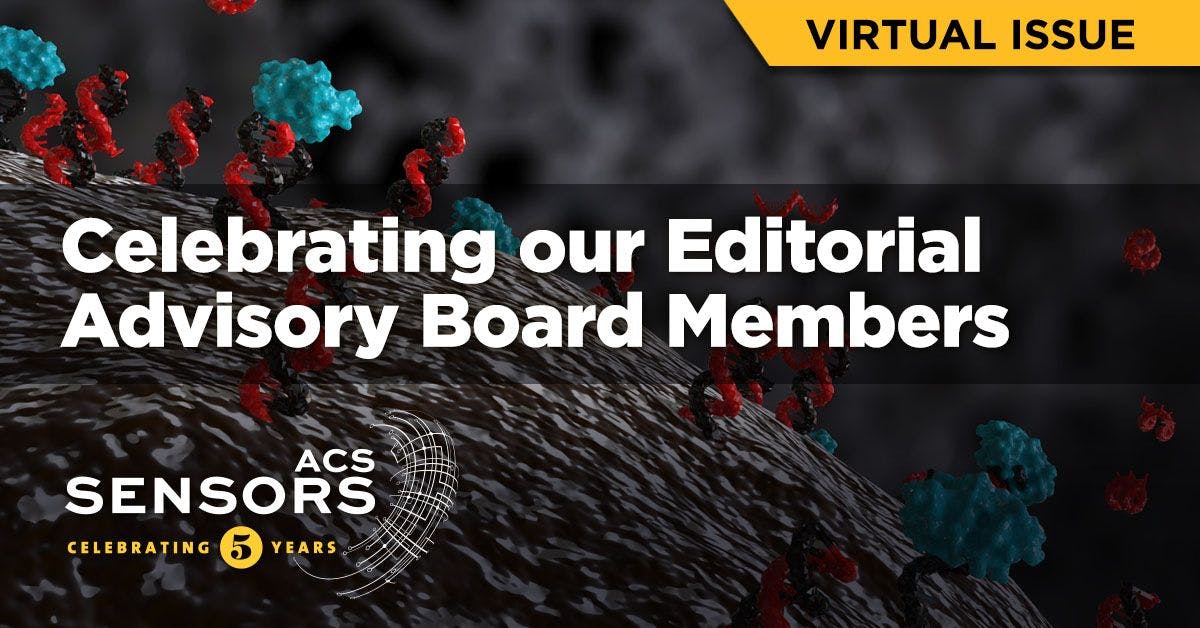
Metal-Organic Frameworks: Fundamental Study and Applications
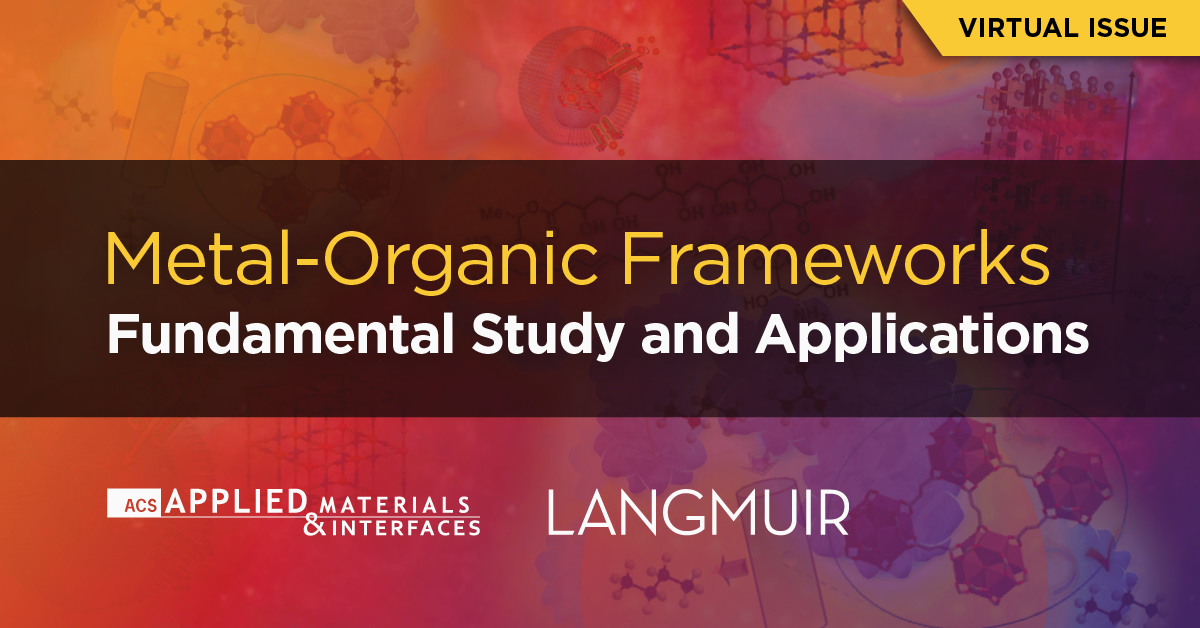
Exciting developments in metal-organic frameworks (MOFs) are the focus of this Virtual Issue that is jointly produced by Langmuir and ACS Applied Materials & Interfaces ( ACS AMI ). These two journals publish complementary and ground-breaking work on interfacial science. ACS AMI has a strong focus on practical applications whereas Langmuir encourages reports of both fundamental and applied nature, when rational design is a highlighted feature of the work.
Inorganic Synthesis in Uncommon Reaction Media
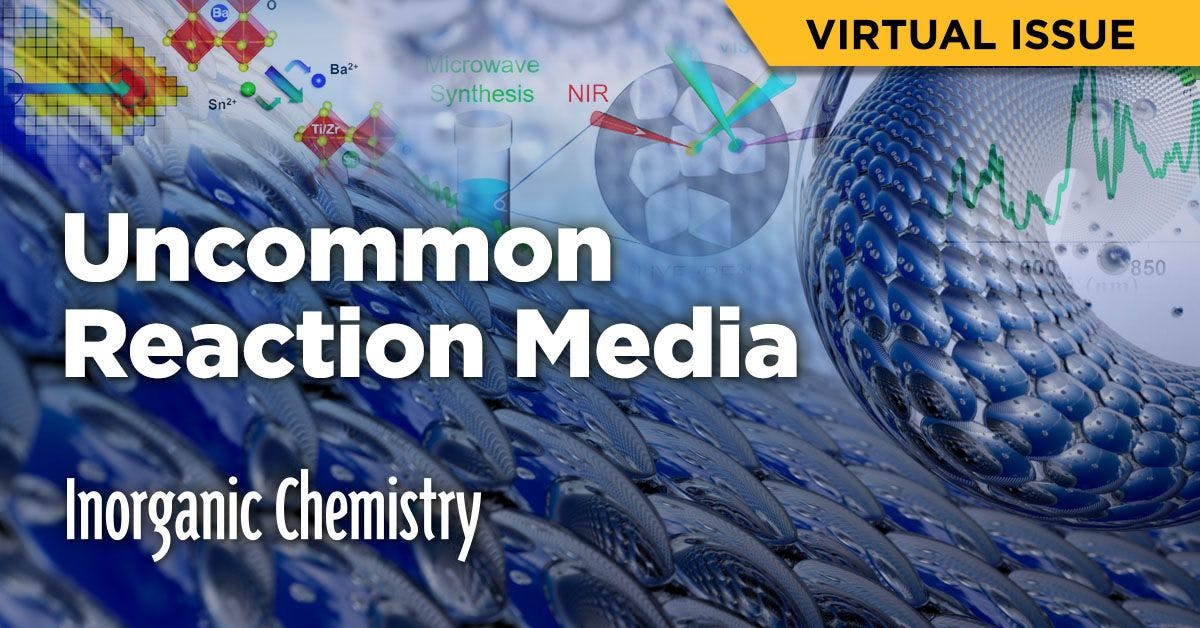
Water and organic solvents have long been the most common reaction media for chemical synthesis. Nevertheless, given limits in solubility and the need for extreme temperatures sometimes, especially for inorganic substances, chemists have had a growing interest in moving to “uncommon” reaction media to improve the access to certain compounds or to permit the fundamental study of the behavior of chemicals under unique conditions. In this Virtual Issue, “Inorganic Synthesis in Uncommon Reaction Media,” Guest Editor Julia Chan and Associate Editor Stefanie Dehnen highlight recent reports from Inorganic Chemistry and additionally from Chemistry of Materials and Crystal Growth & Design that feature reactions taking place in currently used uncommon systems: molten metals (metal flux), molten salts (nonmetal flux), ionic liquids (ionothermal if carried out under elevated temperatures), supercritical solvents (solvothermal), and liquefied gases.
The Challenge of Antibacterial Drug Permeation and Current Advances
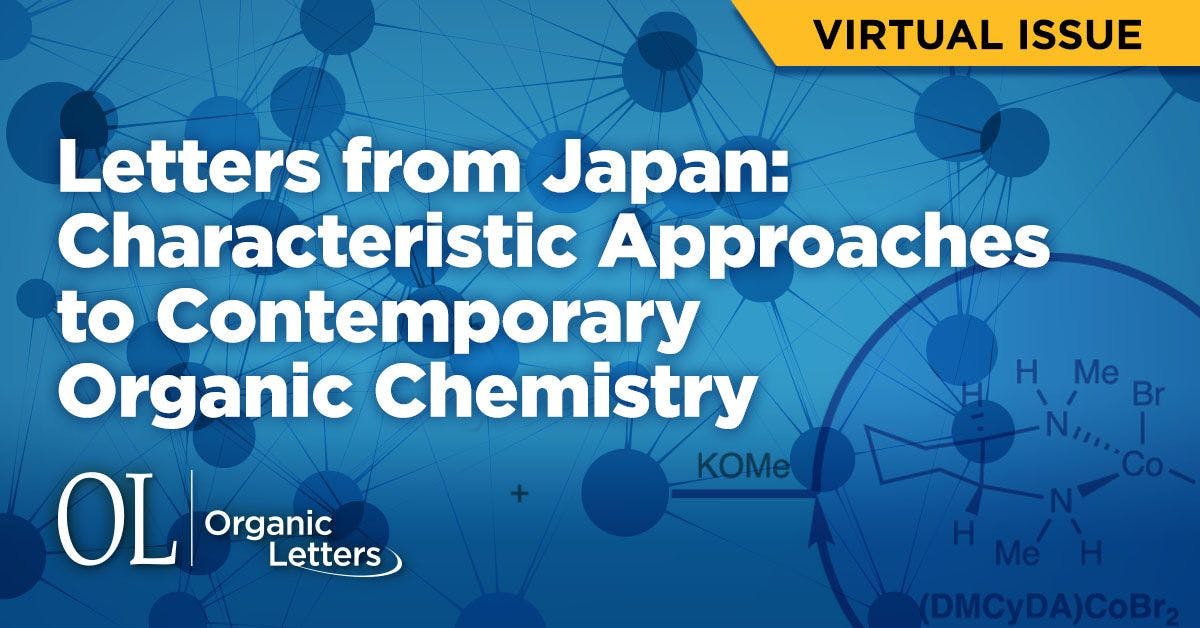
Recent advances in the area of drug permeation feed the pipeline of antibacterial agents with new and improved activities and keep the ever-changing landscape of antibiotic resistance effectively managed by small molecule therapeutics. The articles included in this Virtual Issue broadly represent three areas of research: 1) new experimental approaches to analyze intracellular accumulation of compounds in whole cells and compound permeation across model membranes; 2) new computational models of drug permeation across the outer membrane and integrated kinetic models of drug permeation across membranes with active efflux; and 3) new antibiotic screening campaigns and exploration of synergistic drug combinations bypassing bacterial permeation barriers.
Organic Chemistry in Japan: A Strong Foundation and Honorable Tradition
Organic chemistry has a strong foundation and honorable tradition in Japan, centering in recent decades predominantly on the development of synthetic methodologies, particularly in an interdisciplinary fashion focusing on cross-coupling and C-H activation and functionalization, the total synthesis of natural products, chemical biology research, supramolecular chemistry, and applications of (opto)electronic materials—all with an eye toward fostering international collaborations. This new Organic Letters Virtual Issue features 25 selected articles form 2019-2020 to highlight these achievements.
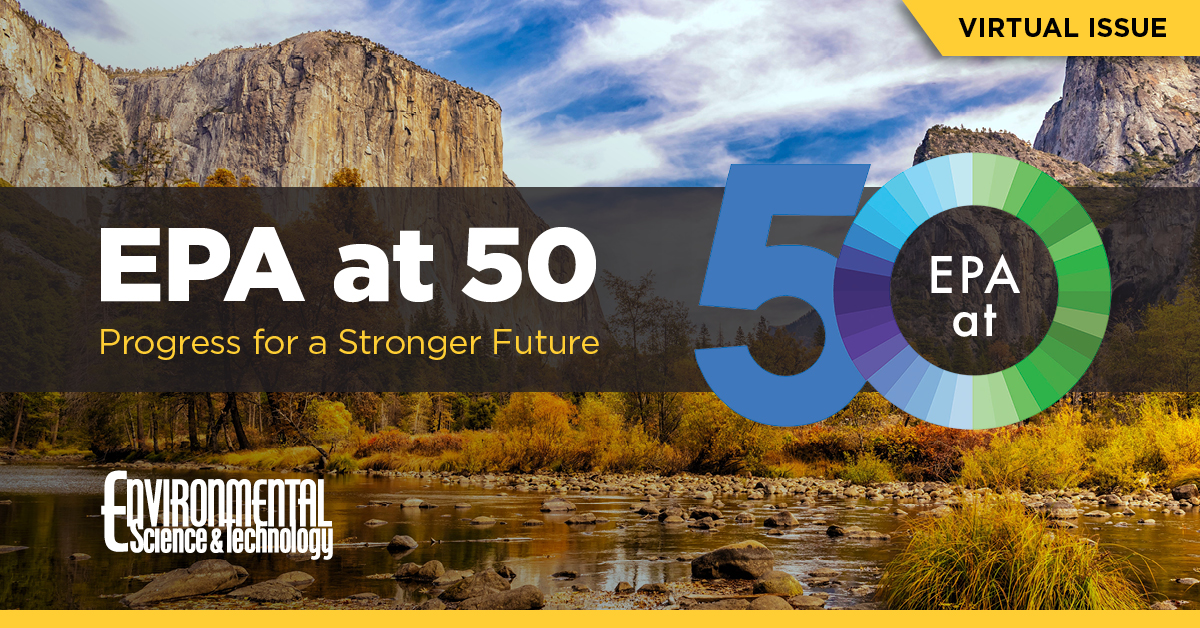
This virtual issue in Environmental Science & Technology ( ES&T ) marks the 50-year anniversary of the United States Environmental Protection Agency (US EPA). Recognizing this significant milestone brings an opportunity to reflect on the enormous achievements and impact this federal agency has had on the remediation and protection of the environment, reaching both domestically within the USA and globally since its official beginnings on December 2nd, 1970.
Bioconjugate Chemistry 30th Anniversary Reviews
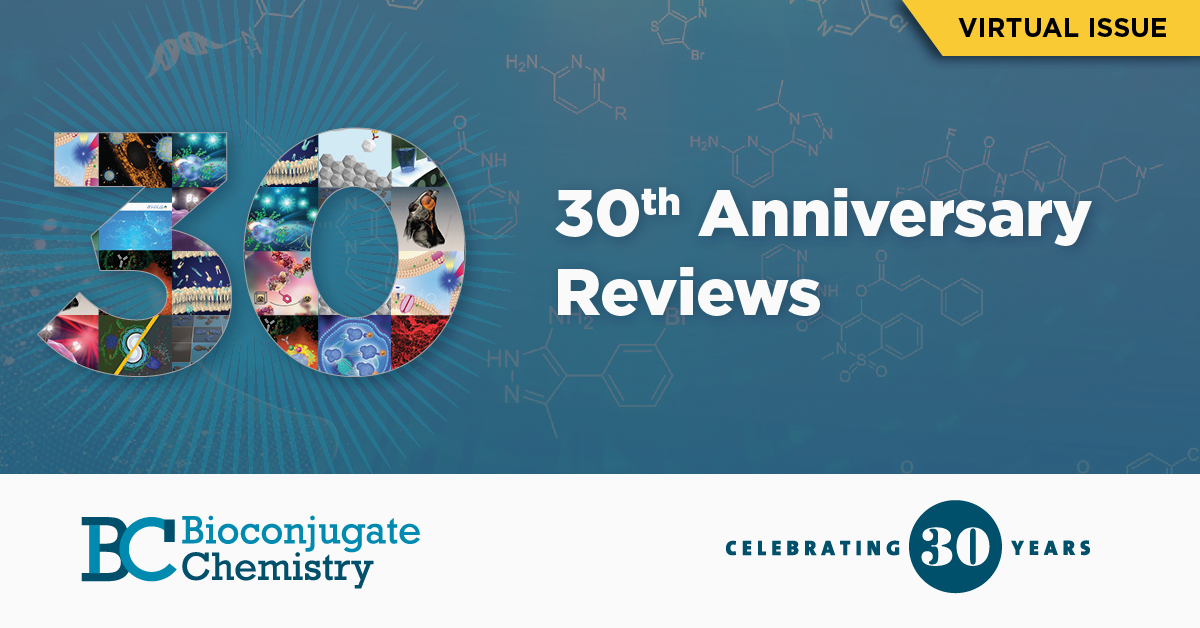
The breadth and impact of these 30th anniversary reviews demonstrate how the Bioconjugate Chemistry of today continues the forward-looking embrace of new science and systems while maintaining the old-fashioned virtues of scientific rigor and reproducibility.
Want the latest stories delivered to your inbox each month?
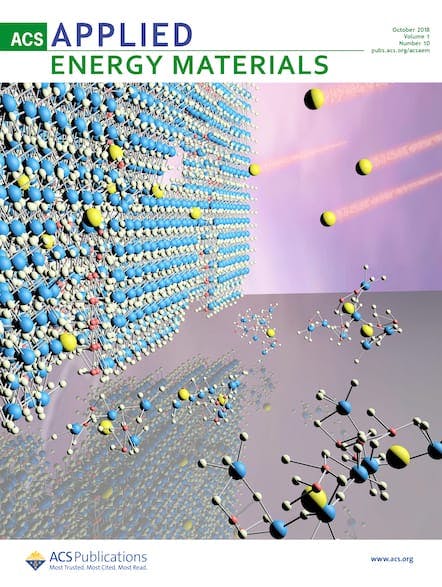
ACS Applied Energy Materials
Students & Educators —Menu
- Educational Resources
- Educators & Faculty
- College Planning
- ACS ChemClub
- Project SEED
- U.S. National Chemistry Olympiad
- Student Chapters
- ACS Meeting Information
- Undergraduate Research
- Internships, Summer Jobs & Coops
- Study Abroad Programs
- Finding a Mentor
- Two Year/Community College Students
- Social Distancing Socials
Planning for Graduate School
- Grants & Fellowships
- Career Planning
- International Students
- Planning for Graduate Work in Chemistry
- ACS Bridge Project
- Graduate Student Organizations (GSOs)
- Schedule-at-a-Glance
- Standards & Guidelines
- Explore Chemistry
- Science Outreach
- Publications
- ACS Student Communities
- You are here:
- American Chemical Society
- Students & Educators
- Undergraduate
- Undergraduate Research Guide
Undergraduate Research in Chemistry Guide
Research is the pursuit of new knowledge through the process of discovery. Scientific research involves diligent inquiry and systematic observation of phenomena. Most scientific research projects involve experimentation, often requiring testing the effect of changing conditions on the results. The conditions under which specific observations are made must be carefully controlled, and records must be meticulously maintained. This ensures that observations and results can be are reproduced. Scientific research can be basic (fundamental) or applied. What is the difference? The National Science Foundation uses the following definitions in its resource surveys:
- Basic research The objective of basic research is to gain more comprehensive knowledge or understanding of the subject under study, without specific applications in mind. In industry, basic research is defined as research that advances scientific knowledge but does not have specific immediate commercial objectives, although it may be in fields of present or potential commercial interest.
- Applied research Applied research is aimed at gaining knowledge or understanding to determine the means by which a specific, recognized need may be met. In industry, applied research includes investigations oriented to discovering new scientific knowledge that has specific commercial objectives with respect to products, processes, or services.
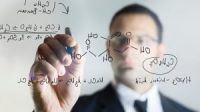
Get on the path to graduate school with our comprehensive guide to selecting an institution and preparing for graduate studies.
What is research at the undergraduate level?
At the undergraduate level, research is self-directed work under the guidance and supervision of a mentor/advisor ― usually a university professor. A gradual transition towards independence is encouraged as a student gains confidence and is able to work with minor supervision. Students normally participate in an ongoing research project and investigate phenomena of interest to them and their advisor. In the chemical sciences, the range of research areas is quite broad. A few groups maintain their research area within a single classical field of analytical, inorganic, organic, physical, chemical education or theoretical chemistry. More commonly, research groups today are interdisciplinary, crossing boundaries across fields and across other disciplines, such as physics, biology, materials science, engineering and medicine.
What are the benefits of being involved in undergraduate research?
There are many benefits to undergraduate research, but the most important are:
- Learning, learning, learning. Most chemists learn by working in a laboratory setting. Information learned in the classroom is more clearly understood and it is more easily remembered once it has been put into practice. This knowledge expands through experience and further reading. From the learning standpoint, research is an extremely productive cycle.
- Experiencing chemistry in a real world setting. The equipment, instrumentation and materials used in research labs are generally more sophisticated, advanced, and of far better quality than those used in lab courses
- Getting the excitement of discovery. If science is truly your vocation, regardless of any negative results, the moment of discovery will be truly exhilarating. Your results are exclusive. No one has ever seen them before.
- Preparing for graduate school. A graduate degree in a chemistry-related science is mostly a research degree. Undergraduate research will not only give you an excellent foundation, but working alongside graduate students and post-doctorates will provide you with a unique opportunity to learn what it will be like.
Is undergraduate research required for graduation?
Many chemistry programs now require undergraduate research for graduation. There are plenty of opportunities for undergraduate students to get involved in research, either during the academic year, summer, or both. If your home institution is not research intensive, you may find opportunities at other institutions, government labs, and industries.
What will I learn by participating in an undergraduate research program?
Conducting a research project involves a series of steps that start at the inquiry level and end in a report. In the process, you learn to:
- Conduct scientific literature searches
- Read, interpret and extract information from journal articles relevant to the project
- Design experimental procedures to obtain data and/or products of interest
- Operate instruments and implement laboratory techniques not usually available in laboratories associated with course work
- Interpret results, reach conclusions, and generate new ideas based on results
- Interact professionally (and socially) with students and professors within the research group, department and school as well as others from different schools, countries, cultures and backgrounds
- Communicate results orally and in writing to other peers, mentors, faculty advisors, and members of the scientific community at large via the following informal group meeting presentations, reports to mentor/advisor, poster presentations at college-wide, regional, national or international meetings; formal oral presentations at scientific meetings; or journal articles prepared for publication
When should I get involved in undergraduate research?
Chemistry is an experimental science. We recommended that you get involved in research as early in your college life as possible. Ample undergraduate research experience gives you an edge in the eyes of potential employers and graduate programs.
While most mentors prefer to accept students in their research labs once they have developed some basic lab skills through general and organic lab courses, some institutions have programs that involve students in research projects the summer prior to their freshman year. Others even involve senior high school students in summer research programs. Ask your academic/departmental advisor about the options available to you.
How much time should I allocate to research?
The quick answer is as much as possible without jeopardizing your course work. The rule of thumb is to spend 3 to 4 hours working in the lab for every credit hour in which you enroll. However, depending on the project, some progress can be achieved in just 3-4 hours of research/week. Most advisors would recommend 8-10 hours/week.
Depending on your project, a few of those hours may be of intense work and the rest may be spent simply monitoring the progress of a reaction or an instrumental analysis. Many research groups work on weekends. Saturdays are excellent days for long, uninterrupted periods of lab work.
How do I select an advisor?
This is probably the most important step in getting involved in undergraduate research. The best approach is multifaceted. Get informed about research areas and projects available in your department, which are usually posted on your departmental website under each professor’s name.
Talk to other students who are already involved in research. If your school has an ACS Student Chapter , make a point to talk to the chapter’s members. Ask your current chemistry professor and lab instructor for advice. They can usually guide you in the right direction. If a particular research area catches your interest, make an appointment with the corresponding professor.
Let the professor know that you are considering getting involved in research, you have read a bit about her/his research program, and that you would like to find out more. Professors understand that students are not experts in the field, and they will explain their research at a level that you will be able to follow. Here are some recommended questions to ask when you meet with this advisor:
- Is there a project(s) within her/his research program suitable for an undergraduate student?
- Does she/he have a position/space in the lab for you?
- If you were to work in her/his lab, would you be supervised directly by her/him or by a graduate student? If it is a graduate student, make a point of meeting with the student and other members of the research group. Determine if their schedule matches yours. A night owl may not be able to work effectively with a morning person.
- Does she/he have funding to support the project? Unfunded projects may indicate that there may not be enough resources in the lab to carry out the project to completion. It may also be an indication that funding agencies/peers do not consider this work sufficiently important enough for funding support. Of course there are exceptions. For example, a newly hired assistant professor may not have external funding yet, but he/she may have received “start-up funds” from the university and certainly has the vote of confidence of the rest of the faculty. Otherwise he/she would not have been hired. Another classical exception is computational chemistry research, for which mostly fast computers are necessary and therefore external funding is needed to support research assistants and computer equipment only. No chemicals, glassware, or instrumentation will be found in a computational chemistry lab.
- How many of his/her articles got published in the last two or three years? When prior work has been published, it is a good indicator that the research is considered worthwhile by the scientific community that reviews articles for publication. Ask for printed references. Number of publications in reputable refereed journals (for example ACS journals) is an excellent indicator of the reputation of the researcher and the quality of his/her work.
Here is one last piece of advice: If the project really excites you and you get satisfactory answers to all your questions, make sure that you and the advisor will get along and that you will enjoy working with him/her and other members of the research group.
Remember that this advisor may be writing recommendation letters on your behalf to future employers, graduate schools, etc., so you want to leave a good impression. To do this, you should understand that the research must move forward and that if you become part of a research team, you should do your best to achieve this goal. At the same time, your advisor should understand your obligations to your course work and provide you with a degree of flexibility.
Ultimately, it is your responsibility to do your best on both course work and research. Make sure that the advisor is committed to supervising you as much as you are committed to doing the required work and putting in the necessary/agreed upon hours.
What are some potential challenges?
- Time management . Each project is unique, and it will be up to you and your supervisor to decide when to be in the lab and how to best utilize the time available to move the project forward.
- Different approaches and styles . Not everyone is as clean and respectful of the equipment of others as you are. Not everyone is as punctual as you are. Not everyone follows safety procedures as diligently as you do. Some groups have established protocols for keeping the lab and equipment clean, for borrowing equipment from other members, for handling common equipment, for research meetings, for specific safety procedures, etc. Part of learning to work in a team is to avoid unnecessary conflict while establishing your ground to doing your work efficiently.
- “The project does not work.” This is a statement that advisors commonly hear from students. Although projects are generally very well conceived, and it is people that make projects work, the nature of research is such that it requires patience, perseverance, critical thinking, and on many occasions, a change in direction. Thoroughness, attention to detail, and comprehensive notes are crucial when reporting the progress of a project.
Be informed, attentive, analytical, and objective. Read all the background information. Read user manuals for instruments and equipment. In many instances the reason for failure may be related to dirty equipment, contaminated reagents, improperly set instruments, poorly chosen conditions, lack of thoroughness, and/or lack of resourcefulness. Repeating a procedure while changing one parameter may work sometimes, while repeating the procedure multiple times without systematic changes and observations probably will not.
When reporting failures or problems, make sure that you have all details at hand. Be thorough in you assessment. Then ask questions. Advisors usually have sufficient experience to detect errors in procedures and are able to lead you in the right direction when the student is able to provide all the necessary details. They also have enough experience to know when to change directions. Many times one result may be unexpected, but it may be interesting enough to lead the investigation into a totally different avenue. Communicate with your advisor/mentor often.
Are there places other than my institution where I can conduct research?
Absolutely! Your school may be close to other universities, government labs and/or industries that offer part-time research opportunities during the academic year. There may also be summer opportunities in these institutions as well as in REU sites (see next question).
Contact your chemistry department advisor first. He/she may have some information readily available for you. You can also contact nearby universities, local industries and government labs directly or through the career center at your school. You can also find listings through ACS resources:
- Research Opportunities (US only)
- International Research Opportunities
- Internships and Summer Jobs
What are Research Experiences for Undergraduates (REU) sites? When should I apply for a position in one of them?
REU is a program established by the National Science Foundation (NSF) to support active research participation by undergraduate students at host institutions in the United States or abroad. An REU site may offer projects within a single department/discipline or it may have projects that are inter-departmental and interdisciplinary. There are currently over 70 domestic and approximately 5 international REU sites with a chemistry theme. Sites consist of 10-12 students each, although there are larger sites that supplement NSF funding with other sources. Students receive stipends and, in most cases, assistance with housing and travel.
Most REU sites invite rising juniors and rising seniors to participate in research during the summer. Experience in research is not required to apply, except for international sites where at least one semester or summer of prior research experience is recommended. Applications usually open around November or December for participation during the following summer. Undergraduate students supported with NSF funds must be citizens or permanent residents of the United States or its possessions. Some REU sites with supplementary funds from other sources may accept international students that are enrolled at US institutions.
- Get more information about REU sites
How do I prepare a scientific research poster?
Here are some links to sites with very useful information and samples.
- How to Prepare a Proper Scientific Paper or Poster
- Creating Effective Poster Presentations
- Designing Effective Poster Presentations
Research and Internship Opportunities
- Internships and Fellowships Find internships, fellowships, and cooperative education opportunities.
- SCI Scholars Internship Program Industrial internships for chemistry and chemical engineering undergraduates.
- ACS International Center Fellowships, scholarships, and research opportunities around the globe
Accept & Close The ACS takes your privacy seriously as it relates to cookies. We use cookies to remember users, better understand ways to serve them, improve our value proposition, and optimize their experience. Learn more about managing your cookies at Cookies Policy .
1155 Sixteenth Street, NW, Washington, DC 20036, USA | service@acs.org | 1-800-333-9511 (US and Canada) | 614-447-3776 (outside North America)
- Terms of Use
- Accessibility
Copyright © 2024 American Chemical Society
Thank you for visiting nature.com. You are using a browser version with limited support for CSS. To obtain the best experience, we recommend you use a more up to date browser (or turn off compatibility mode in Internet Explorer). In the meantime, to ensure continued support, we are displaying the site without styles and JavaScript.
- View all journals
- Explore content
- About the journal
- Publish with us
- Sign up for alerts
Collection
Open Questions in Chemistry
In spite of decades of research and the enormous progress made, chemists continue to grapple with poorly understood aspects of the world around us. Indeed, many fundamental questions remain— will we ever get to the bottom of the different structures of ice, or pinpoint the origins of life on Earth, or obtain a full picture of the complexity of carbon-based molecules in space?
This Collection aims to uncover open questions across the breadth of the chemical sciences. Each Comment article provides an overview of a focused field of research, identifies key open questions, and gives expert opinion on how challenges in answering these questions might be overcome. Our hope is that this series will be of interest to the chemistry community as a whole, and that it will stimulate those working in or adjacent to the research fields that are covered to think about some of the open questions that invite further study.
While the below Comment articles delve into a wide range of important and exciting topics, when one considers the breadth of ongoing research in fundamental areas of chemistry, we have only just begun to scratch the surface. We look forward to publishing more in this series in the months and years to come, and invite experts to submit their own contributions to this exciting Collection.
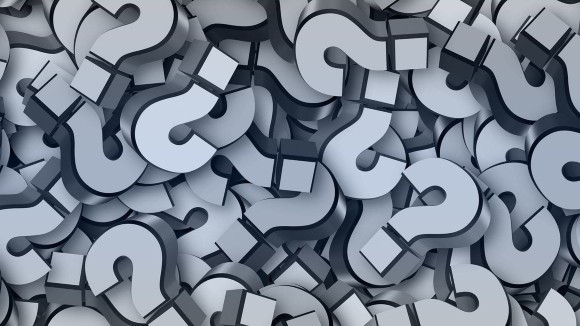
- Collection content
- How to submit
- About the Editors
- About this Collection
Open questions in chemistry
Today, Communications Chemistry launches a series of Comment articles discussing key open questions in specific fields of fundamental chemical research. Here we outline the aims of this series and highlight each contribution within.
Organic chemistry and chemical biology
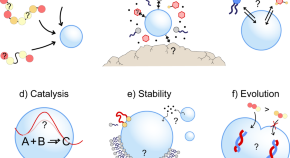
Open questions on liquid–liquid phase separation
Liquid-liquid phase separation (LLPS) underlies the formation of intracellular membraneless compartments in biology and may have played a role in the formation of protocells that concentrate key chemicals during the origins of life. While LLPS of simple systems, such as oil and water, is well understood, many aspects of LLPS in complex, out-of-equilibrium molecular systems remain elusive. Here, the author discusses open questions and recent insights related to the formation, function and fate of such condensates both in cell biology and protocell research.
- Evan Spruijt
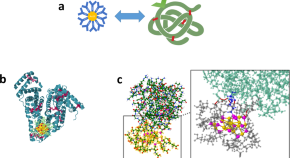
Open questions on proteins interacting with nanoclusters
Interfacing ultrasmall metal nanoclusters (NCs) with proteins can present a dual opportunity: proteins can be used for protecting NCs, and the surface ligands of NCs may interact with proteins. Here, the authors identify and discuss remaining open questions surrounding the bio-NC interface that call for future research efforts.
- Rodolphe Antoine
- Dusica Maysinger
- Vlasta Bonačić-Koutecký
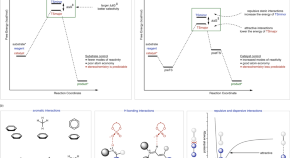
Open questions on the transfer of chirality
The transfer of chiral information from optically pure reaction components to products can generate enantiomerically-enriched molecules, but the control of stereochemistry often proves challenging. Here, the author highlights how our fundamental understanding of stereocontrol has evolved and discusses possible approaches for the rational development of enantioselective catalysts.
- Jolene P. Reid
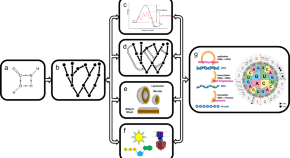
Open questions in understanding life’s origins
The chemical space of prebiotic chemistry is extremely large, while extant biochemistry uses only a few thousand interconnected molecules. Here we discuss how the connection between these two regimes can be investigated, and explore major outstanding questions in the origin of life.
- Christopher J. Butch
- Markus Meringer
- H. James Cleaves II
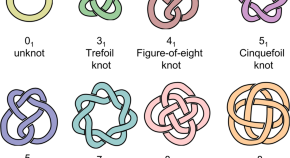
Open questions in functional molecular topology
Molecular knots are evolving from academic curiosities to a practically useful class of mechanically interlocked molecules, capable of performing unique tasks at the nanoscale. In this comment, the author discusses the properties of molecular knots, and highlights future challenges for chemical topology.
- Fredrik Schaufelberger
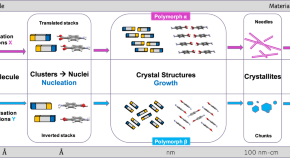
Open questions in organic crystal polymorphism
Polymorphs, crystals with different structure and properties but the same molecular composition, arise from the subtle interplay between thermodynamics and kinetics during crystallisation. In this opinion piece, the authors review the latest developments in the field of polymorphism and discuss standing open questions.
- Aurora J. Cruz-Cabeza
- Neil Feeder
- Roger J. Davey
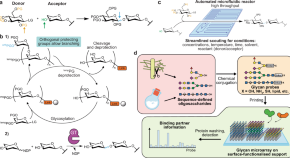
Open questions in chemical glycobiology
Glycans are ubiquitous in biology, but their complex structure and biosynthesis have challenged research of their wide-ranging roles. Here, the authors comment on current trends on the role of chemical methodologies in the field of glycobiology.
- Mia I. Zol-Hanlon
- Benjamin Schumann
Inorganic chemistry
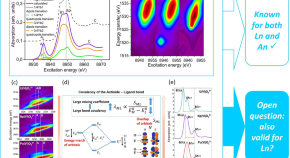
Open questions on bonding involving lanthanide atoms
In-depth understanding of the bonding characteristics of the lanthanide ions in contemporary lanthanide-based materials is mandatory for tailoring their properties for novel applications. Here, the authors elaborate on open questions regarding the bonding situation in mainly molecular lanthanide (4f) compounds, where, as compared to their actinide (5f) analogs in which covalency of the bonds is a common feature, this is still under discussion for the 4f compounds.
- P. W. Roesky
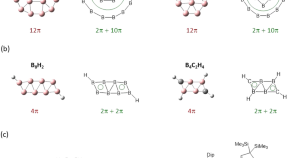
Open questions in boron species with globally 4 n π systems
The Hückel rule defines that monocyclic and planar conjugated systems containing [4 n + 2] π electrons are aromatic. Here, the authors highlight boron species that feature a globally 4 n π system, defying the Hückel rule, but nonetheless exhibit aromaticity.
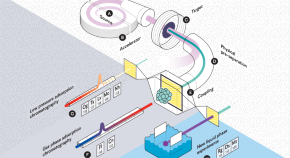
Open questions on chemistry in the synthesis and characterization of superheavy elements
Superheavy elements are ideal for furthering our understanding of relativistic effects and how they affect physicochemical properties of heavy elements. In this comment, the author discusses the role of chemistry in the synthesis of new elements before addressing the future challenges concerning the chemical characterization of superheavy elements.
- Patrick Steinegger
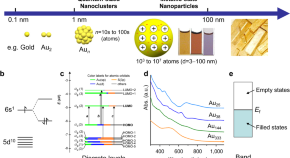
Open questions on the transition between nanoscale and bulk properties of metals
Nanoscience has progressed tremendously in the exploration of new phenomena not seen in bulk materials, however, the transition between nanoscale and bulk properties is not yet fully understood. Here the authors identify and discuss remaining open questions that call for future efforts.
- Rongchao Jin
- Tatsuya Higaki
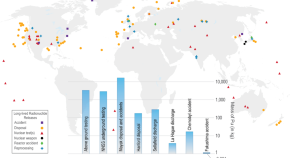
Open questions on the environmental chemistry of radionuclides
Understanding the biogeochemistry of radionuclides in the environment is essential for effective isolation of nuclear waste in repositories, management of contaminated sites, ensuring long-term protection of our ecosystems, and limiting impacts on human health. Here the authors discuss the extreme complexity of this multidimensional chemistry problem, highlighting the outstanding open questions for the next generations of environmental radiochemists.
- Gauthier J.-P. Deblonde
- Annie B. Kersting
- Mavrik Zavarin
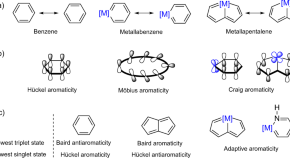
Open questions on aromaticity in organometallics
While sp 2 -hybridized carbon atoms in hydrocarbons typically contribute only one electron to their aromaticity, metals have more electrons from d or f orbitals available for participating in conjugation in organometallics, complicating the electron counting as well as analysis of their aromaticity. Here, the author comments on the challenges towards understanding aromaticity in organometallics and outlines several remaining questions that have yet to be answered.
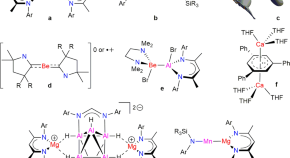

Open questions in low oxidation state group 2 chemistry
The chemistry of stable low oxidation state group 2 metal compounds was initiated in 2007 and has since expanded rapidly, yielding many surprises. Here the author outlines advances in the field and discusses some of the open questions and challenges that remain to be answered in coming years.
- Cameron Jones
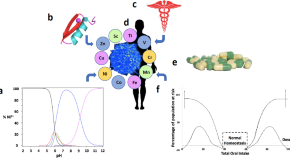
Open questions on the biological roles of first-row transition metals
First-row transition metals play several roles in biological processes and in medicine, but can be toxic in high concentrations. Here the authors comment on the sensitive biochemistry and speciation chemistry of the first-row transition metals, and outline some of the remaining questions that have yet to be answered.
- Debbie C. Crans
- Kateryna Kostenkova
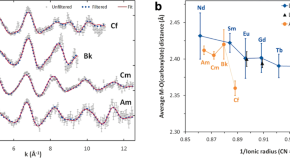
Open questions in transplutonium coordination chemistry
Over the past decade, momentous progress has been made in the characterization of late actinide compounds. Here the authors highlight how advances in spectroscopic and computational tools have developed our understanding of fundamental transplutonium bonding interactions, and discuss whether covalency and heterogeneity changes in 5f-orbital bonding could be harnessed in environmentally and industrially relevant systems.
- Korey P. Carter
- Roger M. Pallares
- Rebecca J. Abergel
Physical chemistry
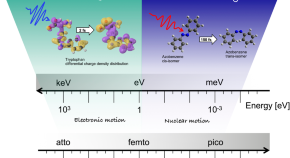
Open questions in attochemistry
Attosecond science is nowadays a well-established research field, and table-top attosecond sources based on high-harmonic generation are routinely used to access electronic motion in matter at its natural time scale. Here, the authors describe a new way of doing chemistry—attochemistry—by directly acting on the electronic motion, and discuss a few key open questions in this emerging field.
- Francesca Calegari
- Fernando Martin
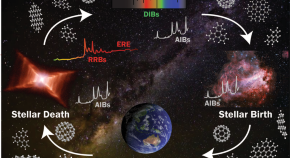
Open questions on carbon-based molecules in space
It has been a great joint achievement of astronomy, laboratory spectroscopy and quantum chemistry to identify interstellar molecules in various astronomical environments and piece together their origins story from the fragmented evidence. Here the authors provide a sketch of what we know and motivate the asking of open questions on carbon-based molecules in space.
- Christopher S. Hansen
- Els Peeters
- Timothy W. Schmidt
Open questions on emergence in chemistry
Strong emergence is the main form of emergence that has been defended with respect to chemistry, and in particular molecular structure. Here, the author spells out this form of emergence, proposes new ways in which one can further explore the question of emergence, and explains why investigating emergence should be of interest not only to philosophers but to chemists as well.
- Vanessa A. Seifert
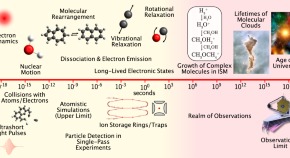
Open questions on the interaction dynamics of molecules and clusters in the gas phase
Emerging experimental techniques combined with theoretical advances allow unprecedented studies of the dynamics of gas phase molecules and clusters induced in interactions with photons, electrons, or heavy particles. Here, the authors highlight recent advances, key open questions, and challenges in this field of research with focus on experimental studies of dynamics of ions stored on millisecond timescales and beyond, and its applications in astrochemistry and astronomy.
- Michael Gatchell
- Henning Zettergren
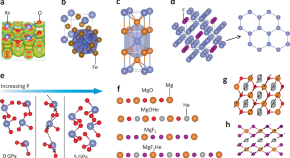
Open questions on the high-pressure chemistry of the noble gases
Recent high-pressure studies have uncovered many types of chemical bonds present in noble gas compounds. Here, by extrapolating what has been found so far, the authors discuss which future discoveries can be expected and recommend further avenues of exploration.
- Maosheng Miao
- Yuanhui Sun
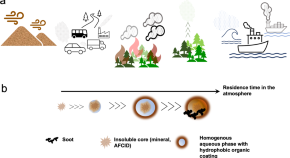
Open questions on transition metals driving secondary thermal processes in atmospheric aerosols
Transition metals are increasingly recognized as key drivers in the formation and aging of light-absorbing organic aerosols, known as brown carbon, which impact the energy flux in the atmosphere. Here the authors discuss somewhat overlooked condensed phase chemical processes and identify research needs to improve our fundamental understanding of atmospheric aerosols and ultimately reduce modelling uncertainties of the direct and indirect effects of aerosol particles on the climate.
- Hind A. Al-Abadleh
- Sergey A. Nizkorodov
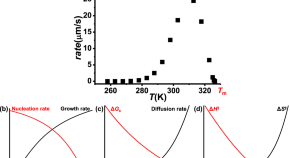
Open questions on physical chemistry of crystal growth from congruent melts
Crystallization is observed in both nature and in the lab, and is critical to diverse areas of science and technology. Here, the author summarizes the theories that have been proposed to explain crystal growth from melts, and raises some open questions that remain.
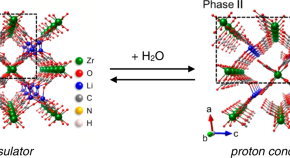
Open questions on water confined in nanoporous materials
Water adsorption in soft nanoporous materials can trigger large-scale structural transitions and introduce new properties in the confined water phase. Here, we look at some of the outstanding questions in this lively field of research.
- François-Xavier Coudert
- Anne Boutin
- Alain H. Fuchs
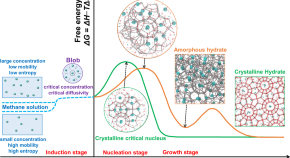
Open questions on methane hydrate nucleation
The commercial use of natural methane hydrate is hampered by several open questions that remain regarding hydrate formation. Here the authors comment on past interpretations and aim to provide a roadmap for developing a predictive theory of methane hydrate nucleation.
- Guang-Jun Guo
- Zhengcai Zhang
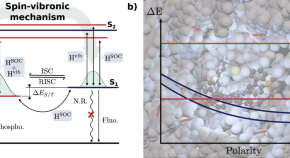
Open questions on the photophysics of thermally activated delayed fluorescence
The process of thermally activated delayed fluorescence (TADF) converts non-radiative triplet states into emissive singlet states. Herein we outline the fundamentals of TADF, some of the recent progress in understanding the key material properties responsible for promoting TADF and finally discuss some remaining challenges for the potential applications of this phenomenon.
- Thomas J. Penfold
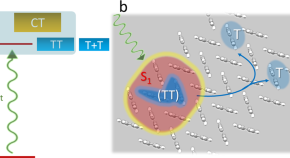
Open questions on the photophysics of ultrafast singlet fission
Ultrafast singlet fission has the potential to facilitate highly efficient photovoltaics through the multiplication of excitons in organic molecular architectures. Here, we consider the interplay of molecular structure and intermolecular coupling toward enabling ultrafast singlet fission and discuss open questions in the field.
- Justin C. Johnson
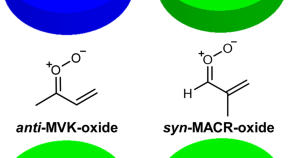
Open questions on the reactivity of Criegee intermediates
Criegee intermediates are reactive intermediates formed in Earth’s atmosphere through ozonolysis of alkenes. Here the authors outline the fundamental chemistry that influences their highly conformer- and substituent-dependent unimolecular and bimolecular reactivity, and discuss open questions of fundamental and atmospheric interest.
- Rebecca L. Caravan
- Michael F. Vansco
- Marsha I. Lester
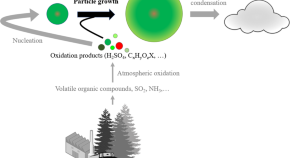
Open questions on atmospheric nanoparticle growth
Cloud droplets form in the atmosphere on aerosol particles, many of which result from nucleation of vapors. Here the authors comment on current knowledge and open questions regarding the condensational growth of nucleated particles to sizes where they influence cloud formation.
- Taina Yli-Juuti
- Claudia Mohr
- Ilona Riipinen
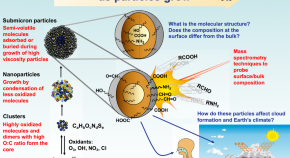
Open questions on the chemical composition of airborne particles
Airborne particles have significant impacts on health, visibility, and climate. Here, an overview of what is known about particle chemical composition is presented, along with open questions and challenges that are central to relating composition to life cycles and impacts.
- Barbara J. Finlayson-Pitts
- Lisa M. Wingen
- Michael J. Ezell
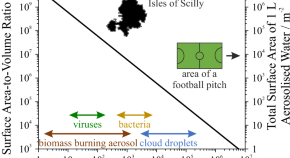
Open questions on the physical properties of aerosols
Aerosols are highly dynamic, non-equilibrium systems exhibiting unique microphysical properties relative to bulk systems. Here the authors discuss the roles aerosols play in (bio)chemical transformations and identify open questions in aerosol-mediated reaction rate accelerations, aerosol optical properties, and microorganism survival.
- Bryan R. Bzdek
- Jonathan P. Reid
- Michael I. Cotterell
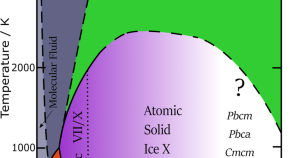
Open questions on the structures of crystalline water ices
Water can form a vast number of topological frameworks owing to its hydrogen-bonding ability, with 19 different forms of ice experimentally confirmed at present. Here, the authors comment on open questions and possible future discoveries, covering negative to ultrahigh pressures.
- Thomas Loerting
- Violeta Fuentes-Landete
- Tobias M. Gasser
Open questions on nonequilibrium thermodynamics of chemical reaction networks
Chemical reaction networks (CRNs) are prototypical complex systems because reactions are nonlinear and connected in intricate ways, and they are also essential to understand living systems. Here, the author discusses how recent developments in nonequilibrium thermodynamics provide new insight on how CRNs process energy and perform sophisticated tasks, and describes open challenges in the field.
- Massimiliano Esposito
Quick links
- Explore articles by subject
- Guide to authors
- Editorial policies

If you're seeing this message, it means we're having trouble loading external resources on our website.
If you're behind a web filter, please make sure that the domains *.kastatic.org and *.kasandbox.org are unblocked.
To log in and use all the features of Khan Academy, please enable JavaScript in your browser.
Chemistry library
Unit 1: atoms, compounds, and ions, unit 2: mass spectrometry, unit 3: chemical reactions and stoichiometry, unit 4: more about chemical reactions, unit 5: electronic structure of atoms, unit 6: periodic table, unit 7: chemical bonds, unit 8: gases and kinetic molecular theory, unit 9: states of matter and intermolecular forces, unit 10: chemical equilibrium, unit 11: acids and bases, unit 12: buffers, titrations, and solubility equilibria, unit 13: thermodynamics, unit 14: redox reactions and electrochemistry, unit 15: kinetics, unit 16: studying for the ap chemistry exam, unit 17: meet the chemistry professional.
October 1, 2011
17 min read
10 Unsolved Mysteries in Chemistry
Many of the most profound scientific questions—and some of humanity's most urgent problems—pertain to the science of atoms and molecules
By Philip Ball
1 How Did Life Begin? The moment when the first living beings arose from inanimate matter almost four billion years ago is still shrouded in mystery. How did relatively simple molecules in the primordial broth give rise to more and more complex compounds? And how did some of those compounds begin to process energy and replicate (two of the defining characteristics of life)? At the molecular level, all of those steps are, of course, chemical reactions, which makes the question of how life began one of chemistry.
The challenge for chemists is no longer to come up with vaguely plausible scenarios, of which there are plenty. For example, researchers have speculated about minerals such as clay acting as catalysts for the formation of the first self-replicating polymers (molecules that, like DNA or proteins, are long chains of smaller units); about chemical complexity fueled by the energy of deep-sea hydrothermal vents; and about an "RNA world," in which DNA’s cousin RNA—which can act as an enzyme and catalyze reactions the way proteins do—would have been a universal molecule, before DNA and proteins appeared.
No, the game is to figure out how to test these ideas in reactions coddled in the test tube. Researchers have shown, for example, that certain relatively simple chemicals can spontaneously react to form the more complex building blocks of living systems, such as amino acids and nucleotides, the basic units of DNA and RNA. In 2009 a team led by John Sutherland, now at the MRC Laboratory of Molecular Biology in Cambridge, England, was able to demonstrate the formation of nucleotides from molecules likely to have existed in the primordial broth. Other researchers have focused on the ability of some RNA strands to act as enzymes, providing evidence in support of the RNA world hypothesis. Through such steps, scientists may progressively bridge the gap from inanimate matter to self-replicating, self-sustaining systems.
On supporting science journalism
If you're enjoying this article, consider supporting our award-winning journalism by subscribing . By purchasing a subscription you are helping to ensure the future of impactful stories about the discoveries and ideas shaping our world today.
Now that scientists have a better view of strange and potentially fertile environments in our solar system—the occasional flows of water on Mars, the petrochemical seas of Saturn’s moon Titan, and the cold, salty oceans that seem to lurk under the ice of Jupiter’s moons Europa and Ganymede—the origin of terrestrial life seems only a part of grander questions: Under what circumstances can life arise? And how widely can its chemical basis vary? That issue is made richer still by the discovery, over the past 16 years, of more than 500 extrasolar planets orbiting other stars—worlds of bewildering variety.
These discoveries have pushed chemists to broaden their imagination about the possible chemistries of life. For instance, NASA has long pursued the view that liquid water is a prerequisite, but now scientists are not so sure. How about liquid ammonia, formamide, an oily solvent like liquid methane or supercritical hydrogen on Jupiter? And why should life restrict itself to DNA, RNA and proteins? After all, several artificial chemical systems have now been made that exhibit a kind of replication from the component parts without relying on nucleic acids. All you need, it seems, is a molecular system that can serve as a template for making a copy and then detach itself.
Looking at life on Earth, says chemist Steven Benner of the Foundation for Applied Molecular Evolution in Gainesville, Fla., “we have no way to decide whether the similarities [such as the use of DNA and proteins] reflect common ancestry or the needs of life universally.” But if we retreat into saying that we have to stick with what we know, he says, “we have no fun.”
2 How Do Molecules Form? Molecular structures may be a mainstay of high school science classes, but the familiar picture of balls and sticks representing atoms and the bonds among them is largely a conventional fiction. The trouble is that scientists disagree on what a more accurate representation of molecules should look like.
In the 1920s physicists Walter Heitler and Fritz London showed how to describe a chemical bond using the equations of then nascent quantum theory, and the great American chemist Linus Pauling proposed that bonds form when the electron orbitals of different atoms overlap in space. A competing theory by Robert Mulliken and Friedrich Hund suggested that bonds are the result of atomic orbitals merging into “molecular orbitals” that extend over more than one atom. Theoretical chemistry seemed about to become a branch of physics.
Nearly 100 years later the molecular-orbital picture has become the most common one, but there is still no consensus among chemists that it is always the best way to look at molecules. The reason is that this model of molecules and all others are based on simplifying assumptions and are thus approximate, partial descriptions. In reality, a molecule is a bunch of atomic nuclei in a cloud of electrons, with opposing electrostatic forces fighting a constant tug-of-war with one another, and all components constantly moving and reshuffling. Existing models of the molecule usually try to crystallize such a dynamic entity into a static one and may capture some of its salient properties but neglect others.
Quantum theory is unable to supply a unique definition of chemical bonds that accords with the intuition of chemists whose daily business is to make and break them. There are now many ways of describing molecules as atoms joined by bonds. According to quantum chemist Dominik Marx of Ruhr University Bochum in Germany, pretty much all such descriptions “are useful in some cases but fail in others.”
Computer simulations can now calculate the structures and properties of molecules from quantum first principles with great accuracy—as long as the number of electrons is relatively small. “Computational chemistry can be pushed to the level of utmost realism and complexity,” Marx says. As a result, computer calculations can increasingly be regarded as a kind of virtual experiment that predicts the course of a reaction. Once the reaction to be simulated involves more than a few dozen electrons, however, the calculations quickly begin to overwhelm even the most powerful supercomputer, so the challenge will be to see whether the simulations can scale up—whether, for example, complicated biomolecular processes in the cell or sophisticated materials can be modeled this way.
3 How Does the Environment Influence Our Genes? The old idea of biology was that who you are is a matter of which genes you have. It is now clear that an equally important issue is which genes you use. Like all of biology, this issue has chemistry at its core.
The cells of the early embryo can develop into any tissue type. But as the embryo grows, these so-called pluripotent stem cells differentiate, acquiring specific roles (such as blood, muscle or nerve cells) that remain fixed in their progeny. The formation of the human body is a matter of chemically modifying the stem cells’ chromosomes in ways that alter the arrays of genes that are turned on and off.
One of the revolutionary discoveries in research on cloning and stem cells, however, is that this modification is reversible and can be influenced by the body’s experiences. Cells do not permanently disable genes during differentiation, retaining only those they need in a “ready to work” state. Rather the genes that get switched off retain a latent ability to work—to give rise to the proteins they encode—and can be reactivated, for instance, by exposure to certain chemicals taken in from the environment.
What is particularly exciting and challenging for chemists is that the control of gene activity seems to involve chemical events happening at size scales greater than those of atoms and molecules—at the so-called mesoscale—with large molecular groups and assemblies interacting. Chromatin, the mixture of DNA and proteins that makes up chromosomes, has a hierarchical structure. The double helix is wound around cylindrical particles made from proteins called histones, and this string of beads is then bundled up into higher-order structures that are poorly understood. Cells exercise great control over this packing—how and where a gene is packed into chromatin may determine whether it is active or not.
Cells have specialized enzymes for reshaping chromatin structure, and these enzymes have a central role in cell differentiation. Chromatin in embryonic stem cells seems to have a much looser, open structure: as some genes fall inactive, the chromatin becomes increasingly lumpy and organized. “The chromatin seems to fix and maintain or stabilize the cells’ state,” says pathologist Bradley Bernstein of Massachusetts General Hospital.
What is more, such chromatin sculpting is accompanied by chemical modification of both DNA and histones. Small molecules attached to them act as labels that tell the cellular machinery to silence genes or, conversely, free them for action. This labeling is called “epigenetic” because it does not alter the information carried by the genes themselves.
The question of the extent to which mature cells can be returned to pluripotency—whether they are as good as true stem cells, which is a vital issue for their use in regenerative medicine—seems to hinge largely on how far the epigenetic marking can be reset.
It is now clear that beyond the genetic code that spells out many of the cells’ key instructions, cells speak in an entirely separate chemical language of genetics—that of epigenetics. “People can have a genetic predisposition to many diseases, including cancer, but whether or not the disease manifests itself will often depend on environmental factors operating through these epigenetic pathways,” says geneticist Bryan Turner of the University of Birmingham in England.
4 How Does the Brain Think and Form Memories? The brain is a chemical computer. Interactions between the neurons that form its circuitry are mediated by molecules: specifically, neurotransmitters that pass across the synapses, the contact points where one neural cell wires up to another. This chemistry of the mind is perhaps at its most impressive in the operation of memory, in which abstract principles and concepts—a telephone number, say, or an emotional association—are imprinted in states of the neural network by sustained chemical signals. How does chemistry create a memory that is both persistent and dynamic, as well as able to recall, revise and forget?
We now know parts of the answer. A cascade of biochemical processes, leading to a change in the amounts of neurotransmitter molecules in the synapse, triggers learning for habitual reflexes. But even this simple aspect of learning has short- and long-term stages. Meanwhile more complex so-called declarative memory (of people, places, and so on) has a different mechanism and location in the brain, involving the activation of a protein called the NMDA receptor on certain neurons. Blocking this receptor with drugs prevents the retention of many types of declarative memory.
Our everyday declarative memories are often encoded through a process called long-term potentiation, which involves NMDA receptors and is accompanied by an enlargement of the neuronal region that forms a synapse. As the synapse grows, so does the “strength” of its connection with neighbors—the voltage induced at the synaptic junction by arriving nerve impulses. The biochemistry of this process has been clarified in the past several years. It involves the formation of filaments within the neuron made from the protein actin—part of the basic scaffolding of the cell and the material that determines its size and shape. But that process can be undone during a short period before the change is consolidated if biochemical agents prevent the newly formed filaments from stabilizing.
Once encoded, long-term memory for both simple and complex learning is actively maintained by switching on genes that give rise to particular proteins. It now appears that this process can involve a type of molecule called a prion. Prions are proteins that can switch between two different conformations. One of the conformations is soluble, whereas the other is insoluble and acts as a catalyst to switch other molecules like it to the insoluble state, leading these molecules to aggregate. Prions were first discovered for their role in neurodegenerative conditions such as mad cow disease, but prion mechanisms have now been found to have beneficial functions, too: the formation of a prion aggregate marks a particular synapse to retain a memory.
There are still big gaps in the story of how memory works, many of which await filling with the chemical details. How, for example, is memory recalled once it has been stored? “This is a deep problem whose analysis is just beginning,” says neuroscientist and Nobel laureate Eric Kandel of Columbia University.
Coming to grips with the chemistry of memory offers the enticing and controversial prospect of pharmacological enhancement. Some memory-boosting substances are already known, including sex hormones and synthetic chemicals that act on receptors for nicotine, glutamate, serotonin and other neurotransmitters. In fact, according to neurobiologist Gary Lynch of the University of California, Irvine, the complex sequence of steps leading to long-term learning and memory means that there are many potential targets for such memory drugs.
5 How Many Elements Exist? The periodic tables that adorn the walls of classrooms have to be constantly revised, because the number of elements keeps growing. Using particle accelerators to crash atomic nuclei together, scientists can create new “superheavy” elements, which have more protons and neutrons in their nuclei than do the 92 or so elements found in nature. These engorged nuclei are not very stable—they decay radioactively, often within a tiny fraction of a second. But while they exist, the new synthetic elements such as seaborgium (element 106) and hassium (element 108) are like any other insofar as they have well-defined chemical properties. In dazzling experiments, researchers have investigated some of those properties in a handful of elusive seaborgium and hassium atoms during the brief instants before they fell apart.
Such studies probe not just the physical but also the conceptual limits of the periodic table: Do superheavy elements continue to display the trends and regularities in chemical behavior that make the table periodic in the first place? The answer is that some do, and some do not. In particular, such massive nuclei hold on to the atoms’ innermost electrons so tightly that the electrons move at close to the speed of light. Then the effects of special relativity increase the electrons’ mass and may play havoc with the quantum energy states on which their chemistry—and thus the table’s periodicity—depends.
Because nuclei are thought to be stabilized by particular “magic numbers” of protons and neutrons, some researchers hope to find what they call the island of stability, a region a little beyond the current capabilities of element synthesis in which superheavies live longer. Yet is there any fundamental limit to their size? A simple calculation suggests that relativity prohibits electrons from being bound to nuclei of more than 137 protons. More sophisticated calculations defy that limit. “The periodic system will not end at 137; in fact, it will never end,” insists nuclear physicist Walter Greiner of the Johann Wolfgang Goethe University Frankfurt in Germany. The experimental test of that claim remains a long way off.
6 Can Computers Be Made Out of Carbon? Computer chips made out of graphene—a web of carbon atoms—could potentially be faster and more powerful than silicon-based ones. The discovery of graphene garnered the 2010 Nobel Prize in Physics, but the success of this and other forms of carbon nanotechnology might ultimately depend on chemists’ ability to create structures with atomic precision.
The discovery of buckyballs—hollow, cagelike molecules made entirely of carbon atoms—in 1985 was the start of something literally much bigger. Six years later tubes of carbon atoms arranged in a chicken wire–shaped, hexagonal pattern like that in the carbon sheets of graphite made their debut. Being hollow, extremely strong and stiff, and electrically conducting, these carbon nanotubes promised applications ranging from high-strength carbon composites to tiny wires and electronic devices, miniature molecular capsules, and water-filtration membranes.
For all their promise, carbon nanotubes have not resulted in a lot of commercial applications. For instance, researchers have not been able to solve the problem of how to connect tubes into complicated electronic circuits. More recently, graphite has moved to center stage because of the discovery that it can be separated into individual chicken wire–like sheets, called graphene, that could supply the fabric for ultraminiaturized, cheap and robust electronic circuitry. The hope is that the computer industry can use narrow ribbons and networks of graphene, made to measure with atomic precision, to build chips with better performance than silicon-based ones.
“Graphene can be patterned so that the interconnect and placement problems of carbon nanotubes are overcome,” says carbon specialist Walt de Heer of the Georgia Institute of Technology. Methods such as etching, however, are too crude for patterning graphene circuits down to the single atom, de Heer points out, and as a result, he fears that graphene technology currently owes more to hype than hard science. Using the techniques of organic chemistry to build up graphene circuits from the bottom up—linking together “polyaromatic” molecules containing several hexagonal carbon rings, like little fragments of a graphene sheet—might be the key to such precise atomic-scale engineering and thus to unlocking the future of graphene electronics.
7 How Do We Tap More Solar Energy? With every sunrise comes a reminder that we currently tap only a pitiful fraction of the vast clean-energy resource that is the sun. The main problem is cost: the expense of conventional photovoltaic panels made of silicon still restricts their use. Yet life on Earth, almost all of which is ultimately solar-powered by photosynthesis, shows that solar cells do not have to be terribly efficient if, like leaves, they can be made abundantly and cheaply enough.
“One of the holy grails of solar-energy research is using sunlight to produce fuels,” says Devens Gust of Arizona State University. The easiest way to make fuel from solar energy is to split water to produce hydrogen and oxygen gas. Nathan S. Lewis and his collaborators at Caltech are developing an artificial leaf that would do just that using silicon nanowires.
Earlier this year Daniel Nocera of the Massachusetts Institute of Technology and his co-workers unveiled a silicon-based membrane in which a cobalt-based photocatalyst does the water splitting. Nocera estimates that a gallon of water would provide enough fuel to power a home in developing countries for a day. “Our goal is to make each home its own power station,” he says.
Splitting water with catalysts is still tough. “Cobalt catalysts such as the one that Nocera uses and newly discovered catalysts based on other common metals are promising,” Gust says, but no one has yet found an ideal inexpensive catalyst. “We don’t know how the natural photosynthetic catalyst, which is based on four manganese atoms and a calcium atom, works,” Gust adds.
Gust and his colleagues have been looking into making molecular assemblies for artificial photosynthesis that more closely mimic their biological inspiration, and his team has managed to synthesize some of the elements that could go into such an assembly. Still, a lot more work is needed on this front. Organic molecules such as the ones nature uses tend to break down quickly. Whereas plants continually produce new proteins to replace broken ones, artificial leaves do not (yet) have the full chemical-synthesis machinery of a living cell at their disposal.
8 What Is the Best Way to Make Biofuels? Instead of making fuels by capturing the rays of the sun, how about we let plants store the sun’s energy for us and then turn plant matter into fuels? Biofuels such as ethanol made from corn and biodiesel made from seeds have already found a place in the energy markets, but they threaten to displace food crops, particularly in developing countries where selling biofuels abroad can be more lucrative than feeding people at home. The numbers are daunting: meeting current oil demand would mean requisitioning huge areas of arable land.
Turning food into energy, then, may not be the best approach. One answer could be to exploit other, less vital forms of biomass. The U.S. produces enough agricultural and forest residue to supply nearly a third of the annual consumption of gasoline and diesel for transportation.
Converting this low-grade biomass into fuel requires breaking down hardy molecules such as lignin and cellulose, the main building blocks of plants. Chemists already know how to do that, but the existing methods tend to be too expensive, inefficient or difficult to scale up for the enormous quantities of fuel that the economy needs.
One of the challenges of breaking down lignin—cracking open the carbon-oxygen bonds that link “aromatic,” or benzene-type, rings of carbon atoms—was recently met by John Hartwig and Alexey Sergeev, both at the University of Illinois. They found a nickel-based catalyst able to do it. Hartwig points out that if biomass is to supply nonfossil-fuel chemical feedstocks as well as fuels, chemists will also need to extract aromatic compounds (those having a backbone of aromatic rings) from it. Lignin is the only major potential source of such aromatics in biomass.
To be practical, such conversion of biomass will, moreover, need to work with mostly solid biomass and convert it into liquid fuels for easy transportation along pipelines. Liquefaction would need to happen on-site, where the plant is harvested. One of the difficulties for catalytic conversion is the extreme impurity of the raw material—classical chemical synthesis does not usually deal with messy materials such as wood. “There’s no consensus on how all this will be done in the end,” Hartwig says. What is certain is that an awful lot of any solution lies with the chemistry, especially with finding the right catalysts. “Almost every industrial reaction on a large scale has a catalyst associated” with it, Hartwig points out.
9 Can We Devise New Ways to Create Drugs? The core business of chemistry is a practical, creative one: making molecules, a key to creating everything from new materials to new antibiotics that can outstrip the rise of resistant bacteria.
In the 1990s one big hope was combinatorial chemistry, in which thousands of new molecules are made by a random assembly of building blocks and then screened to identify those that do a job well. Once hailed as the future of medicinal chemistry, “combi-chem” fell from favor because it produced little of any use.
But combinatorial chemistry could enjoy a brighter second phase. It seems likely to work only if you can make a wide enough range of molecules and find good ways of picking out the minuscule amounts of successful ones. Biotechnology might help here—for example, each molecule could be linked to a DNA-based “bar code” that both identifies it and aids its extraction. Or researchers can progressively refine the library of candidate molecules by using a kind of Darwinian evolution in the test tube. They can encode potential protein-based drug molecules in DNA and then use error-prone replication to generate new variants of the successful ones, thereby finding improvements with each round of replication and selection.
Other new techniques draw on nature’s mastery at uniting molecular fragments in prescribed arrangements. Proteins, for example, have a precise sequence of amino acids because that sequence is spelled out by the genes that encode the proteins. Using this model, future chemists might program molecules to assemble autonomously. The approach has the advantage of being “green” in that it reduces the unwanted by-products typical of traditional chemical manufacturing and the associated waste of energy and materials.
David Liu of Harvard University and his co-workers are pursuing this approach. They tagged the building blocks with short DNA strands that program the linker’s structure. They also created a molecule that walks along that DNA, reading its codes and sequentially attaching small molecules to the building block to make the linker—a process analogous to protein synthesis in cells. Liu’s method could be a handy way to tailor new drugs. “Many molecular life scientists believe that macromolecules will play an increasingly central, if not dominant, role in the future of therapeutics,” Liu says.
10 Can We Continuously Monitor Our Own Chemistry? Increasingly, chemists do not want to just make molecules but also to communicate with them: to make chemistry an information technology that will interface with anything from living cells to conventional computers and fiber-optic telecommunications.
In part, it is an old idea: biosensors in which chemical reactions are used to report on concentrations of glucose in the blood date back to the 1960s, although only recently has their use for monitoring diabetes been cheap, portable and widespread. Chemical sensing could have countless applications—to detect contaminants in food and water at very low concentrations, for instance, or to monitor pollutants and trace gases present in the atmosphere. Faster, cheaper, more sensitive and more ubiquitous chemical sensing would yield progress in all of those areas.
It is in biomedicine, though, that new kinds of chemical sensors would have the most dramatic potential. For instance, some of the products of cancer genes circulate in the bloodstream long before the condition becomes apparent to regular clinical tests. Detecting these chemicals early might make prognoses more timely and accurate. Rapid genomic profiling would enable drug regimens to be tailored to individual patients, thereby reducing risks of side effects and allowing some medicines to be used that today are hampered by their dangers to a genetic minority.
Some chemists foresee continuous, unobtrusive monitoring of all manner of biochemical markers of health and disease, perhaps providing real-time information to surgeons during operations or to automated systems for delivering remedial drug treatments. This futuristic vision depends on developing chemical methods for selectively sensing particular substances and signaling about them even when the targets occur in only very low concentrations.
Read the articles in our Special Year of Chemistry Celebration
» Atom Power: 2011 is the International Year of Chemistry—a well-deserved celebration of that science's profound power » The Scent of Your Thoughts: Although we are usually unaware of it, we communicate through chemical signals just as much as birds and bees do
Kraemer Family Library
Chemistry research: review books & practice problems.
- Review Books & Practice Problems
- Look Up Chemical Properties
- Find Journal Articles & Proceedings
- Writing & Citing
General Chemistry
Schaum's Outlines provide overviews of a topic and include hundreds of solved problems that you can use to practice your skills.
Overviews and practice problems for general chemistry can often be found with call numbers QD42 . Alternative textbook are in QD31 .
Here are a few examples of the books you can find in that area:
Organic Chemistry
Overviews and practice problems for organic chemistry can generally be found with call numbers QD256 - 257 . Alternative organic chemistry textbook are in QD251 .
Test Preparation
- Preparing for you ACS Examination in Physical Chemistry Call Number: QD456 .H65 2009 ON RESERVE - Bring this call number to the Main Circulation desk
Looking for MCAT study guides in other subject areas? Check out the Biology Research guide for a full list.
- << Previous: Home
- Next: Look Up Chemical Properties >>
- Last Updated: Feb 2, 2024 1:47 PM
- URL: https://libguides.uccs.edu/chem
Need Research Assistance? Get Help
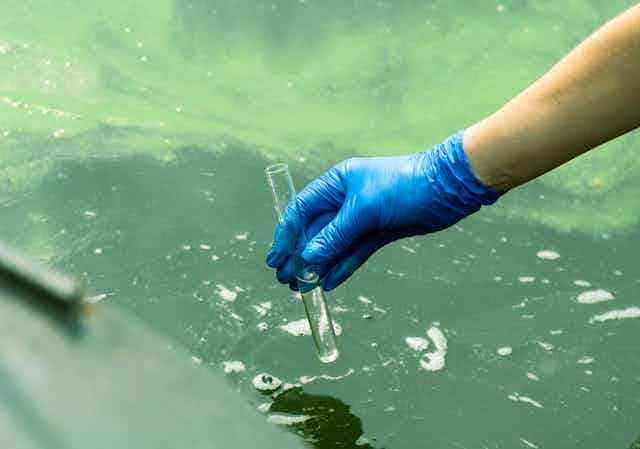
Five chemistry research projects that you can get involved in
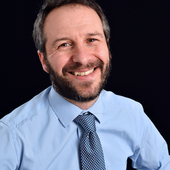
Professor of Science Communication and Chemistry, University of Hull
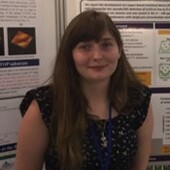
Lecturer of Analytical Chemistry, University of Hull
Disclosure statement
Mark Lorch receives funding from European Regional Development Fund through the Interreg VB North Sea Region Programme
[email protected] receives funding from European Regional Development Fund through the Interreg VB North Sea Region Programme.
University of Hull provides funding as a member of The Conversation UK.
View all partners
Sometimes the most powerful tool in research is people spending a few minutes to record their observations while going about their daily lives. An early example of this sort of “citizen science” is the annual garden bird watch in the UK, which has been running since 1978 and is organised by the nature conservation charity, RSBP . All you need do to take part is spending an hour watching the wildlife in you garden or local park.
Today, citizen science projects are increasingly popular, with people surveying and monitoring everything from weather events , invasive plant species and ladybirds to planets orbiting stars other than our Sun.
As the citizen science field has developed, boundaries have blurred and scientists have begun involving citizens as more active researchers – carrying out important experiments, collecting environmental measurements and generating data.
Here are five just such projects with a distinctly chemical theme.
Our new paper, published in PLOS One , presents the results of such a project, RiverDip , which enables and encourages citizens to monitor the chemical health of their local waterways.
This involves monitoring phosphates and nitrates – essential nutrients, making up the basis of agricultural fertilisers. But if they run off fields and into waterways they cause significant problems.
The fertilisers encourage rapid growth of algae and weeds, which form dense green mats on the surface of waterways. These block out the light to other plants. What’s more, later, when they rot they use up some of the dissolved oxygen in the water, resulting in deoxygenation that harms other aquatic plants and animals.
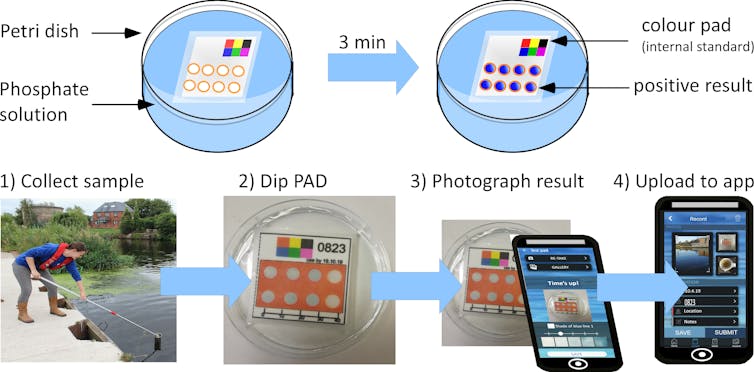
RiverDip was developed as part of the EU-funded Sullied Sediments project as a means to allow citizens to monitor the phosphate levels in waterways. We provided interested folk with paper-based sensors that change colour in the presence of phosphates. The measurement takes just three minutes. After it’s done, volunteers upload their results via a bespoke mobile app.
Together we have collected hundreds of measurements and begun to map phosphate levels across the Europe’s North Sea Region, consisting of countries including the Scandinavian nations, England, the Netherlands and Germany. Having lots of measurements from different seasons will help us to understand how nutrient levels change over time, and we are currently looking for interested volunteer groups to continue this project.
The Big Compost experiment
If you like rummaging in the garden, this one is for you. Lots of packaging is now labelled as biodegradable or compostable, but what does this really mean and do these products really break down in a domestic compost bin? The Big Compost experiment investigates new ways of reducing plastic waste, asking participants to check how well biodegradable and compostable packaging breaks down.
You can help answer these questions by simply bagging up materials that claim to be compostable (such as some tea bags, carrier bags and disposable cups), placing them in your compost heap and then observing what happens. You can record your results via the experiment’s home page .
Fold-at-home
If you fancy something easier and less messy, there are some great projects which you can contribute to from the comfort of your sofa.
Proteins are the molecular machines that govern all the chemical processes and interactions that make up a living organism. And like any machine (be it a proteins or a motor car), they help to understand how all the parts fit together when designing modifications and upgrades. So understanding proteins’ incredibly complex structures, how they interact with each other and potential drugs provides pharmaceutical developers with critical information that allows them to design more effective therapeutics. But modelling this requires vast amounts of computing power. One approach would therefore be to use vast amounts of money to build a computer dedicated to solving this problem.
But scientists have realised that, alternatively, you could ask people to contribute spare computing power of their home PCs to form a giant global supercomputer. All you need do is install the Fold-at-home software on your computer and when you nip off to make a cup of tea or plug into the television, your computer gets to work on folding proteins, which could lead to the development of COVID drugs or cancer therapies.
If puzzles and computer games are more your cup of tea, you may enjoy Fold-it . This project attempts to predict the structure of a protein, but this time it needs a bit more human input. It takes advantage of people’s puzzle-solving intuitions when playing games competitively and challenges them to fold the best proteins.
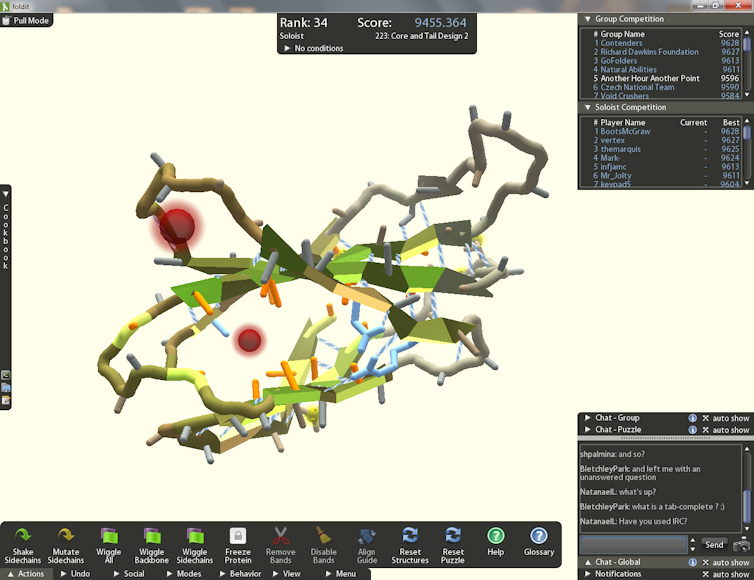
This information helps researchers understand if human pattern recognition and puzzle solving abilities are better than current computer programs. Such information could be used to develop new computer strategies to predict protein structures even faster. This is really helpful as understanding how proteins fold and interact enables scientists to develop new proteins to help combat diseases such as Alzheimer’s and HIV/AIDS.
Sensor community
The sensor community project aims to build a network of small sensors to collect and openly share environmental data such as the nitrogen dioxide air pollution generated by internal combustion engines and burning of fossil fuels.
Currently, the community has constructed and deployed nearly 14,000 active sensors in 69 countries, all of which are returning data in real time. To take part in this project, you build sensors using kits developed by the researchers and place them somewhere. The project has different communities that focus on different aspects of environmental pollution (including noise).
Getting involved in these kind of citizen sciences projects can be a great way to have a positive impact on the world, collecting large volumes of data that enable us to understand our impact on the planet.
- Citizen science

Data Manager

Research Support Officer

Director, Social Policy

Head, School of Psychology

Senior Research Fellow - Women's Health Services
Advertisement
Comprehending complex chemistry problems in a structured and enjoyable manner: A concept mapping-based contextual gaming approach
- Published: 14 March 2024
Cite this article
- Gwo-Jen Hwang 1 , 2 , 3 ,
- Wen-Hua Chuang 2 , 4 &
- Lu-Ho Hsia ORCID: orcid.org/0000-0003-1081-5484 5
215 Accesses
Explore all metrics
Many scientific concepts and theorems are often abstract and challenging to relate to real-life problems, making it difficult for students to grasp them. Therefore, some researchers have attempted to enhance students’ understanding by employing a contextual learning approach, which allows students to apply scientific knowledge to real situations in their daily lives. The aim is to improve students’ learning experiences by moving away from rote memorization. However, if a contextual gaming approach is offered without encouraging deep reflection, students may focus solely on the game itself and overlook the importance of fully understanding the knowledge and contemplating the meaningful relationships between scientific concepts. To address this issue, for this study we developed a Concept Mapping-based Digital Game-Based Learning for Complex Chemistry Problems (short for CM-DGBL-CCP) learning system to assist students in understanding complex chemistry problems. To verify the effects of the proposed approach, the experiment was conducted in a secondary school with two groups. The experimental group with 49 students adopted the CM-DGBL-CCP learning model, while the control group with 56 students utilized the traditional digital game-based learning for complex chemistry problems (T-DGBL-CCP) learning model. The experimental results revealed that there were no significant differences between the two groups of students in terms of learning achievement and cognitive load. However, the experimental group students outperformed the control group in areas such as problem-solving tendency, scientific self-efficacy, scientific learning strategies, and the ability to use deep-level strategies to solve problems.
This is a preview of subscription content, log in via an institution to check access.
Access this article
Price includes VAT (Russian Federation)
Instant access to the full article PDF.
Rent this article via DeepDyve
Institutional subscriptions

Similar content being viewed by others
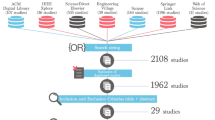
Tailored gamification in education: A literature review and future agenda
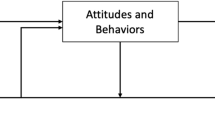
The impact of gamification in educational settings on student learning outcomes: a meta-analysis
Harnessing the Power of AI to Create Intelligent Tutoring Systems for Enhanced Classroom Experience and Improved Learning Outcomes
Data availability.
The data and materials are available upon request to the corresponding author.
Code availability
Not applicable.
Abd El-Hay, S. A., Mezayen, E., S. E., & Ahmed, R. E. (2018). Effect of concept mapping on problem solving skills, competence in clinical setting and knowledge among undergraduate nursing students. Journal of Nursing Education and Practice , 8 (8), 34–46.
Article Google Scholar
Acquah, E. O., & Katz, H. T. (2020). Digital game-based L2 learning outcomes for primary through high-school students: A systematic literature review. Computers & Education , 143 , 103667.
Bakeman, R., & Gottman, J. M. (1997). Observing interaction: An introduction to sequential analysis (2nd ed.). Cambridge University Press.
Barzilai, S., & Blau, I. (2014). Scaffolding game-based learning: Impact on learning achievements, perceived learning, and game experiences. Computers & Education , 70 , 65–79.
Bilik, Ö., Kankaya, E. A., & Deveci, Z. (2020). Effects of web-based concept mapping education on students’ concept mapping and critical thinking skills: A double blind, randomized, controlled study. Nurse Education Today , 86 , 104312.
Article PubMed Google Scholar
Brezovszky, B., McMullen, J., Veermans, K., Hannula-Sormunen, M. M., Rodríguez-Aflecht, G., Pongsakdi, N., Laakkonen, E., & Lehtinen, E. (2018). Effects of a mathematics game-based learning environment on primary school students’ adaptive number knowledge. Computers & Education , 128 , 63–74.
Brown, J. S., Collins, A., & Duguid, P. (1989). Situated cognition and the culture of learning. Educational Researcher , 18 (1), 32–42.
Chang, C. C., Hwang, G. J., & Tu, Y. F. (2022). Roles, applications, and trends of concept map-supported learning: A systematic review and bibliometric analysis of publications from 1992 to 2020 in selected educational technology journals. Interactive Learning Environments . https://doi.org/10.1080/10494820.2022.2027457 .
Charsky, D., & Ressler, W. (2011). Games are made for fun: Lessons on the effects of concept maps in the classroom use of computer games. Computers & Education , 56 (3), 604–615.
Cheng, M. T., She, H. C., & Annetta, L. A. (2015). Game immersion experience: Its hierarchical structure and impact on game-based science learning. Journal of Computer Assisted Learning , 31 (3), 232–253.
Chu, H. C., Yang, K. H., & Chen, J. H. (2014). A time sequence-oriented concept map approach to developing educational computer games for history courses. Interactive Learning Environments , 23 (2), 212–229.
Chu, H. C., Wang, C. C., & Wang, L. (2019). Impacts of concept map-based collaborative mobile gaming on English grammar learning performance and behaviors. Journal of Educational Technology & Society , 22 (2), 86–100.
Google Scholar
Clark, D. B., Nelson, B. C., Chang, H. Y., Martinez-Garza, M., Slack, K., & D’Angelo, C. M. (2011). Exploring newtonian mechanics in a conceptually-integrated digital game: Comparison of learning and affective outcomes for students in Taiwan and the United States. Computers & Education , 57 (3), 2178–2195.
Collins, A., Brown, J. S., & Newman, S. E. (1988). Cognitive apprenticeship: Teaching the craft of reading, writing, and mathematics. Thinking: The Journal of Philosophy for Children , 8 (1), 2–10.
Connolly, T. M., Stansfield, M., & Hainey, T. (2007). An application of games-based learning within software engineering. British Journal of Educational Technology , 38 (3), 416–428.
D’Souza, A. C. D., & Clare, A. C. (2018). Effect of situated learning model on critical problem solving skills among higher secondary pupils. i-manager’s Journal on School Educational Technology , 14 (1), 27–34.
Fu, Q. K., Lin, C. J., Hwang, G. J., & Zhang, L. (2019). Impacts of a mind mapping-based contextual gaming approach on EFL students’ writing performance, learning perceptions and generative uses in an English course. Computers& Education , 137 , 59–77.
Hodges, G. W., Wang, L., Lee, J., Cohen, A., & Jang, Y. (2018). An exploratory study of blending the virtual world and the laboratory experience in secondary chemistry classrooms. Computers & Education , 122 , 179–193.
Hwang, G. J., & Wang, S. Y. (2016). Single loop or double loop learning: English vocabulary learning performance and behavior of students in situated computer games with different guiding strategies. Computers & Education , 102 , 188–201.
Hwang, G. J., Yang, L. H., & Wang, S. Y. (2013). A concept map-embedded educational computer game for improving students’ learning performance in natural science courses. Computers & Education , 69 , 121–130.
Hwang, G. J., Lee, H. Y., & Chen, C. H. (2019). Lessons learned from integrating concept mapping and gaming approaches into learning scenarios using mobile devices: Analysis of an activity for a geology course. International Journal of Mobile Learning and Organisation , 13 (3), 286–308.
Janakiraman, S., Watson, S. L., Watson, W. R., & Newby, T. (2021). Effectiveness of digital games in producing environmentally friendly attitudes and behaviors: A mixed methods study. Computers & Education , 160 , 104043.
Kao, G. Y. M., Chiang, C. H., & Sun, C. T. (2017). Customizing scaffolds for game-based learning in physics: Impacts on knowledge acquisition and game design creativity. Computers & Education , 113 , 294–312.
Ke, F. (2008). Computer games application within alternative classroom goal structures: Cognitive, metacognitive, and affective evaluation. Educational Technology Research & Development , 56 (5), 539–556.
Krath, J., Schürmann, L., & Von Korflesch, H. F. (2021). Revealing the theoretical basis of gamification: A systematic review and analysis of theory in research on gamification, serious games and game-based learning. Computers in Human Behavior , 125 , 106963.
Lai, C. L., & Hwang, G. J. (2014). Effects of mobile learning time on students’ conception of collaboration, communication, complex problem-solving, meta-cognitive awareness and creativity. International Journal of Mobile Learning and Organisation , 8 (3), 276–291.
Article MathSciNet Google Scholar
Lee, J., & Choi, H. (2017). What affects learner’s higher-order thinking in technology-enhanced learning environments? The effects of learner factors. Computers & Education , 115 , 143–152.
Lee, M. H., Johanson, R. E., & Tsai, C. C. (2008). Exploring Taiwanese high school students’ conceptions of and approaches to learning science through a structural equation modeling analysis. Science Education , 92 (2), 191–220.
Article ADS Google Scholar
Novak, J. D. (2002). Meaningful learning: The essential factor for conceptual change in limited or appropriate propositional hierarchies (LIPHs) leading to empowerment of learners. Science Education , 86 (4), 548–571.
Osborne, J., & Collins, J. (2001). Pupils’ views of the role and value of the science curriculum: A focus group study. International Journal of Science Education , 23 (5), 441–467.
Pankratius, W. J. (1990). Building an organized knowledge base: Concept mapping and achievement in secondary school physics. Journal of Research in Science Teaching , 27 (4), 315–333.
Pintrich, P. R., Smith, D. A. F., Garcia, T., & McKeachie, W. J. (1991). A manual for the use of the motivated strategies for learning questionnaire (MSLQ) . MI: National Center for Research to Improve Postsecondary Teaching and Learning. (ERIC Document Reproduction Service No. ED 338122).
Quintana, C., Reiser, B. J., Davis, E. A., Krajcik, J., Fretz, E., Duncan, R. G., et al. (2004). A scaffolding design framework for software to support science inquiry. Journal of the Learning Sciences , 13 (3), 337–386.
Roshangar, F., Azar, E. F., Sarbakhsh, P., & Azarmi, R. (2020). The effect of case-based learning with or without conceptual mapping method on critical thinking and academic self-efficacy of nursing students. Journal of Biochemical Technology , 11 (1), 37–44.
Scherer, R., & Tiemann, R. (2012). Factors of problem-solving competency in a virtual chemistry environment: The role of metacognitive knowledge about strategies. Computers & Education , 59 (4), 1199–1214.
Schroeder, N. L., Nesbit, J. C., Anguiano, C. J., & Adesope, O. O. (2018). Studying and constructing concept maps: A meta-analysis. Educational Psychology Review , 30 , 431–455.
Shaw, R. S. (2010). A study of learning performance of e-learning materials design with knowledge maps. Computers & Education , 54 (1), 253–264.
Sirhan, G. (2007). Learning difficulties in chemistry: An overview. Journal of Turkish Science Education , 4 (2), 2–20.
Srisawasdi, N., & Panjaburee, P. (2019). Implementation of game-transformed inquiry-based learning to promote the understanding of and motivation to learn chemistry. Journal of Science Education and Technology , 28 (2), 152–164.
Stenberdt, V. A., & Makransky, G. (2023). Mastery experiences in immersive virtual reality promote pro-environmental waste-sorting behavior. Computers & Education , 198 , 104760.
Sung, H. Y., Hwang, G. J., Lin, C. J., & Hong, T. W. (2017). Experiencing the analects of confucius: An experiential game-based learning approach to promoting students’ motivation and conception of learning. Computers & Education , 110 , 143–153.
Sweller, J., Van Merriënboer, J. J. G., & Paas, F. G. W. C. (1998). Cognitive architecture and instructional design. Educational Psychology Review , 10 (3), 251–297.
Tay, J., Goh, Y. M., Safiena, S., & Bound, H. (2022). Designing digital game-based learning for professional upskilling: A systematic literature review. Computers & Education , 104518.
Tiemann, R., & Annaggar, A. (2020). A framework for the theory-driven design of digital learning environments (FDDLEs) using the example of problem-solving in chemistry education. Interactive Learning Environments . https://doi.org/10.1080/10494820.2020.1826981 .
Wen, C. T., Chang, C. J., Chang, M. H., Chiang, S. H. F., Liu, C. C., Hwang, F. K., & Tsai, C. C. (2018). The learning analytics of model-based learning facilitated by a problem-solving simulation game. Instructional Science , 46 (6), 847–867.
Wouters, P., van Nimwegen, C., van Oostendorp, H., & van der Spek, E. D. (2013). A meta-analysis of the cognitive and motivational effects of serious games. Journal of Educational Psychology , 105 (2), 249–265.
Yang, Y. T. C. (2012). Building virtual cities, inspiring intelligent citizens: Digital games for developing students’ problem solving and learning motivation. Computers & Education , 59 (2), 365–377.
Zhao, L., Liu, X., Wang, C., & Su, Y. S. (2022). Effect of different mind mapping approaches on primary school students’ computational thinking skills during visual programming learning. Computers & Education , 181 , 104445.
Download references
This study is supported in part by the National Science and Technology Council of Taiwan under contract numbers NSTC 112-2410-H-011-012-MY3 and NSTC 112-2410-H-167-002-MY2. The study is also supported by the “Empower Vocational Education Research Center” of National Taiwan University of Science and Technology (NTUST) from the Featured Areas Research Center Program within the framework of the Higher Education Sprout Project by the Ministry of Education (MOE) in Taiwan.
Author information
Authors and affiliations.
Graduate Institute of Educational Information and Measurement, National Taichung University of Education, Taichung City, Taiwan
Gwo-Jen Hwang
Graduate Institute of Digital Learning and Education, National Taiwan University of Science and Technology, Taipei City, Taiwan
Gwo-Jen Hwang & Wen-Hua Chuang
Yuan Ze University, Taoyuan City, Taiwan
Empower Vocational Education Research Center, National Taiwan University of Science and Technology, Taipei City, Taiwan
Wen-Hua Chuang
Office of Physical Education, National Chin-Yi University of Technology, Taichung, Taiwan
You can also search for this author in PubMed Google Scholar
Contributions
All authors contributed to the study conception and design. Material preparation, data collection and analysis were performed by W.C. Project administration were performed by G.H. Methodology and supervision were performed G.H and L.H. The first draft of the manuscript was written by W.C and L.H. All authors commented on previous versions of the manuscript. All authors read and approved the final manuscript.
Corresponding author
Correspondence to Lu-Ho Hsia .
Ethics declarations
Ethical approval.
The ethical requirements for research in this selected university were followed.
Consent to participate
The participants all agreed to take part in this study.
Consent for publication
The publication of this study has been approved by all authors.
Competing interests
There is no potential conflict of interest in this study.
Additional information
Publisher’s note.
Springer Nature remains neutral with regard to jurisdictional claims in published maps and institutional affiliations.
Rights and permissions
Springer Nature or its licensor (e.g. a society or other partner) holds exclusive rights to this article under a publishing agreement with the author(s) or other rightsholder(s); author self-archiving of the accepted manuscript version of this article is solely governed by the terms of such publishing agreement and applicable law.
Reprints and permissions
About this article
Hwang, GJ., Chuang, WH. & Hsia, LH. Comprehending complex chemistry problems in a structured and enjoyable manner: A concept mapping-based contextual gaming approach. Educ Inf Technol (2024). https://doi.org/10.1007/s10639-024-12615-0
Download citation
Received : 17 November 2023
Accepted : 04 March 2024
Published : 14 March 2024
DOI : https://doi.org/10.1007/s10639-024-12615-0
Share this article
Anyone you share the following link with will be able to read this content:
Sorry, a shareable link is not currently available for this article.
Provided by the Springer Nature SharedIt content-sharing initiative
- Contextual learning
- Game-based learning
- Concept map
- Problem solving
- Chemistry courses
- Find a journal
- Publish with us
- Track your research

Problems and Problem Solving in Chemistry Education: Analysing Data, Looking for Patterns and Making Deductions
Problem solving is central to the teaching and learning of chemistry at secondary, tertiary and post-tertiary levels of education, opening to students and professional chemists alike a whole new world for analysing data, looking for patterns and making deductions. As an important higher-order thinking skill, problem solving also constitutes a major research field in science education. Relevant education research is an ongoing process, with recent developments occurring not only in the area of quantitative/computational problems, but also in qualitative problem solving.
The following situations are considered, some general, others with a focus on specific areas of chemistry: quantitative problems, qualitative reasoning, metacognition and resource activation, deconstructing the problem-solving process, an overview of the working memory hypothesis, reasoning with the electron-pushing formalism, scaffolding organic synthesis skills, spectroscopy for structural characterization in organic chemistry, enzyme kinetics, problem solving in the academic chemistry laboratory, chemistry problem-solving in context, team-based/active learning, technology for molecular representations, IR spectra simulation, and computational quantum chemistry tools. The book concludes with methodological and epistemological issues in problem solving research and other perspectives in problem solving in chemistry.
With a foreword by George Bodner.
- Cite Icon Cite
Problems and Problem Solving in Chemistry Education: Analysing Data, Looking for Patterns and Making Deductions, The Royal Society of Chemistry, 2021.
Download citation file:
- Ris (Zotero)
- Reference Manager
Digital access
Print format, table of contents.
- Front Matter
- Acknowledgments
- Author Biographies
- Chapter 1: Introduction − The Many Types and Kinds of Chemistry Problems p1-14 By Georgios Tsaparlis Georgios Tsaparlis University of Ioannina, Department of Chemistry Ioannina Greece [email protected] Search for other works by this author on: This Site PubMed Google Scholar Abstract Open the PDF Link PDF for Chapter 1: Introduction − The Many Types and Kinds of Chemistry Problems in another window
- Chapter 2: Qualitative Reasoning in Problem-solving in Chemistry p15-37 By Vicente Talanquer Vicente Talanquer Department of Chemistry and Biochemistry, University of Arizona Tucson AZ 85721 USA [email protected] Search for other works by this author on: This Site PubMed Google Scholar Abstract Open the PDF Link PDF for Chapter 2: Qualitative Reasoning in Problem-solving in Chemistry in another window
- Chapter 3: Scaffolding Metacognition and Resource Activation During Problem Solving: A Continuum Perspective p38-67 By Nicole Graulich ; Nicole Graulich Justus-Liebig-Universität Gießen Germany Search for other works by this author on: This Site PubMed Google Scholar Axel Langner ; Axel Langner Justus-Liebig-Universität Gießen Germany Search for other works by this author on: This Site PubMed Google Scholar Kimberly Vo ; Kimberly Vo Monash University Australia [email protected] Search for other works by this author on: This Site PubMed Google Scholar Elizabeth Yuriev Elizabeth Yuriev Monash University Australia [email protected] Search for other works by this author on: This Site PubMed Google Scholar Abstract Open the PDF Link PDF for Chapter 3: Scaffolding Metacognition and Resource Activation During Problem Solving: A Continuum Perspective in another window
- Chapter 4: Deconstructing the Problem-solving Process: Beneath Assigned Points and Beyond Traditional Assessment p68-92 By Ozcan Gulacar ; Ozcan Gulacar University of California, Davis, Department of Chemistry One Shields Avenue Davis CA 95616 USA [email protected] Search for other works by this author on: This Site PubMed Google Scholar Charlie Cox ; Charlie Cox Duke University, Department of Chemistry Box 90346, 128 Science Drive Durham NC 27708-0346 USA Search for other works by this author on: This Site PubMed Google Scholar Herb Fynewever Herb Fynewever Calvin University, Department of Chemistry 3201 Burton SE Grand Rapids MI 49546 USA Search for other works by this author on: This Site PubMed Google Scholar Abstract Open the PDF Link PDF for Chapter 4: Deconstructing the Problem-solving Process: Beneath Assigned Points and Beyond Traditional Assessment in another window
- Chapter 5: It Depends on the Problem and on the Solver: An Overview of the Working Memory Overload Hypothesis, Its Applicability and Its Limitations p93-126 By Georgios Tsaparlis Georgios Tsaparlis University of Ioannina, Department of Chemistry Ioannina Greece [email protected] Search for other works by this author on: This Site PubMed Google Scholar Abstract Open the PDF Link PDF for Chapter 5: It Depends on the Problem and on the Solver: An Overview of the Working Memory Overload Hypothesis, Its Applicability and Its Limitations in another window
- Chapter 6: Mechanistic Reasoning Using the Electron-pushing Formalism p127-144 By Gautam Bhattacharyya Gautam Bhattacharyya Missouri State University, Department of Chemistry 901 South National Avenue Springfield MO 65897 USA [email protected] Search for other works by this author on: This Site PubMed Google Scholar Abstract Open the PDF Link PDF for Chapter 6: Mechanistic Reasoning Using the Electron-pushing Formalism in another window
- Chapter 7: Scaffolding Synthesis Skills in Organic Chemistry p145-165 By Alison B. Flynn Alison B. Flynn Department of Chemistry and Biomolecular Sciences, University of Ottawa 10 Marie Curie Ottawa Ontario K1N 6N5 Canada [email protected] Search for other works by this author on: This Site PubMed Google Scholar Abstract Open the PDF Link PDF for Chapter 7: Scaffolding Synthesis Skills in Organic Chemistry in another window
- Chapter 8: Problem Solving Using NMR and IR Spectroscopy for Structural Characterization in Organic Chemistry p166-198 By Megan C. Connor ; Megan C. Connor Department of Chemistry, University of Michigan Ann Arbor Michigan USA [email protected] Search for other works by this author on: This Site PubMed Google Scholar Ginger V. Shultz Ginger V. Shultz Department of Chemistry, University of Michigan Ann Arbor Michigan USA [email protected] Search for other works by this author on: This Site PubMed Google Scholar Abstract Open the PDF Link PDF for Chapter 8: Problem Solving Using NMR and IR Spectroscopy for Structural Characterization in Organic Chemistry in another window
- Chapter 9: Assessing System Ontology in Biochemistry: Analysis of Students’ Problem Solving in Enzyme Kinetics p199-216 By Jon-Marc G. Rodriguez ; Jon-Marc G. Rodriguez University of Iowa, Department of Chemistry E355 Chemistry Building Iowa City Iowa 52242-1294 USA [email protected] Search for other works by this author on: This Site PubMed Google Scholar Sven J. Philips ; Sven J. Philips Purdue University, Department of Chemistry 560 Oval Drive West Lafayette IN 47907 USA Search for other works by this author on: This Site PubMed Google Scholar Nicholas P. Hux ; Nicholas P. Hux Purdue University, Department of Chemistry 560 Oval Drive West Lafayette IN 47907 USA Search for other works by this author on: This Site PubMed Google Scholar Marcy H. Towns Marcy H. Towns Purdue University, Department of Chemistry 560 Oval Drive West Lafayette IN 47907 USA Search for other works by this author on: This Site PubMed Google Scholar Abstract Open the PDF Link PDF for Chapter 9: Assessing System Ontology in Biochemistry: Analysis of Students’ Problem Solving in Enzyme Kinetics in another window
- Chapter 10: Problem Solving in the Chemistry Teaching Laboratory: Is This Something That Happens? p217-252 By Ian Hawkins ; Ian Hawkins Welch College Gallatin TN 37066 USA Search for other works by this author on: This Site PubMed Google Scholar Vichuda K. Hunter ; Vichuda K. Hunter Middle Tennessee State University, Department of Chemistry PO Box 68 Murfreesboro TN 37132 USA [email protected] Search for other works by this author on: This Site PubMed Google Scholar Michael J. Sanger ; Michael J. Sanger Middle Tennessee State University, Department of Chemistry PO Box 68 Murfreesboro TN 37132 USA [email protected] Search for other works by this author on: This Site PubMed Google Scholar Amy J. Phelps Amy J. Phelps Middle Tennessee State University, Department of Chemistry PO Box 68 Murfreesboro TN 37132 USA [email protected] Search for other works by this author on: This Site PubMed Google Scholar Abstract Open the PDF Link PDF for Chapter 10: Problem Solving in the Chemistry Teaching Laboratory: Is This Something That Happens? in another window
- Chapter 11: Problems and Problem Solving in the Light of Context-based Chemistry p253-278 By Karolina Broman Karolina Broman Umeå University Sweden [email protected] Search for other works by this author on: This Site PubMed Google Scholar Abstract Open the PDF Link PDF for Chapter 11: Problems and Problem Solving in the Light of Context-based Chemistry in another window
- Chapter 12: Using Team Based Learning to Promote Problem Solving Through Active Learning p279-319 By Natalie J. Capel ; Natalie J. Capel Keele University UK [email protected] Search for other works by this author on: This Site PubMed Google Scholar Laura M. Hancock ; Laura M. Hancock Keele University UK [email protected] Search for other works by this author on: This Site PubMed Google Scholar Chloe Howe ; Chloe Howe Keele University UK [email protected] Search for other works by this author on: This Site PubMed Google Scholar Graeme R. Jones ; Graeme R. Jones Keele University UK [email protected] Search for other works by this author on: This Site PubMed Google Scholar Tess R. Phillips ; Tess R. Phillips Keele University UK [email protected] Search for other works by this author on: This Site PubMed Google Scholar Daniela Plana Daniela Plana Keele University UK [email protected] Search for other works by this author on: This Site PubMed Google Scholar Abstract Open the PDF Link PDF for Chapter 12: Using Team Based Learning to Promote Problem Solving Through Active Learning in another window
- Chapter 13: Technology, Molecular Representations, and Student Understanding in Chemistry p321-339 By Jack D. Polifka ; Jack D. Polifka Department of Chemistry, Human Computer Interaction Program, Iowa State University Ames IA 50011 USA [email protected] Search for other works by this author on: This Site PubMed Google Scholar John Y. Baluyut ; John Y. Baluyut Math and Science Division, University of Providence Great Falls MT, 59405 USA Search for other works by this author on: This Site PubMed Google Scholar Thomas A. Holme Thomas A. Holme Department of Chemistry, Human Computer Interaction Program, Iowa State University Ames IA 50011 USA [email protected] Search for other works by this author on: This Site PubMed Google Scholar Abstract Open the PDF Link PDF for Chapter 13: Technology, Molecular Representations, and Student Understanding in Chemistry in another window
- Chapter 14: An Educational Software for Supporting Students’ Learning of IR Spectral Interpretation p340-360 By Maria Limniou ; Maria Limniou School of Psychology, University of Liverpool UK [email protected] Search for other works by this author on: This Site PubMed Google Scholar Nikos Papadopoulos ; Nikos Papadopoulos Department of Chemistry, Aristotle University of Thessaloniki Greece Search for other works by this author on: This Site PubMed Google Scholar Dimitris Gavril ; Dimitris Gavril Department of Chemistry, Aristotle University of Thessaloniki Greece Search for other works by this author on: This Site PubMed Google Scholar Aikaterini Touni ; Aikaterini Touni Department of Chemistry, Aristotle University of Thessaloniki Greece Search for other works by this author on: This Site PubMed Google Scholar Markella Chatziapostolidou Markella Chatziapostolidou Department of Chemistry, Aristotle University of Thessaloniki Greece Search for other works by this author on: This Site PubMed Google Scholar Abstract Open the PDF Link PDF for Chapter 14: An Educational Software for Supporting Students’ Learning of IR Spectral Interpretation in another window
- Chapter 15: Exploring Chemistry Problems with Computational Quantum Chemistry Tools in the Undergraduate Chemistry Curriculum p361-384 By Michael P. Sigalas Michael P. Sigalas Aristotle University of Thessaloniki, Laboratory of Quantum and Computational Chemistry, Department of Chemistry Thessaloniki 54124 Greece [email protected] Search for other works by this author on: This Site PubMed Google Scholar Abstract Open the PDF Link PDF for Chapter 15: Exploring Chemistry Problems with Computational Quantum Chemistry Tools in the Undergraduate Chemistry Curriculum in another window
- Chapter 16: Methodological and Epistemological Issues in Science Education Problem-solving Research: Linear and Nonlinear Paradigms p385-413 By Dimitrios Stamovlasis ; Dimitrios Stamovlasis Aristotle University of Thessaloniki Thessaloniki Greece [email protected] Search for other works by this author on: This Site PubMed Google Scholar Julie Vaiopoulou Julie Vaiopoulou Democritus University of Thrace Alexandroupolis Greece [email protected] University of Nicosia Nicosia Cyprus Search for other works by this author on: This Site PubMed Google Scholar Abstract Open the PDF Link PDF for Chapter 16: Methodological and Epistemological Issues in Science Education Problem-solving Research: Linear and Nonlinear Paradigms in another window
- Chapter 17: Issues, Problems and Solutions: Summing It All Up p414-444 By Georgios Tsaparlis Georgios Tsaparlis University of Ioannina, Department of Chemistry Ioannina Greece [email protected] Search for other works by this author on: This Site PubMed Google Scholar Abstract Open the PDF Link PDF for Chapter 17: Issues, Problems and Solutions: Summing It All Up in another window
- Chapter 18: Postscript – Two Issues for Provocative Thought: (a) The Potential Synergy Between HOTS and LOTS (b) When Problem Solving Might Descend to Chaos Dynamics p445-456 By Georgios Tsaparlis Georgios Tsaparlis University of Ioannina, Department of Chemistry Ioannina Greece [email protected] Search for other works by this author on: This Site PubMed Google Scholar Abstract Open the PDF Link PDF for Chapter 18: Postscript – Two Issues for Provocative Thought: (a) The Potential Synergy Between HOTS and LOTS (b) When Problem Solving Might Descend to Chaos Dynamics in another window
- Subject Index p457-467 Open the PDF Link PDF for Subject Index in another window
Advertisement
- Campaigning and outreach
- News and events
- Awards and funding
- Privacy policy
- Journals and databases
- Locations and contacts
- Membership and professional community
- Teaching and learning
- Help and legal
- Cookie policy
- Terms and conditions
- Get Adobe Acrobat Reader
- Registered charity number: 207890
- © Royal Society of Chemistry 2023
This Feature Is Available To Subscribers Only
Sign In or Create an Account

An official website of the United States government
The .gov means it’s official. Federal government websites often end in .gov or .mil. Before sharing sensitive information, make sure you’re on a federal government site.
The site is secure. The https:// ensures that you are connecting to the official website and that any information you provide is encrypted and transmitted securely.
- Publications
- Account settings
Preview improvements coming to the PMC website in October 2024. Learn More or Try it out now .
- Advanced Search
- Journal List
- Taylor & Francis Open Select

Ethics in Science: The Unique Consequences of Chemistry
Jeffrey kovac.
a Department of Chemistry , University of Tennessee , Knoxville, Tennessee, USA
This article discusses the ethical issues unique to the science and practice of chemistry. These issues arise from chemistry’s position in the middle between the theoretical and the practical, a science concerned with molecules that are of the right size to directly affect human life. Many of the issues are raised by the central activity of chemistry––synthesis. Chemists make thousands of new substances each year. Many are beneficial, but others are threats. Since the development of the chemical industry in the nineteenth century, chemistry has contributed to the deterioration of the environment but has also helped to reduce pollution. Finally, we discuss the role of codes of ethics and whether the current codes of conduct for chemists are adequate for the challenges of today’s world.
Editor’s Note In this special issue of Accountability in Research, it is fitting and even necessary that the first article deals with important ethical issues facing the world, in general, and chemists and the chemical community, in particular. How do we preserve and improve the health and safety of the planet? What are the roles and consequences of the chemicals we produce, and how do we produce and manage them? What responsibilities do we have regarding the creation and manufacture of weapons—all of which are “chemical” in one way or another? In this article, we are privileged to have a professor of chemistry and an expert in ethics in science address these and related issues. Jeffrey Kovac has studied, lectured, and published extensively in this field for years. In addition, his CV includes theoretical research in the areas of thermodynamics and statistical mechanics of condensed matter—so, he’s a real scientist! He has also been active in advancing STEM (science, technology, engineering, and mathematics) to pre-collegiate students as director of Tennessee’s Governor’s School for the Sciences and Engineering and as director of the Tennessee Science Olympiad State Tournament. We are fortunate that Professor Kovac has joined our project.
Jeffrey I. Seeman
Guest Editor
University of Richmond
Richmond, Virginia 23173, USA
E-mail: [email protected]
What makes chemistry unique? And how does this uniqueness reflect on chemistry’s unique concerns with ethics?
As Roald Hoffmann argues, it is because chemistry is in the “tense middle,” occupying a space between several pairs of extremes (Hoffmann, 1995 ). Perhaps most importantly, chemistry has always inhabited a frontier between science and technology, the pure and the applied, the theoretical and the practical (Bensaude-Vincent and Simon, 2008 ). Unlike the other natural sciences, chemistry traces its origins to both philosophy and the craft tradition. Chemists are discoverers of knowledge and creators of new substances.
The objects of study in chemistry, molecules and the macroscopic systems made up of molecules, are intermediate between the very small, the elementary particles, and the very large, the cosmos. Chemical systems are the right size to affect humans directly, for better or worse. They are the building blocks of biological organisms; they are the substances we eat and drink; they are the drugs that have dramatically improved human health over the past century; they comprise the materials that we use to construct the products that we use daily; but they are also the environmental pollutants that can plague our world. Chemicals can also be used as weapons.
Being in the middle means that chemists face a unique set of ethical issues, which I will try to explicate in this article. These issues derive, in part, from the nature of chemistry as a science, a science that does not fit the neat picture that is drawn in the first chapter of textbooks. They also derive from the fact that ethics is an inquiry into right human conduct: What is a good life? Chemistry has contributed more to the betterment of human life than any other science, but at the same time has also contributed significantly to the deterioration of the environment. To develop a more complete picture of the nature of chemistry, we need to examine the distinction between pure and applied research more carefully.
PURE VS. APPLIED RESEARCH
As noted, chemistry has always been a mix of pure and applied science, a familiar distinction. The usual view is that pure science is undertaken for its own sake and that its outcome is knowledge or ideas as exemplified by the scientific article. Applied research, on the other hand, is practical. Its aim is to allow us to do things we could not otherwise do, and the outcome is some product or procedure. Applied research uses the results of pure research but does not contribute to the stock of fundamental knowledge.
In his book, Pasteur’s Quadrant , Donald Stokes points out that this bipolar classification does not adequately describe the practice of science (Stokes, 1997 ). Instead of classifying research along a linear scale ranging from pure to applied, Stokes uses a quadrant model. He argues that there are two distinct motivations for research: the quest for fundamental understanding, traditionally called pure research, and the consideration for use, traditionally called applied research. Stokes puts these two motivations, both legitimate, on perpendicular axes as shown in Fig. 1 . This leads to four distinct categories of research. The first category, where the primary motivation is the quest for fundamental understanding with no obvious use, is called Bohr’s quadrant. The work done in this quadrant is what is traditionally called pure research. Certainly, Bohr’s investigations into the fundamentals of quantum mechanics were not conducted with any practical end in sight. Traditional applied research lies in what Stokes called Edison’s quadrant where well-established science is used to solve practical problems. The most interesting category is Pasteur’s quadrant, where the quest for fundamental understanding is carried out in the context of a well-defined end use. Although much of Pasteur’s research was motivated by practical problems of French industry, in the process he essentially created the science of microbiology. The fourth quadrant, which Stokes did not name, is not empty, but it is not relevant to science policy.

Quadrant model for scientific research.
Much of chemistry lies in Pasteur’s quadrant (McGrayne, 2001 ). Although the landscape is changing with the rise of biotechnology, chemistry has always been more connected to the practical than the other sciences. A large fraction of chemists are employed by industry. There is a thriving chemical industry, but no comparable “physical industry,” for example. To be sure, physicists, biologists, geologists, and other scientists also contribute to industrial progress, but not to the same extent as chemists. The usual view is that physicists explore the deep secrets of the universe; biologists are concerned with the evolution of life; chemists make useful stuff.
Take for example the work of Wallace Hume Carothers on condensation or step-growth polymerization at DuPont. Although Carothers was looking for a way to demonstrate the existence of macromolecules using well-known organic reactions, a controversial hypothesis at the time, his work was motivated by the possibility of a commercial product. In the process, he and his research team developed nylon (Hermes, 1996 ).
Carrothers’s work is an example of chemical synthesis, which is perhaps the central activity of chemistry. Chemists make new substances, thousands every year, which means that synthetic chemists change the material world. Most scientists study nature; chemists actually create many of the objects they study. Chemical synthesis confounds the classic categories of pure and applied research. As noted, the usual view is that the outcome of pure research is an idea or knowledge, but synthesis can be undertaken for its own sake to make a new molecule that is interesting not because of its potential use, but because it is particularly symmetric or because the synthesis is particularly challenging. Certainly, new knowledge is gained, but there is another outcome, a new substance which might turn out to be useful.
Another core activity of chemistry is analysis: qualitative and quantitative. Having synthesized or isolated a substance, it is necessary to figure out what one actually has in hand, beginning with the molecular formula and ending with the structure and properties. Until fairly recently, this was done using chemical methods, but these have largely been replaced by spectroscopic techniques, particularly nuclear magnetic resonance (NMR). Analytical chemistry is also concerned with mixtures, both identifying what components are present and determining their concentrations. Analytical chemistry is a combination of science and technology because it often involves the development of a new technique or scientific instrument. An important advance in analytical chemistry during the 20th century was the development of the pH meter which is another example of use-inspired research. Arnold Beckman’s development of the pH meter was stimulated by the needs of the California citrus growers to quickly and accurately determine the acidity of lemons.
Is instrument development pure or applied research? If it is pure research, exactly what new knowledge has been gained? In his provocative book, Thing Knowledge , Davis Baird argues that the physical instrument is actually a form of knowledge, which he terms encapsulated knowledge (Baird, 2004 ). The instrument uses scientific principles, but it also has to work so real-world concerns with materials and how they fit together are important. The pH meter needs two electrodes, the glass electrode sensitive to the hydrogen ion concentration and a reference electrode. Constructing and calibrating reliable electrodes is challenging (Ives and Janz, 1961 ). Like so many things in chemistry, it is both an art and a science. A pH meter also needs appropriate electronics to measure and display the voltage. As Baird points out, in the development of instruments, one runs up against the “thing-y-ness of things,” practical, engineering-like constraints.
The geographic center of chemistry is in the laboratory, a place where scientific knowledge and technique meet. Whatever the chemical experiment, synthesis, analysis, determination of physical, or chemical properties—technique is important. Early chemistry books were filled with recipes, ways to make or isolate substances. Getting a chemical reaction to work is often not easy. There is an old saying that “chemists think with their hands.” Chemistry has often been compared to cooking, usually by those who want to criticize chemistry for its lack of rigorous theory (Bensaude-Vincent and Simon, 2008 ). But for chemists, the close connection to experiment is one of the strengths of the science and also part of the fascination (Sacks, 2001 ).
The practice of chemistry, and all other sciences, raises ethical questions on several levels. Many of these questions arise from the day-to-day work in the laboratory: the responsible conduct of research. Others are related to the relationships of chemists to their colleagues and to the relationship between science and society. To put these questions in context, we need to understand the nature of professional ethics and the moral ideals that underlie the profession of science.
Professionalism and Moral Ideals for Science
In several articles, I have argued that science should be considered a profession based on a definition given by Michael Davis:
A profession is a number of individuals in the same occupation voluntarily organized to earn a living by openly serving a moral ideal in a morally-permissible way beyond what law, market and morality would otherwise require. (Davis, 2002 )
Davis goes on to say that a code of ethics is a central feature of a profession. Chemistry fits this definition and codes of ethics have been adopted by the American Chemical Society (American Chemical Society, 2012 ), American Institute of Chemists (American Institute of Chemists, 1983 ), and the Royal Society of Chemistry (Royal Society of Chemistry, 2012 ). For the classic professions such as law and medicine, it is relatively easy to identify a moral ideal that underlies the profession and its code of ethics. For science, which is not client or patient oriented, the moral ideals are less obvious, particularly in light of the dual motivations for research. There are at least three different ideals corresponding to different aspects of the scientific process (Kovac, 2001 , 2007 ). In the search for fundamental understanding, there must be an ideal that ensures the integrity of the research process. I have called this the ideal of the habit of truth (Bronowski, 1956 ). Whatever the motivation for the research, the day-to-day practice of science requires an absolute integrity. There is an enormous literature on the responsible conduct of research, so I will say little about it in this article, not because it is unimportant, but rather because most of the ethical issues are common to all the sciences. There are several good textbooks that provide an introduction to ethics in science (Shamoo and Resnik, 2009 ; Steneck, 2004 ; Seebauer and Barry, 2001 ; Kovac, 2004 ).
Because science is a form of public knowledge, an ideal of open communication is needed, the ideal of the gift economy. The gifts of the scientist’s research through open communication are what sustain the growth of knowledge. Secrecy is an anathema to the health and progress of science. The ideal of open communication based on the concept of the gift economy is more problematic, particularly when commercial interests are involved, so this will be the topic of some discussion below. Finally, because science has uses there must be an ideal that governs the choice of applications, what I have called, following Norman Care, shared-fate individualism which states that in the conditions of today’s world, service to others should take preference to self-realization in important life decisions including career choice and the choice of research problems (Care, 2000 ; Kovac, 2007 ). Because chemistry is a complex mixture of the fundamental and the applied and because the products of chemistry substantially affect our daily lives, ethical questions related to the uses of chemicals are a central concern. Certainly, other sciences have ethical concerns. Physicists, for example, are involved in the debates regarding the use of nuclear power. Biotechnology has raised questions related to the use of genetically-modified organisms and human cloning. All of these are important, but the products of chemistry, drugs, food additives, personal care products, detergents, as well as the effects of environmental pollution, permeate our lives.
Moral Communities
All chemists simultaneously belong to several communities and each has its own set of responsibilities (Sinsheimer, 1990 ). Each of us is a citizen of a national society with a history, goals and ideals. With citizenship comes obligations. Second, the chemist is a member of a profession subject to the broad professional ethics of science and the more specific codes of ethics of chemistry. Third, almost all chemists are employed by an institution, a college or university, a government or private research laboratory, a government agency, or a corporation. Each of these has its own culture and expectations. Because a large fraction of chemists are employed by industry, the influence of the institution is a more important factor in the ethics of chemistry than for almost any other branch of science. Fourth, all chemists are members of the human community and have the same moral obligations as all other people. Simultaneous membership in these different communities can certainly give rise to moral dilemmas. For example, when does chemist’s moral responsibility as a member of the larger human community take precedence over obligations to an institution or country? The moral landscape might be further complicated by the scientist’s religious beliefs and practices. Because chemistry is a secular pursuit, I will not consider the moral demands of particular faith traditions, but it is important to remember that religious beliefs can strongly influence certain moral decisions.
With this background concerning the unique nature of chemistry as a science “in the middle,” and the nature of professional ethics, we can now turn to a discussion of specific ethical issues. Because it is arguably the central activity of chemistry, we will begin with synthesis, but move from there to a discussion of the laboratory, dangerous substances, especially chemical weapons, and chemistry and the environment. We will end with a critical discussion of the professional codes of ethics of chemistry asking the question of whether they are adequate to deal with the ethical problems of today’s world.
ETHICS AND CHEMICAL SYNTHESIS
Chemical synthesis is an activity that can be carried out in all three quadrants. Some of the ethical questions are the same in all three situations, but the commercial production of chemicals does raise additional issues. I will first consider Bohr’s quadrant where the synthesis is carried out with no particular use in mind. It is often assumed that pure research conducted to gain fundamental understanding is obviously good, but in the case of chemical synthesis there are some complications. Synthesis of a new compound certainly adds to knowledge, but, as pointed out by Joachim Schummer, the new compound also adds to what he calls non-knowledge because the number of substances in the universe has increased and all the properties and reactions of the compound are unknown (Schummer, 2001 ). This presents an interesting ethical question. The production of new knowledge is widely accepted as being a good thing to do, but in the synthesis of a new compound one is also creating uncertainty, which is generally not thought to be good. In addition, every new substance is a new potential threat. Even if the original synthesis only creates a few milligrams the new substance is now known and can be made by others. Perhaps the new compound is a potent neurotoxin or an explosive or a precursor to such a substance. Certainly, it is never possible to envision all the possible outcomes, but it is essential to try. As Roald Hoffmann remarks, “The invention or implementation of a tool without consideration of its use is deeply incomplete” (Hoffmann, 2012a ). This ethical perspective has become a political principle, the precautionary principle, which states that “if an action or policy has a suspected risk of causing harm to the public or to the environment, in the absence of a scientific consensus that the action or policy is harmful, the burden of proof that it is not harmful falls on those taking an act” (Precautionary principle, 2013 ).
In Pasteur’s and Edison’s quadrants, the synthesis is motivated by a possible use for the new substance. There are several ethical questions. First, and most obvious, what will the new substance be used for? There are at least six broad categories of chemicals used in modern society. First are structural chemicals, bulk plastics, and synthetic fibers. Second are agricultural products, pesticides, herbicides, and fertilizers. Third are drugs. The fourth category includes process chemicals, both for industrial and domestic uses. A fifth category is personal care products such as soaps and cosmetics. Finally, there are food-related chemicals. These would include bulk products such as salt and sugar but also food additives, such as flavorings and preservatives. Within these broad categories, however, there are a multitude of kinds of molecules that a chemist might try to make. The decision includes scientific, economic, and ethical considerations. These three factors will be weighed differently if the research is done in a university or government laboratory where the chemist has considerable control over what he or she does, or is it done in an industrial setting where the research agenda is largely determined by the company. My concern in this article is the ethical component.
I assume that the substance to be synthesized has a potential use that will be beneficial to society, perhaps a new drug. But, as noted earlier, every new molecule is a potential threat, so one important ethical question is whether the potential threat is sufficiently outweighed by the benefits. This can be a difficult problem. For example, all drugs have adverse effects—some of them minor, while others catastrophic. Some of these only affect a small percentage of those who take the drug; some affect essentially everybody. Depending on the disease to be treated, patients might be willing to suffer a side effect if the drug treats a terrible disease. Finally, the negative effects might not be easily foreseen.
A useful historical example is the chlorofluorcarbon refrigerants. At the time of their introduction, these were considered to be a great advance because they replaced toxic substances such as methyl chloride and sulfur dioxide as well as liquid ammonia. It was only much later that the negative environmental effects of the chlorofluorocarbons were discovered and a search for more benign alternatives initiated. What was originally considered a great advantage, chemical stability, was later shown to lead to a significant environmental problem, the destruction of ozone in the stratosphere. This is a common situation in the introduction of new chemicals. The evaluation is based on what they will replace. If the new substance has significant advantages and lacks most of the disadvantages of what is currently in use, it is accepted.
The problem of unforseen biological effects is complicated by the existence of chirality. Two compounds that are identical in every way expect that they are stereoisomers can have significantly different effects on an organism. The most familiar example is the sad story of (±)-thalidomide which was prescribed to help pregnant women with morning sickness between 1957 and 1962 but was withdrawn from the market when it was found to be a potent teratogen causing multiple kinds of birth defects. Thalidomide was sold as a racemic mixture as were most drugs at the time because of the cost of separating the left- and right-handed forms compared to the then lack of knowledge about the differences in physiological effects of the two enantiomers. Research done after the drug was removed from the market suggests that only one of the enantiomers is teratogenic, but the situation is complicated by the fact that the “harmless” enantiomer converts to the “harmful” form under physiological conditions. The thalidomide tragedy has led to stronger regulation on drug testing and to the increased production of single enantiomer drugs (Hoffmann, 1995 , Chapter 27; DeCamp, 1989 ). Although drugs and some agricultural products are closely regulated, most chemicals are not, so there is significant risk of unforseen consequences with every new substance.
Purity is another serious issue, particularly in commercial products. Most chemical reactions do not result in a 100% pure product. Unwanted side products are usually formed, and they can be difficult and expensive to remove. Sometimes, these impurities, even when present in very low concentrations, are deadly. The familiar example is dioxin, a highly toxic compound that is an inevitable contaminant of the widely used herbicide 2,4,5-T. Dioxin is found in varying concentrations in all commercial preparations of the herbicide. In principle, it can be removed, but at what cost? At sufficient exposures dioxin is a serious health hazard, but the practical question is whether the level of contamination is large enough to pose a real danger to the public health.
What Should Chemists Synthesize?
Another ethical consideration for use-inspired research is based on the moral ideal of shared-fate individualism. Several years ago, Freeman Dyson published two articles in which he suggested that science was in trouble with the public because of a poor choice of goals (Dyson, 1993 , 1997 ). Rather provocatively, he stated that scientists should be working on “necessities for the poor” rather than “toys for the rich.” A philosophical basis for this suggestion was given by Norman Care (Care, 2000 ). Care analyzes the situation in today’s world which includes vast disparities in quality of life particularly between the developed and developing countries but also between socioeconomic groups in our own country. To this, he adds a concern for future generations and for the future of the planet. (A similar analysis has been given by John Forge, in Forge, 2008 ). In light of the conditions of today’s world, Care suggests that it should be a moral imperative that what he calls competent individuals, those who have significant talent, education, and resources, to put service to others ahead of self-realization in significant life decisions. Chemists certainly meet Care’s criteria for being competent individuals based on their advanced educations and continuing professional experience. I have suggested that for scientists Care’s principle means that in the choice of research problems, necessities for the poor should take priority over toys for the rich (Kovac, 2007 ). Further, it means that doing research to ensure the long-term well-being of the population and the planet should take on the highest priority. For example, if one is synthesizing new drugs, treatments for diseases that affect large number of people, such as malaria, should take priority over designing a drug to treat a relatively rare disease suffered only by affluent people in developed countries of the world. On the other hand, it can be argued that it is necessary for pharmaceutical companies to produce high-value drugs to maintain their profitability so that they can engage in research on drugs to treat diseases in underdeveloped countries. This is certainly one of the ethical tensions of commercial science.
Shared-fate individualism is a moral ideal, not a moral rule. Ideals represent our best aspirations, but no one should be blamed for not reaching an ideal. The choice of research problems is not always in the hands of the individual, particularly for chemists who work in industry or even in government laboratories. A second constraint is that the individual chemist may not have the background or research competence required to tackle a socially important problem. Finally, it is often hard to predict the ultimate effects of a particular invention. A drug designed to cure a particular disease might turn out to be effective for a completely different condition. A “toy for the rich” might eventually become something that makes life better for large numbers of non-affluent people. Many modern electronic devices, such as the personal computer and the cell phone, which were once only available to the affluent, have become so inexpensive that they are available to large numbers of people world-wide and have changed their lives for the better.
Ethics in the Laboratory
Chemistry is rooted in the laboratory where ideas, knowledge, and technique come together. Getting a chemical reaction to work satisfactorily, in a reasonable amount of time, and with a good yield can be tricky. A classic volumetric analysis requires careful use of glassware: volumetric flasks, pipettes, and burettes. Accurate weighing is an essential part of chemistry.
One of the core principles of science, and an important moral rule, is to describe experimental procedures completely and carefully so that another person can reproduce the results. Anyone who has tried to reproduce an experiment described in the chemical literature knows that this is easier said than done. Sometimes, an experiment cannot be reproduced because it really did not happen that way; the results are fabricated. Usually, however, the reasons do not involve scientific misconduct. Often experimental details are unintentionally omitted due to carelessness or because they seem obvious or are part of the usual routine of a particular research group or because of poor record keeping. A more ethically interesting reason is that some people are better at doing experiments than others. They are more careful or just seem to have a knack for making things work. For example, apparently the only person who could get Robert Boyle’s air pump to work properly was the man who made it, Robert Hooke (Shapin, 2010 ). In chemistry, we say that some people just have “magic hands” (Stemwedel, 2006 ). The difficult ethical issue is the following one. Can we call an experiment reproducible if the only people who can get it to work properly are those with magic hands? What is the responsibility of the original research group to ensure that the procedure can be reproduced by an average chemist? This is a problem in all of laboratory science, but it is perhaps most important in chemistry which involves as much art as science.
Some related issues have been recently discussed by Carlson and Hudicky who discuss malpractice in organic synthesis (Carlson and Hudlicky, 2012 ). They distinguish malpractice from scientific misconduct. Malpractice is not a deliberate attempt to deceive, but instead it is a result of improper practice. They list three classes of improper practice in organic synthesis: improper experimental protocols, improper methods used in characterization of compounds, and the lack of proper citations to previous work. Probably the greatest concern is improper characterization which can lead to errors in structural assignments. The primary method used is NMR, a powerful tool, but not always definite. When syntheses are carried out in microscale, it may be impossible to obtain some of the classic data, such as a percent composition by combustion analysis, or even a good melting point. Without a definitive characterization of the product, the results may not be trustworthy.
Analytical chemistry also presents ethical challenges in the laboratory. As discussed above, many synthetic commercial chemical products contain impurities, some of which are dangerous. Products derived from natural sources are usually complex mixtures. Sea salt, which has recently gained popularity in gourmet cooking, is mainly sodium chloride, but also contains small concentrations of other cations and anions. Beverages such as coffee, tea, or wine can contain hundreds of components in widely varying concentrations. The task of the analytical chemist is to determine what components are present, which involves separation and identification, and then to determine how much is present. Knowing what is present is an important question in product safety for example.
There are several aspects of the analysis process that can raise ethical questions. One is the problem of detection limit. All chemical analyses have a lower limit of detection so the question of whether a trace component is part of a mixture can only be answered by saying that, if it is there, it is present in a quantity less than the detection limit. If the suspected contaminant is pernicious, this can put the analyst in a difficult position. Here is a simple example (Kovac, 2000 ). Suppose the analytical chemist is called to testify in a lawsuit where the crucial issue is whether a particular contaminant was present. The question from the lawyer would be, “Was substance A present?” In an adversary proceeding, the expected answer is either “yes” or “no.” But the professional ethics of science require a more nuanced answer like, “It was not detected, but the method used can only detect concentrations greater than X.” Although the analyst could answer “no” without committing perjury, such an answer would not be scientifically responsible.
Another issue is chemical calibration (Edmonds, 1999 ). Quantitative determination of the concentration of a substance often requires a chemical calibration using known amounts of the substance to be determined, which may have to be synthesized, in a matrix that is sufficiently similar to that of the unknown sample. The question of what is sufficiently similar is a matter of judgment and, as I and others have argued, scientific judgments have both a technical and a moral component. Depending on the circumstances, getting it wrong can have serious consequences.
Chemical Weapons and Other Dangerous Substances
A very difficult ethical question for chemists is whether to conduct research on chemical weapons. These substances are banned under the Chemical Weapons Convention of 1993, which has been signed by nearly every country in the world including the United States. The Chemical Weapons convention prohibits the development, production, stockpiling, and use of chemical weapons. It also mandated the destruction of all chemical weapons and destruction or conversion of all production facilities by 2007. Progress in accomplishing this latter goal has been delayed, but most of the stockpiles of these weapons have been destroyed and the production plants deactivated.
In recent years, there has been less fear of the use of chemical weapons in wars between nations, but more concern about their production and use by terrorist organizations or by what are sometimes called rogue states. Research on chemical weapons continues, for example at the U.S. Army Medical Institute of Chemical Weapons Defense (Chemical Weapons Defense, 2013 ). Of course, much of this research is classified, but even defensive research might involve the development of new chemical weapons with the goal of finding appropriate counter measures.
There is also research on what are being called non-lethal chemical weapons which include anti-traction agents and malodorants or other novel chemical agents (Guardian, 2008 ). There is some question as to whether these new non-lethal agents violate the Chemical Weapons Convention because it is not known whether they are toxic at high doses. Malodorants might also have adverse effects other than toxicity, adverse psychological effects for example.
The broader ethical question as to whether scientists should engage in war-related research is complex, but chemical weapons raise that question in stark terms (Kovac, 2013 ). The use of chemical weapons, initially chlorine gas by Germany in World War I and subsequently chlorine and other compounds by the Allies, produced enormous moral outrage. One reason is that chemical weapons pose a greater risk to innocent bystanders than conventional weapons. Unlike bullets, they cannot be precisely aimed. Once the cloud of poison is released, everyone who encounters it is affected. Even in the just war tradition, there is a prohibition against using weapons that are “evil in themselves” (no means mala in se ). Chemical weapons such as nerve agents seem to fit this description, but so do weapons such as napalm that was extensively used in the Vietnam War. The broad scale use of herbicides such as the infamous “Agent Orange,” also used in Vietnam, also seem to be inhumane. Because chemists are the ones that develop these substances, chemists must confront the ethical implications.
Weapons research is an area where the obligations of the different moral communities to which the chemist belongs can come into conflict. As citizens, chemists may feel an obligation to contribute to the national defense of their country including weapons development. As employees of a particular institution, they may be pressured to work on weapons related research. On the other hand, as a member of the human community, the chemist might feel that use of chemical weapons is immoral. Unfortunately, the professional codes of ethics of chemists do not help to resolve this dilemma. This is a question that will be discussed in more detail below.
Weapons research is a particularly challenging issue, but there are others. For example, tobacco use is a serious health risk. Should a chemist engage in research to produce safer cigarettes? On the one hand, it might be argued that such research would reduce the risks of smoking. On the other hand, the chemist is helping to perpetuate an industry that produces an intrinsically harmful product. Another example is a better abortion drug, the so-called “morning-after pill.” Here is where personal morals, perhaps derived from religious beliefs, come into play.
Environmental Pollution and Green Chemistry
Whatever the substance to be made, there are ethical issues related to the method of production. Since its inception, the modern chemical industry has been responsible for widespread environmental degradation (Bensaude-Vincent and Simon, 2008 ). Well publicized accidents such as the disaster in Bhopal, where thousands of Indians were poisoned by methyl isocyanate leaking from a Union Carbide plant, have added to the negative public image of chemistry.
There are practical, economic, and ethical reasons to improve safety at chemical plants and to reduce the environmental impact of the production of chemicals. The effort to make chemical production more environmentally benign is usually called Green Chemistry. I think we can stipulate that the chemical industry will adopt cleaner and greener methods of production if they make economic sense or are required by government regulation or severe public pressure. The question is who will develop the new chemistry. Certainly, some of the research will take place in industrial laboratories, but as recently argued by Roald Hoffmann, “the spiritual center of chemistry in our country remains in the research universities. Where people are taught, values are formed” (Hoffmann. 2012b ). The ideal of shared fate individualism suggests that chemists in research universities should focus more of their research efforts on green chemistry: atom and energy economy and benign solvents. It will be a hard sell because there is a prejudice that such research is boring, routine industrial-style research to find a new way to make something ordinary. What self-respecting academic organic chemist would want to find a greener synthesis of ibuprofen? What glory in that?
As Hoffmann points out, polymer chemistry once had a similar reputation of being a mundane, mainly industrial field. Today, because of the research accomplishments of some outstanding academic chemists polymer chemistry has become a respectable academic endeavor. If a few prominent academic chemists responded to the moral ideal of shared-fate individualism and showed that Green Chemistry is both scientifically interesting and attractive to graduate students, the field might blossom. Adding case studies of the successes of green chemistry to the curriculum in textbooks and laboratory courses would also help interest students in this field.
The current environmental crisis raises other scientific and ethical questions. First is environmental remediation, cleaning up the messes that industrial chemistry has made over the past century and more. A second one is what has come to be called sustainability, finding ways to use renewable resources rather than petroleum as both fuels and feedstocks. There is an obvious link to global warming, reducing the production of greenhouse gases. There has been progress; the development of the modern catalytic converter has done much to reduce air pollution, but much more needs to be done. Bensaude-Vincent and Simon argue that we need a new chemical culture in relationship to the environment, one that proscribes the causes of dangers to human and environmental health (Bensaude-Vincent and Simon, 2008 ). They suggest that chemists embrace the Hippocratic principle of medical ethics: first, do no harm. They extend Hoffmann’s argument that the chemistry needs to consider the possible uses of a new substance or process to say that chemists should anticipate the long-term negative consequences of their actions.
Codes of Ethics
The Chemist’s Code of Conduct of the American Chemical Society presumably applies to all chemists (American Chemical Society, 2012 ). It lists the responsibilities of chemists to various groups beginning with the responsibilities of chemists to the public:
Chemists have a professional responsibility to serve the public interest and welfare and to further knowledge of the science. Chemists should actively be concerned with the health and welfare of coworkers, consumers and the community. Public comments on scientific matters should be made with care and precision, without unsubstantiated, exaggerated, or premature statements. (American Chemical Society, 2012 )
Michael Davis contrasts this statement with several of the provisions of the code of ethics for engineers adopted by the Accreditation Board of Engineering and Technology (ABET) which presumably applies to all engineers (Davis, 2002 ). One of the fundamental principles of the ABET code is the following one:
Engineers uphold and advance the integrity, honor, and dignity of the engineering profession by: 1. using their knowledge and skill for the advancement of human welfare.
The first “fundamental canon” of the ABET code states as follows:
1. Engineers shall hold paramount the health, safety, and welfare of the public in the performance of their professional duties (ABET, 1977 ).
An identical statement is the first requirement of the Code of Ethics of the American Institute of Chemical Engineers (AIChE, 2006 ).
Although the two codes are similar, there are important differences. Chemists are supposed to be “actively concerned with the health and welfare of coworkers, consumers and the community” (American Chemical Society, 2012 ), but engineers are to “hold paramount the health, safety, and welfare of the public” (ABET, 1977 ). Further, engineers should advance human welfare, not just be actively concerned with it. As Davis points out, the ACS code lists various responsibilities with no guidance as to how to deal with conflicts. If the chemist’s responsibility for welfare comes in conflict with the chemist’s responsibility to an employer, the code does not say which should have priority. For the engineer, the priorities are clear. Take care of the public first; everything else comes second.
Davis raises an important issue for the chemical profession. In a world where the public health and welfare is threatened by the effects of chemicals, particularly environmental pollution, should the code of ethics be revised to make the chemist’s responsibility to the health and well-being of the public and to the environment a higher priority. Should chemists also be encouraged to use their talents for the advancement of human welfare? The profession needs to be held to a higher standard, and the codes of ethics for chemists need to be revised.
CONCLUDING REMARKS
Throughout its history, chemistry has made significant contributions to human progress but with those successes have come problems, especially problems of environmental pollution. Synthetic chemicals have become a major part of our lives. The circumstances of today’s world provide both scientific and ethical challenges for chemistry. In this article, I have outlined many of those challenges focusing on ethical questions that derive from the unique nature of chemistry as a science. Perhaps the most important ethical issues involve chemical synthesis. When a new substance is created, chemists need to think about the long-term effects of that compound. If the substance is made commercially, we need to develop “greener” methods of production that conserve nonrenewable resources and minimize the effects on the environment. On a broader level we need to consider the problems of today’s world and work on problems that will improve the human condition, particularly the lives of those in underdeveloped countries. Chemists also need to think carefully about their role in preserving the health and safety of the planet, including their role in the creation of weapons.
More than the other sciences, chemistry is centered in the laboratory so it is important that laboratory practice adhere to the highest professional and ethical standards. Finally, the chemical profession through the professional societies needs to reexamine the codes of ethics to ensure that they respond to the practical and ethical challenges of today’s world in which synthetic chemicals touch essentially every part of our daily lives.
Of course, chemists face the same ethical challenges that all other scientists, and indeed all human beings must confront. Our future depends on our willingness to ask and answer these crucial ethical questions.
ACKNOWLEDGMENTS
I am grateful to Jeffrey I. Seeman, Roald Hoffmann, Stan Guffey, and Christopher Pynes for reading drafts of this article and providing suggestions for its improvement.
The author thanks the Innovative Grant Program (IGP) of the American Chemical Society. The IGP has provided the funds that permit permanent open access to this article and all articles in this special issue of Accountability in Research titled, “Ethics and Responsible Conduct of Research within the Chemical Community. Ideas and Experiences Worth Sharing.”
- ABET 1977 Code of Ethics of Engineers, https://engineering.purdue.edu/MSE/Academics/Undergrad/ethics.pdf (Accessed June 3, 2015)
- AIChe 2006 Code of ethics. http://sts.aiche.org/content/code-ethics (accessed August 1, 2013)
- American Chemical Society 2012 The chemical professional’s code of conduct. http://www.acs.org/content/acs/en/careers/profdev/ethics/the-chemical-professionals-code-of-conduct.html (accessed July 29, 2013)
- American Institute of Chemists 1983 Code of ethics. http://www.theaic.org/about_ethics.html (accessed July 29, 2013)
- Baird D. Thing knowledge: A philosophy of scientific instruments . Berkeley and Los Angeles: University of California Press; 2004. [ Google Scholar ]
- Bensaude-Vincent B. Simon J. Chemistry: The impure science . London: Imperial College Press; 2008. [ Google Scholar ]
- Bronowski J. Science and human values . Revised. New York: Harper Torchbooks; 1956. Edition. [ Google Scholar ]
- Care N. S. Decent people . Lanham, MD: Rowman and Littlefield; 2000. [ Google Scholar ]
- Carlson R. Hudlicky T. On hype, malpractice, and scientific misconduct in organic synthesis. Helvetica Chimica Acta . 2012; 95 :2052–2062. [ Google Scholar ]
- Chemical Weapons Defense 2013 Research for the warfighter. http://chemdef.apgea.army.mil/ (accessed January 5, 2015)
- Davis M. Do the professional ethics of chemists and engineers differ? Hyle . 2002; 8 :21–34. [ Google Scholar ]
- DeCamp W. H. The FDA perspective on the development of stereoisomers. Chirality . 1989:2–6. [ PubMed ] [ Google Scholar ]
- Dyson F. Science in trouble. The American Scholar . 1993; 62 :513–522. [ Google Scholar ]
- Dyson F. Can science be ethical. New York Review of Books . 1997; 44 :6. [ Google Scholar ]
- Edmonds T. E. A philosophical approach to analytical chemistry. Unpublished manuscript; 1999. [ Google Scholar ]
- Forge J. The responsible scientist: A philosophical inquiry . Pittsburgh: University of Pittsburgh; 2008. [ Google Scholar ]
- Guardian 2008 US weapons research is raising a stink. http://www.guardian.co.uk/science/2008/jul/10/weaponstechnology.research (accessed July 1, 2013).
- Hermes M. E. Enough for one lifetime: Wallace carothers, inventor of Nylon . Washington, DC: American Chemical Society and Chemical Heritage Foundation; 1996. [ Google Scholar ]
- Hoffmann R. The Same and not the same . New York: Columbia University Press; 1995. [ Google Scholar ]
- Hoffmann R. Ethics and science. In: Kovac J., editor; Weisberg M., editor. Roald Hoffmann on the Philosophy, Art, and Science of Chemistry . New York: Oxford; 2012a. pp. 360–376. [ Google Scholar ]
- Hoffmann R. The material and spiritual rationales are inseparable. In: Kovac J., editor; Weisberg M., editor. Roald hoffmann on the philosophy, art, and science of chemistry . New York: Oxford; 2012b. pp. 339–346. [ Google Scholar ]
- Ives D. J. G., editor; Janz G. J., editor. Reference electrodes . New York and London: Academic Press; 1961. [ Google Scholar ]
- Kovac J. Science, law, and the ethics of expertise. Tennessee law review . 2000; 67 :397–408. [ Google Scholar ]
- Kovac J. Gifts and commodities in chemistry. Hyle . 2001; 7 :141–153. [ Google Scholar ]
- Kovac J. The ethical chemist: Professionalism and ethics in science . Upper Saddle River: Pearson Education; 2004. [ Google Scholar ]
- Kovac J. Moral rules, moral ideals and use-inspired research. Science and Engineering Ethics . 2007; 13 :159–169. [ PubMed ] [ Google Scholar ]
- Kovac J. Science, ethics and war: A pacifist’s perspective. Science and Engineering Ethics . 2013; 19 :449–460. [ PubMed ] [ Google Scholar ]
- McGrayne S. B. Prometheans in the lab: Chemistry and the making of the modern world . New York: McMillan; 2001. [ Google Scholar ]
- Precautionary principle 2013 Precautionary principle. http://en.wikipedia.org/wiki/Precautionary_principle . (accessed August 12, 2013)
- Royal Society of Chemistry 2012 Regulation of the profession and code of conduct. www.rsc.org/images/code-of-conduct_tcm18-5101.pdf (accessed June 3, 2015.
- Sacks O. Uncle tungsten . New York: A. A. Knopf; 2001. [ Google Scholar ]
- Schummer J. Ethics of chemical synthesis. Hyle . 2001; 7 :103–124. [ Google Scholar ]
- Seebauer E. G. Barry R. L. Fundamentals of ethics for scientists and engineers . New York and Oxford: Oxford University Press; 2001. [ Google Scholar ]
- Shamoo A. E.Resnik D. B.2009 Responsible conduct of research second edition Oxford, New York: Oxford University Press [ Google Scholar ]
- Shapin S. Shapin Steven. Never Pure . Baltimore: Johns Hopkins Press; 2010. Who was Robert Hooke?” [ Google Scholar ]
- Sinsheimer R. L. Scientists and Research. In: Wright S., editor. Preventing a Biological Arms Race . Cambridge: MIT Press; 1990. pp. 71–77. [ Google Scholar ]
- Stemwedel J. 2006 Reproducibility and retracted papers. http://scientopia.org/blogs/ethicsandscience/2006/06/15/reproducibility-and-retracted-papers/ (accessed June 3, 2015)
- Steneck N. H. Introduction to the responsible conduct of research . Washington, D.C.: U. S. Government Printing Office; 2004. [ Google Scholar ]
- Stokes D. E. Pasteur’s quadrant . Washington, D.C.: Brookings Institution Press; 1997. pp. 46–49. [ Google Scholar ]

Solving the problems of proton-conducting perovskites for next-generation fuel cells
As a newly developed perovskite with a large amount of intrinsic oxygen vacancies, BaSc 0.8 W 0.2 O 2.8 achieves high proton conduction at low and intermediate temperatures, report scientists at Tokyo Tech. By the donor doping of large W 6+ , this material can take up more water to increase its proton concentration, as well as reduce the proton trapping through electrostatic repulsion between the dopant and proton. These findings could pave the way to the rational design of novel perovskites for protonic ceramic fuel cells (PCFCs) and electrolysis cells (PCECs).
In line with global efforts towards cleaner energy technologies, fuel cells may soon become an indispensable tool for converting chemical energy -- stored in the form of hydrogen or other fuels -- into electrical energy. Among the various types of fuel cells being actively researched, those that use solid electrolytes rather than liquid ones have inherent safety and stability advantages.
In particular, protonic ceramic fuel cells (PCFCs) have attracted special attention among scientists. These devices do not operate via the conduction of oxide ions (O 2− ) but light protons (H + ) with smaller valence. A key feature of PCFCs is their ability to function at low and intermediate temperatures in the range of 50-500 °C. However, PCFCs based on perovskite electrolytes reported thus far suffer from low proton conductivity at low and intermediate temperatures.
In a recent study, a research team led by Professor Masamoto Yashima from Tokyo Institute of Technology (Tokyo Tech), in collaboration with High Energy Accelerator Research Organization (KEK), has set out to address this limitation of perovskite-based proton conductors. Their findings were published in the Journal of Materials Chemistry A on May 3, 2024.
But why is the conductivity of the conventional perovskite-type proton conductors so low? "A major problem with the conventional proton conductors is a phenomenon known as proton trapping, in which protons are trapped by acceptor dopant via electrostatic attraction between the dopant and proton," explains Yashima. "Another major problem among such proton conductors would also be their low proton concentration due to the small amount of oxygen vacancies."
To tackle these issues, the researchers developed a highly oxygen-deficient perovskite, namely BaScO 2.5 doped with W 6+ cations, or BaSc 0.8 W 0.2 O 2.8 . Thanks to its large amounts of oxygen vacancies, this material has a higher proton concentration than other proton-conducting perovskites. However, since proton hopping occurs between oxygen atoms, the oxygen vacancies would lower proton conductivity rather than increase it.
This problem was solved by full hydration of the perovskite, turning it into BaSc 0.8 W 0.2 O 3 H 0.4 . Because of the large size of the W 6+ dopant, the perovskite has a larger lattice volume, which means it can take up more water molecules than those doped with other cations such as small Mo 6+ . The high water uptake facilitates high proton conductivity by further increasing the proton concentration.
As for proton trapping, the high positive charge of the W 6+ dopant leads to a stronger repulsion with protons, which are also positively charged. This effect was confirmed through ab initio molecular dynamics simulations, which revealed the migration pathways of protons near the Sc cation when transporting across the material. The repulsion indicates reduced proton trapping by the W 6+ dopant, which leads to the high proton conductivity at low and intermediate temperatures.
Taken together, the insights provided by this study could help establish fundamental design principles for proton-conducting perovskites. "The stabilization of perovskites with disordered intrinsic oxygen vacancies and full hydration enabled by doping of large donor dopant could be an effective strategy towards next-generation proton conductors," remarks Yashima.
In addition to PCFCs, proton conductors are also needed in proton-conducting electroysis cells (PCECs), which can efficiently utilize electricity. Both of these technologies will be essential in the near future as we collectively strive towards sustainability through novel proton conductors.
Overall, the present findings may open new avenues to realize clean energy societies.
- Organic Chemistry
- Energy and Resources
- Alternative Fuels
- Electricity
- Ethanol fuel
- Quantum dot
- Fossil fuel
- Tensile strength
Story Source:
Materials provided by Tokyo Institute of Technology . Note: Content may be edited for style and length.
Journal Reference :
- Kei Saito, Kensei Umeda, Kotaro Fujii, Kazuhiro Mori, Masatomo Yashima. High proton conduction by full hydration in highly oxygen deficient perovskite . Journal of Materials Chemistry A , 2024; DOI: 10.1039/d4ta01978d
Cite This Page :
Explore More
- How Statin Therapy May Prevent Cancer
- Origins of 'Welsh Dragons' Exposed
- Resting Brain: Neurons Rehearse for Future
- Observing Single Molecules
- A Greener, More Effective Way to Kill Termites
- One Bright Spot Among Melting Glaciers
- Martian Meteorites Inform Red Planet's Structure
- Volcanic Events On Jupiter's Moon Io: High Res
- What Negative Adjectives Mean to Your Brain
- 'Living Bioelectronics' Can Sense and Heal Skin
Trending Topics
Strange & offbeat.
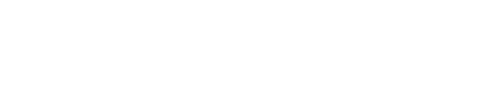
May 30, 2024
Guilford Chemistry Major Recognized for Research in Producing Hydrogen
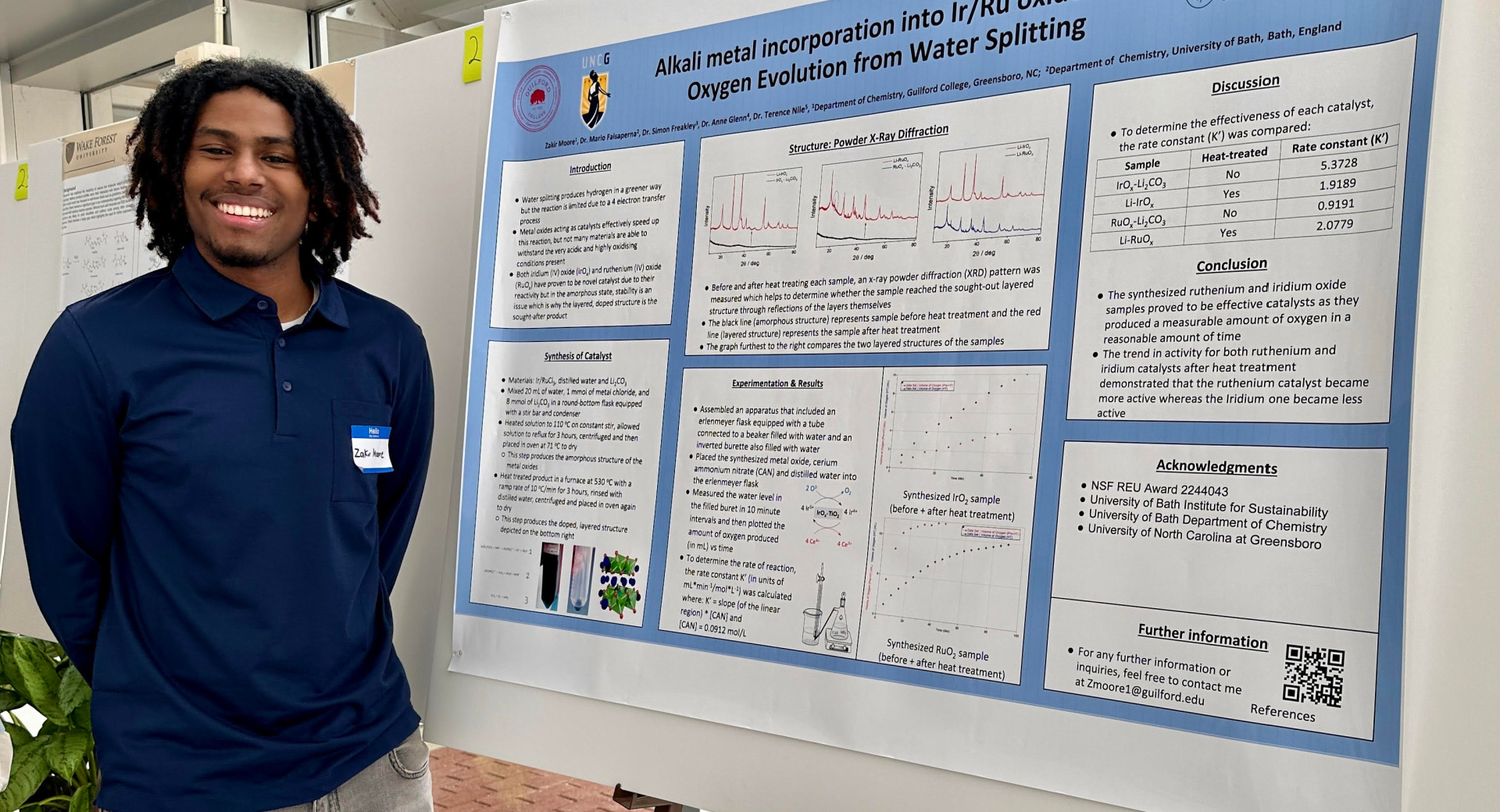
Zakir Moore '25 stands next to his winning presentation.
Out of more than 60 entries, Zakir Moore's work was recognized by the Central North Carolina Section of the American Chemical Society.
Zakir Moore ’25 was recently recognized for his research into producing industrial quantities of hydrogen in a safer and a more environmentally friendly method.
The Central North Carolina Section of the American Chemical Society awarded Zakir first place for his research among undergraduates at the group’s annual Poster Vendor Night at the Joint School of Nanoscience and Nanoengineering in Greensboro.
Poster Vendor Night is an opportunity for college students and faculty to talk with vendors about the latest lab products and instruments as well as showcasing research being done in the Triad area by students.
Currently, more than 90 percent of hydrogen produced industrially comes from fossil fuels, which release greenhouse gasses into the atmosphere and contribute to global warming. Hydrogen can be produced in a safer and greener way through the electrolysis of water, but the reaction can be slow and inefficient.
Zakir’s research poster, one of more than 60 that were presented by graduate and undergraduate students, examined using metal oxides as catalysts to speed up the reaction and proceed effectively.
Fellow Chemistry majors Noah Dabney '24 and Maggie Mahanes '25 also presented. Noah and Zakir presented research they did as participants in the NSF-funded Research Experience for Undergraduates (REU) Program "Chemistry for a Sustainable Future" at the University of Bath in 2023.
Maggie presented her research from work she did last summer at Wake Forest University as part of a program funded by the Wake Forest University Translational Science Center.
Share This!
Guilford Trustees Endorse Strategic Plan
Wendy gates corbett '91 writes about workplace connections, grant will help enhance underground railroad trail, we’ve saved your selection..
If you would like to keep your notes for further reference, please create an account.
Already have an account? Log in

Green Chemistry
Light-swing co2 capture: photoirradiation-based chemical co2 release based on photoisomerization of azobenzene-amine/guanidine derivatives.
The world is committed to reducing CO2 emissions, and research on CO2 capture and effective utilization is being actively studied. Among the methods in development, direct air capture (DAC) is classified as a negative emission technology and has attracted significant study. The current problem with CO2 capture technologies for decarbonization is their cost due to the high separation energy required to release CO2. We have developed a new light-swing method that can potentially utilize a natural source of energy, i.e., sunlight, as an alternative to temperature- and pressure-swing methods. Herein, we report photoirradiation-based CO2 capture based on photoisomerization of azobenzene-amine and guanidine derivatives. The visible light-swing CO2 absorption and release system using azobenzene-guanidine has shown potential in DAC systems owing to its reusability. A plausible mechanism for CO2 release under light irradiation involves photoisomerization from trans- to cis-azobenzene in which steric repulsion with other molecules is the driving force, and CO2 is released due to the functional disruption of intermolecular interactions. This concept demonstrates the potential of using various photokinetic molecules as a driving force for light-swing CO2 capture.
Supplementary files
- Supplementary information PDF (3654K)
- Crystal structure data CIF (1402K)
Article information

Download Citation
Permissions.

R. Murakami, K. Shiota, A. Uchida and F. Inagaki, Green Chem. , 2024, Accepted Manuscript , DOI: 10.1039/D4GC00736K
This article is licensed under a Creative Commons Attribution 3.0 Unported Licence . You can use material from this article in other publications without requesting further permissions from the RSC, provided that the correct acknowledgement is given.
Read more about how to correctly acknowledge RSC content .
Social activity
Search articles by author.
This article has not yet been cited.
Advertisements
Your browser is not supported
Sorry but it looks as if your browser is out of date. To get the best experience using our site we recommend that you upgrade or switch browsers.
Find a solution
- Skip to main content
- Skip to navigation
- hot-topics Extras
- Newsletters
- Reading room
Tell us what you think. Take part in our reader survey
Celebrating twenty years
- Back to parent navigation item
- Collections
- Water and the environment
- Chemical bonding
- Antimicrobial resistance
- Energy storage and batteries
- AI and automation
- Sustainability
- Research culture
- Nobel prize
- Food science and cookery
- Plastics and polymers
- Periodic table
- Coronavirus
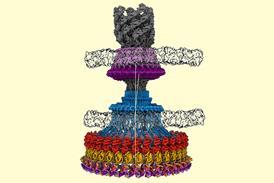
- More from navigation items
Simplified hydroformylation replaces rhodium with base metal

- No comments
A bench-friendly alternative to traditional hydroformylation replaces the pressurised toxic gas mixture and expensive rhodium catalyst with a commercially available electrophile and cheap copper reagent. Through careful choice of ligand, US-based researchers closely controlled the stereoselectivity of the reaction to generate a range of valuable aldehydes for synthesis and drug discovery.
‘Hydroformylation is an atom-economic and catalytic method to convert alkenes into functional molecules such as aldehydes,’ says Samir Chikkali , an organometallic chemist at the CSIR-National Chemical Laboratory in India. In the presence of a rhodium catalyst, a pressurised mixture of hydrogen and carbon monoxide adds across the alkene double bond to form an aldehyde. Further transformations may then convert this product into other useful feedstocks such as alcohols, amines and carboxylic acids. The ubiquity of these functional groups across pharmaceutical, fragrance, and agrochemical products makes hydroformylation one of the most important industrial processes globally. But despite this, Chikkali explains that the reaction does have limitations. In particular, he points to the requirement of ‘precious and rare rhodium’ and ‘the use of toxic carbon monoxide under high pressures’.
‘These conditions are not amenable to employment by everyday chemists, particularly in the pharmaceutical industry,’ says Stephen Buchwald , a catalysis chemist at the Massachusetts Institute of Technology, US. ‘What we’ve done is develop an alternative technique that people in academia or the pharmaceutical industry could use straight out of the box.’
Breaking the hydroformylation reaction into its component parts, the team, jointly led by Buchwald and computational chemist Peng Liu at the University of Pittsburgh, used established organocopper chemistry to activate a variety of styrene substrates, introducing the carbonyl via a reactive oxocarbenium electrophile. The overall transformation therefore proceeds via a completely different mechanism from the industrial process, avoiding the use of both an expensive rhodium catalyst and pressurised toxic gas.
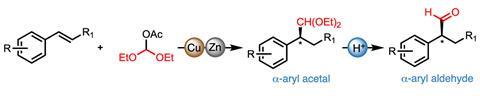
Source: © 2024 American Chemical Society
The new process converts styrene substrates into chiral aldehydes
‘The clever part is how they’ve converted the hydrocupration reaction into a hydroformylation by introducing the oxocarbenium electrophile,’ explains Arnald Grabulosa , a catalysis researcher at the University of Barcelona in Spain. ‘The copper hydride adds over the double bond to catalytically form an organocopper complex. This reacts with the extremely electrophillic oxocarbenium species, generated in situ from a commercial orthoester, to give the acetal which is hydrolysed to the hydroformylation product.’
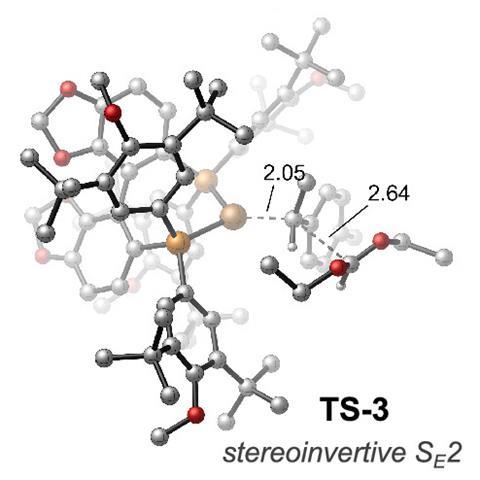
The transition state through which the addition of the oxocarbenium ion to the Cu-alkyl intermediate proceeds
At 6%, the catalyst loading for this process is significantly higher than the industrial rhodium reaction but neither the US team, nor Grabulosa believe this will impact its use in academic and discovery chemistry. Crucially, careful choice of the ligand for the hydrocupration step enabled Buchwald and Liu to control the stereochemistry of the final aldehyde product. ‘We’re always happier at lower catalyst loadings but the expensive thing is the ligand and not the copper,’ says Buchwald. ‘And truthfully, it only really matters at large scale which is not the focus here.’
Both Chikkali and Grabulosa are excited to see how this simplified method will impact hydroformylation chemistry in academia. ‘This is an excellent piece of work and offers considerable potential to replace rhodium with copper. The enantioselectivity is the icing on the cake,’ says Chikkali.
‘It can be considered an upgraded version of hydroformylation and has the potential to be widely used,’ says Grabulosa. ‘The next steps should be to expand the substrate scope beyond styrenes and explore other ligands.’
S Garhwal et al , J. Am. Chem. Soc. , 2024, DOI: 10.1021/jacs.4c04287
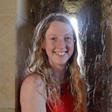
More from Victoria Atkinson
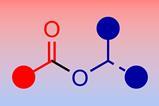
How A-level chemistry solved the 200-year-old problem with the haloform reaction
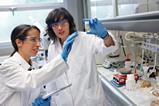
How to find and make the most of a summer placement
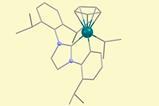
Summer students act as an important catalyst for research
- asymmetric catalysis
- Homogeneous catalysis
- Industrial chemistry
- Organic chemistry
- organometallic
- Pharmaceutical industry
Related articles
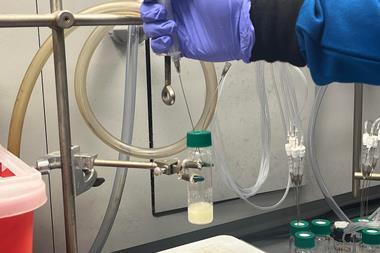
Cross-coupling technique cracks open alcohols for chemical synthesis
2024-03-26T14:30:00Z
By James Urquhart
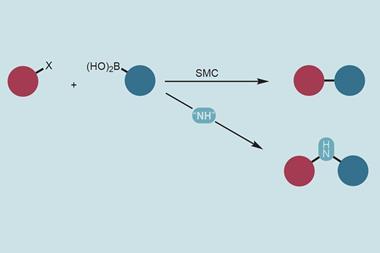
Classic cross-coupling reactions rerouted to make new products
2024-03-07T09:30:00Z
By Ellis Wilde
![research problems chemistry An image showing a thermal ellipsoid plot of [Co(acac)(DPPBz)(THF)]+](https://d2cbg94ubxgsnp.cloudfront.net/Pictures/380x253/1/1/2/503112_thumbhoodsom20013120_936160.png)
Cobalt makes a comeback in hydroformylation catalysis
2020-01-30T19:00:00Z
By Jamie Durrani
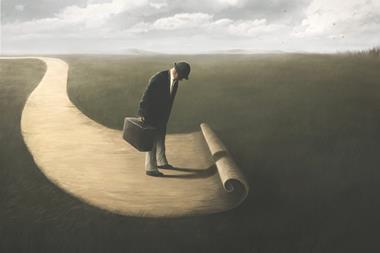
Will science ever reach an end?
2024-05-30T11:00:00Z
By Derek Lowe
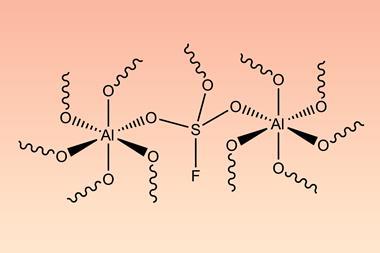
Simple preparation method improves access to long-known superacid
2024-05-24T13:30:00Z
By Jamie Purcell
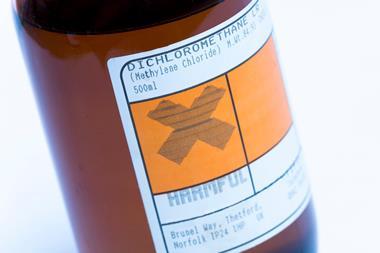
What the dichloromethane ban might mean for university labs in the US
2024-05-23T13:30:00Z
By Rebecca Trager
No comments yet
Only registered users can comment on this article., more from news.
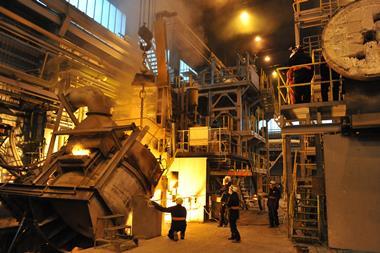
Recycled construction waste could cut cement and steel’s carbon footprint
2024-05-31T11:42:00Z
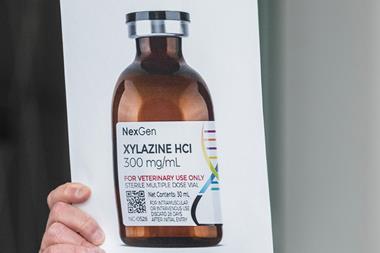
Conjugate vaccine curbs xylazine effects in mice
2024-05-31T08:51:00Z
By Laura Cooper
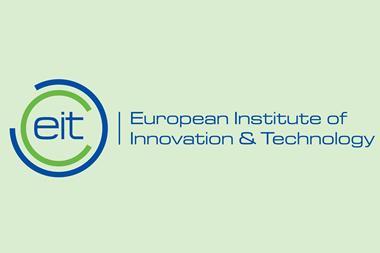
Calls to kill off the European Institute of Innovation and Technology mount
2024-05-30T13:30:00Z
By Angeli Mehta
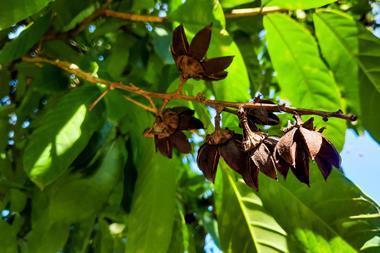
Genetic engineering feat coaxes yeast to produce valuable vaccine compound
2024-05-30T08:30:00Z
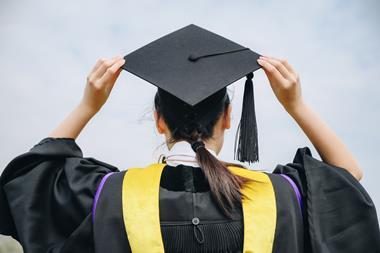
Graduate visa route stays, as UK government proposes ‘crackdown’ on abuses and migration
2024-05-29T13:30:00Z
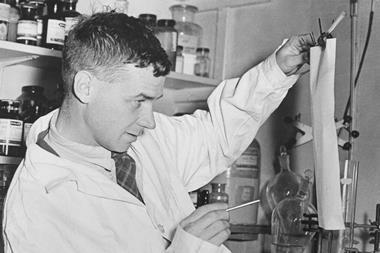
Second Nobel prize medal for partition chromatography to be auctioned
2024-05-29T08:50:00Z
- Contributors
- Terms of use
- Accessibility
- Permissions
- This website collects cookies to deliver a better user experience. See how this site uses cookies .
- This website collects cookies to deliver a better user experience. Do not sell my personal data .
- Este site coleta cookies para oferecer uma melhor experiência ao usuário. Veja como este site usa cookies .
Site powered by Webvision Cloud
- Departments and Units
- Majors and Minors
- LSA Course Guide
- LSA Gateway
Search: {{$root.lsaSearchQuery.q}}, Page {{$root.page}}
- Diversity, Equity & Inclusion
- Climate Reporting
- Technical Services
- News and Events
- Portal/IntrAnet
- Undergraduates
- Alumni and Friends

- Undergraduate Research
- Transfer Credit Policies & Procedures
- Chemistry Graduation Ceremony
- Honors Overview
- Lab Class Resources
- Applying to Graduate School
- Accelerated Degree Program
- Industrial Recruiting
- Useful Links
- PhD Program
- Future Faculty GSI Program
- Teaching Resources
- Fields of Study
- Masters Program
- GSI Job Posting
- MCORE Program
- Graduate Student Resources
- Keep In Touch
- Donor Spotlight
- Annual Alumni Reception
- Newsletters
- Make An Impact
- Search News
Kerri Pratt receives 2024 national Brown Investigator award
- Archived News
- UM Chemistry Featured Elsewhere
- Kopelman Conference | Celebrating the Legacy of Dr. Raoul Kopelman

University of Michigan chemist Kerri Pratt was named to the 2024 class of Brown Investigators, the first class selected through the newly formed Brown Institute for Basic Sciences at Caltech. Pratt, a professor of chemistry and of earth and environmental sciences, will receive $2 million over five years to support her research.
The cohort comprises eight distinguished mid-career faculty working on fundamental challenges in the physical sciences, particularly those with potential long-term practical applications in chemistry and physics.
Pratt studies the chemical interactions between atmospheric trace gases, particles, clouds and snow in the Arctic, which is warming faster than elsewhere on Earth. She focuses as well on wintertime environments, which are highly understudied and often experience poor air quality, she says.
Pratt will focus her Brown Investigator project on the application of new state-of-the-art field-deployable mass spectrometers to discover and measure new chemical compounds in the atmosphere. With these instruments, they will conduct novel “lab-in-the-field” perturbation experiments to explain new chemical mechanisms in the troposphere.
“I was shocked and honored to receive the news from Ross Brown himself,” Pratt said. “I am so excited for my research group to investigate the fundamental details of chemical reactions in the atmosphere. We will use these experiments in the field to discern the details that tell us not just what, but also how and why.”
By comparison, Pratt says, most field measurements and funding are focused on measuring the amount of a chemical compound over time.
The Brown Institute was established in 2023 through a $400-million gift from entrepreneur, philanthropist and Caltech alumnus Ross M. Brown.
Caltech and Brown share a common purpose: advancing fundamental science discoveries with the potential to seed breakthroughs that benefit society.
“My hope is the support provided by the Brown Investigator Awards will help to spark and encourage the researchers’ creativity and enable them to pursue riskier innovative ideas that extend beyond their existing research efforts and align with new or developing passions,” Brown said. “By supporting mid-career faculty, we can provide funding at a time when they are poised and prepared to make profound contributions to their fields.”
--Morgan Sherburne, UM News

- Information For
- Prospective Students
- Current Students
- Faculty and Staff
- More about LSA
- How Do I Apply?
- LSA Magazine
- Student Resources
- Academic Advising
- Global Studies
- LSA Opportunity Hub
- Social Media
- Update Contact Info
- Privacy Statement
- Report Feedback

IMAGES
VIDEO
COMMENTS
Unlike other chemistry research topics, these include elements such as metals, minerals, and inorganic compounds. If you are looking for inorganic chemistry research topics on inorganic chemistry, here are some ideas to get you started: ... There are a lot of environmental problems that need to be solved, and research is the key to solving them ...
Organic Chemistry Research Topics. Organic Chemistry Research Topics are as follows: Development of novel synthetic routes for the production of biologically active natural products. Investigation of reaction mechanisms and kinetics for organic transformations. Design and synthesis of new catalysts for asymmetric organic reactions.
Chemistry articles from across Nature Portfolio. Chemistry is a branch of science that involves the study of the composition, structure and properties of matter. Often known as the central science ...
ACS Publications regularly produces collections of the most important chemistry research topics. These Virtual Collections of the most important chemistry research topics bring together the most important ideas in the field in a variety of ways, including Special Issues and ACS Selects from across the portfolio journals. These collections reflect the most important chemistry research […]
In Chapter 16, Stamovlasis and Vaiopoulou address methodological and epistemological issues concerning research in chemistry problem solving. Following a short review of the relevant literature with emphasis on methodology and the statistical modeling used, the weak points of the traditional approaches are discussed and a novel epistemological ...
Undergraduate Research in Chemistry Guide. Research is the pursuit of new knowledge through the process of discovery. Scientific research involves diligent inquiry and systematic observation of phenomena. Most scientific research projects involve experimentation, often requiring testing the effect of changing conditions on the results.
How A-level chemistry solved the 200-year-old problem with the haloform reaction. 2024-05-23T08:30:00+01:00 By Victoria Atkinson. ... Effects of such microscopic plastic particle ...
The physical sciences community is increasingly taking advantage of the possibilities offered by modern data science to solve problems in experimental chemistry and potentially to change the way ...
Today, Communications Chemistry launches a series of Comment articles discussing key open questions in specific fields of fundamental chemical research. Here we outline the aims of this series and ...
Part of a journal that explores the role of chemistry in our everyday lives, this section publishes significant fundamental and applied work across all branches of organic chemistry. ... Research Topics; Type at least 3 characters 93 Research Topics Guest edit your own article collection Suggest a topic. Submission.
Machine learning models are poised to make a transformative impact on chemical sciences by dramatically accelerating computational algorithms and amplifying insights available from computational chemistry methods. However, achieving this requires a confluence and coaction of expertise in computer science and physical sciences. This Review is written for new and experienced researchers working ...
The chemistry library contains legacy chemistry content, and is not being updated with new content. For our most up-to-date, mastery-enabled courses, check out high school chemistry and AP Chemistry! Unit 1: Atoms, compounds, and ions.
This article was originally published with the title "10 Unsolved Mysteries in Chemistry" in Scientific American Magazine Vol. 305 No. 4 (October 2011) View This Issue. Many of the most ...
This Review of Chemistry Education Research (CER) provides an overview of the development of research in chemistry education from the early days, when ideas about how to teach chemistry and help students learn were guided by practitioner wisdom, to current research that is based on theories of learning and provides evidence from which to make arguments about improving teaching and learning. We ...
An introduction to the Kraemer Family Library's chemistry resources. This guide highlights study guides with practice problems, and has information on looking up chemical properties and on finding chemistry articles for research assignments.
Getting involved in these kind of citizen sciences projects can be a great way to have a positive impact on the world, collecting large volumes of data that enable us to understand our impact on ...
Solving Real Problems with Chemistry (1) is a collection of 20 activities designed to reinforce students' problem solving skills in general chemistry. And yet, it is not just a book of practice problems for students. Each is a process-oriented, guided-inquiry, learning (POGIL) in-context exercise. Also, each POGIL-IC activity is not simply a ...
Many scientific concepts and theorems are often abstract and challenging to relate to real-life problems, making it difficult for students to grasp them. Therefore, some researchers have attempted to enhance students' understanding by employing a contextual learning approach, which allows students to apply scientific knowledge to real situations in their daily lives. The aim is to improve ...
The following situations are considered, some general, others with a focus on specific areas of chemistry: quantitative problems, qualitative reasoning, metacognition and resource activation, deconstructing the problem-solving process, an overview of the working memory hypothesis, reasoning with the electron-pushing formalism, scaffolding ...
Problems in chemistry are considered unsolved when an expert in the field considers it unsolved or when several experts in the field disagree about a solution to a problem. ... Matthew Tirrell - Departments of Chemical Engineering and Materials, Materials Research Laboratory, California NanoSystems Institute, University of California, Santa ...
Abstract. This article discusses the ethical issues unique to the science and practice of chemistry. These issues arise from chemistry's position in the middle between the theoretical and the practical, a science concerned with molecules that are of the right size to directly affect human life. Many of the issues are raised by the central ...
When he first began doing chemical educational research (CER), he, therefore, focused on problem solving in chemistry. The literature on this topic is now so large it would be impossible to review. This chapter, therefore, focuses on the evolution of a model of problem solving developed by the author's research group, which is (i) consistent ...
Two-dimensional (2D) materials, with unique electronic properties, superior optoelectronic properties, and dangling-bond-free surfaces, have attracted significant attention and experienced rapid development both in fundamental science and for practical applications. Amid the plethora of 2D materials, indium Journal of Materials Chemistry A Recent Review Articles
Solving the problems of proton-conducting perovskites for next-generation fuel cells. ScienceDaily . Retrieved May 31, 2024 from www.sciencedaily.com / releases / 2024 / 05 / 240529144022.htm
Zakir Moore '25 was recently recognized for his research into producing industrial quantities of hydrogen in a safer and a more environmentally friendly method. The Central North Carolina Section of the American Chemical Society awarded Zakir first place for his research among undergraduates at the group's annual Poster Vendor Night at the ...
Journal of Materials Chemistry C. First-Principles Study of Optoelectronic Properties and Electric field Modulation in PbS Quantum Dot/Graphene Hybrid Systems ... However, further research and development are needed before this system can be truly applied and commercialized. In this article, the optoelectronic properties of the PbS QD/graphene ...
The world is committed to reducing CO2 emissions, and research on CO2 capture and effective utilization is being actively studied. Among the methods in development, direct air capture (DAC) is classified as a negative emission technology and has attracted significant study. The current problem with CO2 captu
By Victoria Atkinson 23 May 2024. A bench-friendly alternative to traditional hydroformylation replaces the pressurised toxic gas mixture and expensive rhodium catalyst with a commercially ...
University of Michigan chemist Kerri Pratt was named to the 2024 class of Brown Investigators, the first class selected through the newly formed Brown Institute for Basic Sciences at Caltech. Pratt, a professor of chemistry and of earth and environmental sciences, will receive $2 million over five years to support her research.
Rare event sampling is a central problem in modern computational chemistry research. Among the existing methods, transition path sampling (TPS) can generate unbiased representations of reaction processes. However, its efficiency depends on the ability to generate reactive trial paths, which in turn depends on the quality of the shooting algorithm used. We propose a new algorithm based on the ...